Registration Dossier
Registration Dossier
Data platform availability banner - registered substances factsheets
Please be aware that this old REACH registration data factsheet is no longer maintained; it remains frozen as of 19th May 2023.
The new ECHA CHEM database has been released by ECHA, and it now contains all REACH registration data. There are more details on the transition of ECHA's published data to ECHA CHEM here.
Diss Factsheets
Use of this information is subject to copyright laws and may require the permission of the owner of the information, as described in the ECHA Legal Notice.
EC number: 910-757-7 | CAS number: -
- Life Cycle description
- Uses advised against
- Endpoint summary
- Appearance / physical state / colour
- Melting point / freezing point
- Boiling point
- Density
- Particle size distribution (Granulometry)
- Vapour pressure
- Partition coefficient
- Water solubility
- Solubility in organic solvents / fat solubility
- Surface tension
- Flash point
- Auto flammability
- Flammability
- Explosiveness
- Oxidising properties
- Oxidation reduction potential
- Stability in organic solvents and identity of relevant degradation products
- Storage stability and reactivity towards container material
- Stability: thermal, sunlight, metals
- pH
- Dissociation constant
- Viscosity
- Additional physico-chemical information
- Additional physico-chemical properties of nanomaterials
- Nanomaterial agglomeration / aggregation
- Nanomaterial crystalline phase
- Nanomaterial crystallite and grain size
- Nanomaterial aspect ratio / shape
- Nanomaterial specific surface area
- Nanomaterial Zeta potential
- Nanomaterial surface chemistry
- Nanomaterial dustiness
- Nanomaterial porosity
- Nanomaterial pour density
- Nanomaterial photocatalytic activity
- Nanomaterial radical formation potential
- Nanomaterial catalytic activity
- Endpoint summary
- Stability
- Biodegradation
- Bioaccumulation
- Transport and distribution
- Environmental data
- Additional information on environmental fate and behaviour
- Ecotoxicological Summary
- Aquatic toxicity
- Endpoint summary
- Short-term toxicity to fish
- Long-term toxicity to fish
- Short-term toxicity to aquatic invertebrates
- Long-term toxicity to aquatic invertebrates
- Toxicity to aquatic algae and cyanobacteria
- Toxicity to aquatic plants other than algae
- Toxicity to microorganisms
- Endocrine disrupter testing in aquatic vertebrates – in vivo
- Toxicity to other aquatic organisms
- Sediment toxicity
- Terrestrial toxicity
- Biological effects monitoring
- Biotransformation and kinetics
- Additional ecotoxological information
- Toxicological Summary
- Toxicokinetics, metabolism and distribution
- Acute Toxicity
- Irritation / corrosion
- Sensitisation
- Repeated dose toxicity
- Genetic toxicity
- Carcinogenicity
- Toxicity to reproduction
- Specific investigations
- Exposure related observations in humans
- Toxic effects on livestock and pets
- Additional toxicological data

Endpoint summary
Administrative data
Link to relevant study record(s)
- Endpoint:
- basic toxicokinetics in vitro / ex vivo
- Type of information:
- read-across from supporting substance (structural analogue or surrogate)
- Adequacy of study:
- supporting study
- Study period:
- 2005
- Reliability:
- 1 (reliable without restriction)
- Rationale for reliability incl. deficiencies:
- test procedure in accordance with generally accepted scientific standards and described in sufficient detail
- Remarks:
- This study was conducted according to GLP and sufficient data is available for interpretation of results.
- Objective of study:
- metabolism
- Principles of method if other than guideline:
- In vitro metabolism in rat, mouse and human liver and lung microsomes was reported.
- GLP compliance:
- yes
- Radiolabelling:
- no
- Species:
- other: microsomes from liver and lung tissues from rat, mouse and human
- Strain:
- other: male rats (un-induced Fischer 344 rats), male mice (un-induced B6C3F1 mice) and humans (mixed gender)
- Sex:
- male/female
- Details on test animals or test system and environmental conditions:
- Mouse liver and lung microsomes were prepared from the pool of 126 and 100 animals, respectively. Human liver microsomes were from the pool of 50 individuals of mixed gender, race (Caucasian, African, and Hispanic) and age (6 to 78 years old with most of them between 30 and 50 years), 31 of them died from cerebrovascular stroke, 11 from head trauma, 4 from anoxia, 2 from cardiac arrest, and 2 from aortic aneurysm. Human lung microsmes of non-smokers were prepared from the pool of 4 individuals of mix gender, 1 die d from stroke, 1 from interacranial hemorrhage, 1 from aneurysm, and 1 from motor vehicle accident. Microsomal samples were received in small aliquots (0.5 mL containing 5 or 10 mg protein) in eppendorf tubes on dry ice and immediately stored at –80°C. Each microsomal preparation was analyzed by the supplier for at least CYP1A1 activity by the rate of metabolism of 7-ethoxyresorufin O-dealkylation (EROD assay). The samples were reanalyzed for CYP1A1 activity by EROD assay using a microplate fluorometric method after the completion of the experiments on the microsomes subjected to one thaw and freeze cycle (used in the metabolism study), and found to be active. The 1A1 activity of human lung microsomes was about 7% of the levels measured in rat lung microsomes, and was 4-fold lower than what has been reported for nonsmoker human lung microsomes prepared from fresh tissues obtained within 15 minutes of lobectomy. The 1A1 activity of mouse lung microsomes was 16-fold higher than rat lung. The 1A1 activity in the liver microsomes was in the order of mouse > rat >> human.
- Route of administration:
- other: in vitro
- Vehicle:
- unchanged (no vehicle)
- Details on exposure:
- Two concentrations (750 and 7500 ppm) of EB vapor in air were incubated in triplicates with liver or lung microsomes (2 mg microsomal protein in 2 mL reaction mixture containing 0.1 M phosphate buffer and 1 mM NADPH, pH 7.4) in 25 mL gastight glass vials. Two EB Tedlar bags were prepared by drawing a known volume of ambient air through a heated J-tube containing EB. The buffer containing NADPH and liver or lung microsomes were placed in vials and sealed with open-hole screwcaps fitted with Teflon®/silicone septa. Approximately 150 mL of EB vapor were drawn from each Tedlar bag into an airtight Hamilton syringe and the headspaces of the vials containing reaction mixtures were purged using 23 gauge needles (injection and vent needles). This volume (~150 mL) of EB was enough to effectively flush the headspace of each vial with 5-6 volume changes. Each vial was placed in a shaking water bath maintained at 37°C immediately after adding EB vapor into the headspace.
- Duration and frequency of treatment / exposure:
- The microsomal reaction mixtures were incubated for 30 minutes. After 30 minutes, vials were removed from the water bath and immediately placed on dry ice to condense EB vapors and also to stop metabolic reaction.
- Dose / conc.:
- 750 other: ppm EB vapour in air (nominal)
- Remarks:
- For liver microsomal incubation, the measured concentration of EB vapor in Tedlar bags was 607 ppm (amount added to each vial was 0.57 μmoles). For lung microsomal incubation, the measured concentration of EB vapor in the prepared Tedlar bags was 680 ppm (amount added to each vial was 0.64 μmoles).
- Dose / conc.:
- 7 500 other: ppm EB vapour in air (nominal)
- Remarks:
- For liver microsomal incubation, the measured concentration of EB vapor in Tedlar bags was 6545 ppm (amount added to each vial was 6.16 μmoles). For lung microsomal incubation, the measured concentration of EB vapor in the prepared Tedlar bags was 6712 ppm (amount added to each vial was 6.31 μmoles).
- No. of animals per sex per dose / concentration:
- Two concentrations (750 and 7500 ppm) of EB vapor in air were incubated in triplicates with liver or lung microsomes (2 mg microsomal protein in 2 ml reaction mixture containing 0.1 M phosphate buffer and 1 mM NADPH, pH 7.4) in 25 mL gastight glass vials.
- Control animals:
- other: Two types of control were also prepared and incubated with the microsomal reaction mixtures - one a negative control without EB and the other to determine non-enzymatic conversion of EB to the metabolite/s of interest.
- Positive control reference chemical:
- not applicable
- Details on study design:
- Two concentrations (750 and 7500 ppm) of EB vapor in air were incubated in triplicates with liver or lung microsomes (2 mg microsomal protein in 2 mL reaction mixture containing 0.1 M phosphate buffer and 1 mM NADPH, pH 7.4) in 25 mL gastight glass vials. Two EB Tedlar bags were prepared by drawing a known volume of ambient air through a heated J-tube containing EB. The buffer containing NADPH and liver or lung microsomes were placed in vials and sealed with open-hole screwcaps fitted with Teflon®/silicone septa. Approximately 150 mL of EB vapor were drawn from each Tedlar bag into an airtight Hamilton syringe and the headspaces of the vials containing reaction mixtures were purged using 23 gauge needles (injection and vent needles). This volume (~150 mL) of EB was enough to effectively flush the headspace of each vial with 5-6 volume changes. Each vial was placed in a shaking water bath maintained at 37°C immediately after adding EB vapor into the headspace. The microsomal reaction mixtures were incubated for 30 minutes. After 30 minutes, vials were removed from the water bath and immediately placed on dry ice to condense EB vapors and also to stop metabolic reaction.
- Details on dosing and sampling:
- Once the reaction mixture was frozen, 2 mL of carbon disulfide was injected through the septum-lined caps, the vials were rolled to extract EB from the sides and 200 μL of formic acid: ascorbic acid mixture (10% formic acid: 20% ascorbic acid, v/v in Milli-Q water) was injected. The vials were kept in a –80°C freezer overnight, allowed to thaw at room temperature and shaken for 30 minutes at low speed on a flatbed shaker. The carbon disulfide layer was removed and dried over ~200 mg MgSO4 and analyzed for EB and five metabolites (1-phenylethanol, 2-phenylethanol, 2-ethylphenol, 4-ethylphenol, and acetophenone) using GC/FID.
- Statistics:
- Descriptive statistics (i.e., mean ± standard deviation) for the depletion of parent EB and formation of metabolites were calc ulated using Microsoft Excel spreadsheets in full precision mode (15 digits of accuracy).
- Metabolites identified:
- yes
- Details on metabolites:
- Recovery of Metabolites - Recovery of each of the metabolites from the rat liver and lung microsomes was very consistent ranging from 71-92% from liver microsomes and 73-98% from lung microsomes with the exception of 2-phenylethanol, which was 63 and 67% from liver and lung microsomes, respectively. It was assumed that the recovery of metabolites from the mouse and human tissues was similar to rat tissues.
Metabolism of EB by rat, mouse and human liver microsomes - The major metabolite of EB formed after 30 minutes of incubation was 1-phenylethanol. At the low concentration, mouse liver converted 19% of EB to 1-phenylethanol; conversion at the high concentration was 3%. Human liver microsomes were also active in metabolizing EB to 1-phenylethanol, converting 9 and 1.6% at the low and high concentrations, respectively. Metabolism of EB to 1-phenylethanol by rat liver microsomes was 7 and 2% at the low and high concentrations, respectively. Acetophenone was detected only in rat liver microsomes at both of the concentrations (0.7 and 0.1% conversion at low and high concentrations, respectively) and only at the low concentration (1.7% conversion) in mouse liver microsomes. The levels of acetophenone in human liver microsomal incubations remained below the detection limit of 0.0005 μmoles. Formation of 2-phenylethanol was only detectable at the high concentration in all three liver microsomes ranging between 0.02-0.06% of the added EB. The 2-ethylphenol metabolite was formed only by the mouse liver microsomes at both concentrations accounting for 0.18 and 0.03% conversion at the low and high concentrations, respectively and remained below the limit of detection (0.0005 μmoles) in other species. No 4-ethylphenol was detected above the limit of instrumental detection (0.0007 μmole) in the liver microsomes of any of the species at the end of the incubation period of 30 minutes.
Metabolism of EB by rat, mouse and human lung microsomes - In contrast to liver, human lung microsomes were unable to metabolize EB; levels of all of the targeted metabolites remained below the detection limit of 0.0005-0.0007 μmoles in the incubation mixtures. Similar to liver, the major metabolite of EB detected after 30 minutes of incubation was 1-phenylethanol in rat and mouse lung microsomes. At the low and high concentrations, mouse lung converted 19 and 5% of EB to 1-phenylethanol, respectively. Metabolism of EB to 1-phenylethanol by rat lung microsomes was 11 and 2% at the low and high concentrations, respectively. Much higher levels of acetophenone was detected in mouse lung microsomal incubations, accounting for 6 and 1% (4-fold higher than liver) conversion at the low and high concentrations, respectively. Conversion of EB to acetophenone by rat lung microsomes was comparable to the liver microsomes at both of the concentrations accounting for 0.8 and 0.1% conversion at low and high concentrations, respectively. Again, in contrast to the liver microsomes, rat lung microsomes were much more active (8- to 10-fold) in converting EB to 2-phenylethanol accounting for 0.3 to 2.5%. Detectable levels of 2-ethylphenol were formed both by rats and mouse lung microsomes in contrast to the liver microsomes where only mouse liver microsomes converted EB to 2-ethylphenol, although levels remained between 0.02 and 0.3% conversion. Detectable levels of 4-ethylphenol were formed only in the mouse lung microsomes at the high concentration, others remained below the detection limit of 0.0007 μmole at the end of the incubation period of 30 minutes. - Conclusions:
- Mouse liver microsomes were >2-fold active in converting Ethylbenzene (EB) to its metabolites than rats whereas, EB metabolism rates in human liver microsomes were similar to rats. In case of microsomal metabolism, 2-ethylphenol was found to be one of the minor metabolites, whereas it remained below the limit of detection in humans. The results distinctly demonstrate that mouse liver microsomes are much more active in metabolising EB than either rat or human liver microsomes.
- Executive summary:
The study was designed to generate comparative metabolism data of EB using rat, mouse and human liver and lung microsomes. Two concentrations (750 and 7500 ppm) of EB vapor were incubated with 2 mg of liver or lung microsomal protein in gas-tight glass vials at 37°C for 30 minutes. After completion of the incubation, EB and metabolites were extracted and analyzed by GC/FID.
EB was preferentially metabolized to 1-phenylethanol by liver microsomes, conversion was in the order of mouse >> rat » human. Acetophenone was detected in rat liver microsomes at both of the concentrations and only at the low concentration in mouse liver microsomes, the levels of acetophenone in human liver microsomal incubations remained below the detection limit. Formation of 2-phenylethanol was only detectable at the high concentration in all three liver microsomes. The 2 -ethylphenol was formed only by the mouse liver microsomes at both concentrations accounting for 0.18 and 0.03% conversion at the low and high concentrations, respectively and remained below the limit of detection in other species. No 4-ethylphenol was detected above the limit of detection in the liver microsomes of any of the species.
Both rat and mouse lung microsomes were more active in metabolizing EB than liver microsomes. In contrast to liver, human lung microsomes were unable to metabolize EB and levels of all of the targeted metabolites remained below the detection limit. Similar to liver, the major metabolite of EB was 1-phenylethanol both by rat and mouse lung microsomes. Detectable levels of 2-phenylethanol were formed at the low as well as high concentration by the rat and mouse lung microsomes. Detectable levels of 2-ethylphenol were formed both by rats and mouse lung microsomes in contrast to the liver microsomes where only mouse liver microsomes converted EB to 2-ethylphenol. Detectable levels of 4-ethylphenol were formed only in the mouse lung microsomes at the high concentration, whereas liver microsomes were unable to generate 4-ethylphenol above the detection limit. Mouse lung microsomes were again more active in metabolizing EB than rat lung microsomes
- Endpoint:
- basic toxicokinetics in vitro / ex vivo
- Type of information:
- read-across from supporting substance (structural analogue or surrogate)
- Adequacy of study:
- supporting study
- Study period:
- 2007
- Reliability:
- 1 (reliable without restriction)
- Rationale for reliability incl. deficiencies:
- test procedure in accordance with generally accepted scientific standards and described in sufficient detail
- Remarks:
- This study was conducted according to GLP and sufficient data is available for interpretation of results.
- Objective of study:
- metabolism
- Principles of method if other than guideline:
- Ethylbenzene was incubated at concentrations ranging from 0.22 to 7 mM, with liver and lung microsomes of mouse, rat and human to measure the formation of 1-phenylethanol (1-PE), acetophenone, 2,5-ethylquinone, and 3,4-ethylquinone. The latter two reactive metabolites were monitored via a glutathione (GSH) trapping technique.
- GLP compliance:
- yes
- Radiolabelling:
- not specified
- Species:
- other: microsomes from liver and lung tissues from rats, mice and humans
- Strain:
- other: male rats (un-induced Fischer 344 rats), male mice (un-induced B6C3F1) and humans (mixed gender and race)
- Sex:
- male/female
- Details on test animals or test system and environmental conditions:
- Mouse liver and lung microsomes were prepared from the pool of 126 and 100 animals, respectively. Human liver microsomes were from the pool of 50 individuals of mixed gender, race (Caucasian, African, and Hispanic) and age (6 to 78 years old with most of them between 30 and 50 years), 31 ofthem died from cerebrovascular stroke, 12 from head trauma, 5 from anoxia, 1 from myocardial infarction, and 1 from aortic aneurysm. Human lungmicrosomes of non-smokers were prepared from the pool of 4 individuals of mixed gender (3 male, 1 female), 1 died from stroke, 1 from interacranial hemorrhage, 1 from drug overdose, and 1 from motor vehicle accident. Microsomal samples were received in small aliquots (0.5 mL containing 5 or 10 mg protein) in eppendorf tubes on dry ice and immediately stored at –80°C. Each microsomal preparation was analyzed by the supplier for at least CYP1A1/1A2 activity by the rate of metabolism of 7-ethoxyresorufin O-dealkylation (EROD assay). The samples were reanalyzed for CYP1A1/1A2 activity by EROD assay using a microplate fluorometric method. The 1A1/1A2 activity of human lung microsomes was about 7% of the levels measured in rat lung microsomes, and was 4-fold lower than what has been reported for nonsmoker human lung microsomes prepared from fresh tissues obtained within 15 minutes of lobectomy. The 1A1/1A2 activity of mouse lung microsomes was 16-fold higher than rat lung. The 1A1/1A2 activity in the liver microsomes was in the order of mouse > rat >> human
- Route of administration:
- other: in vitro
- Vehicle:
- not specified
- Details on exposure:
- Microsomal Metabolism of 2- and 4-Ethylphenol - One concentration of 2- or 4-ethylphenol (1 mM final concentration) was incubated for 30 minutes at 37°C with mouse, rat and human lung and liver microsomes (1 mg protein/mL 0.1 M phosphate buffer, cofactors, pH 7.4) in 24-mL gas-tight glass vials. Each treatment (substrate/tissue type) was comprised of three replicates. Two types of control incubations were also conducted. One control type contained all components (0.1M phosphate buffer, NADPH, microsomes), but no substrate; the second control was conducted with substrate and microsomes, but no NADPH. After the completion of the incubations, samples were analyzed for the loss of 2- and 4-ethylphenol, as well as formation of ethylhydroquinone and ethylcatechol by high performance liquid chromatography with ultraviolet detection (HPLC/UV).
As a basis of comparison with the preliminary EB metabolism study, mouse liver and/or lung microsomes were incubated with 750 μg of EB delivered to 1 mL of the incubation medium using propylene glycol (PG) as vehicle (or, 7 mM EB in test system). After completion of 30 minute incubations with EB, samples from the current study were analyzed for formation of 1-phenylethanol, 2EP, 4EP (as done in the preliminary study), as well as ethylhydroquinone (EHQ) and ethylcatechol (ECat).
Rate of Microsomal Metabolism of Ethylbenzene to 1-Phenylethanol, Acetophenone, Ethylhydroquinone and Ethylcatechol - Probe incubations of EB with mouse liver microsomes were conducted (at a single substrate concentration) to assess the linearity of the rates of formation of 1-phenylethanol, acetophenone, 2,5-ethylquinone, and 3,4-ethylquinone over time. Mouse liver microsomes were incubated with 2 mM EB (and 1 mg protein/mL 0.1M phosphate buffer, pH 7.4, 1 mM NADPH, 10 mM glutathione) in glass vials. Incubations were conducted for 10, 15, 30, 60, and 90 minutes (two replicate vessels per incubation time).
The kinetics of 1-PE and AcPh (major metabolites of EB) as well as kinetics of 2,5- and 3,4-ethylquinone (potential reactive metabolites of EB) formation were conducted. Liver and lung microsomes of three species (rat, mouse, human) were incubated in triplicate at 37°C with EB at six concentrations. The substrate concentrations were 7.0, 3.5, 1.8, 0.9, 0.45, and 0.22 mM EB. The incubation time was 30 min, based on the results of the time-course experiments. Six separate PG-based solutions of EB were prepared such that the EB, for all treatment levels, was delivered in a volume of 10 μL.
Three types of control incubations were conducted. Control Type I contained all components (0.1 M phosphate buffer, NADPH, microsomes, glutathione, 10 μL PG), but no EB; Control Type II was conducted with incubates containing 0.45, 1.8, or 7.0 mM EB (single replicate for each [of six] microsome types), glutathione (GSH) and microsomes, but no NADPH; Control Type III contained all components (with 0.45 mM EB), but no microsomes. - Control animals:
- no
- Positive control reference chemical:
- not applicable
- Details on study design:
- Further to description under details of exposure - Following the 30 min incubations, 0.2 mL of a so-called “kill solution” was injected through the septum of each incubation vial (i.e., vials remained sealed from the point of mixing components through incubation period, and until after kill solution was added). The kill solution was comprised of 2% formic acid (to reduce pH), 5% ascorbic acid (anti-oxidant), and a mixture of internal standards in a solution of 20/80 H2O/acetonitrile. In addition to controlling pH and reducing potential for oxidation, the kill solution served the following purposes: a) stop the metabolic reactions; b) enhance the solubility of EB prior to analysis due to the addition of acetonitrile (as substrate concentrations in treatments of 1.8 mM and above may have exceeded the water solubility of EB), c) and internal standards of volatile analytes would control for the loss those analytes during sample preparation in addition to controlling for the variability in MS response during analysis. The internal standards contained in the kill solution were d10-EB, d5-1-PE, and d5-AcPh (with “dx” signifying the number of deuteriums substituted in the molecular structure), at a concentration of 100 μg/mL, each. After the addition of the kill solution, the vials were briefly chilled by placing them in an ice bath. Then the vials were opened, and to remove the precipitated protein and other solids, the samples were filtered through Whatman (Florham Park, New Jersey) 13-mm ZC PTFE syringe filters (0.2 μ pore) and collected in autosampler vials. The syringes used were 1-mL plastic body. The collected filtrate was subdivided for the two separate assays, with each preparation completed as follows. For GC/MS analysis of EB, 1-PE, and AcPh, an 0.3-mL aliquot was transferred to a 1-dram vial and extracted with 0.6 mL of CS2 (by shaking for 30 min on a flatbed shaker). The bottom layer (CS2) was transferred to a 1-dram vial containing ~100 mg MgSO4 to remove absorbed water from the CS2. The “dried” CS2 was then decanted to an autosampler vial for analysis by GC/MS. In the preparation for analysis of reactive metabolites (e.g., 2EP-GSH and 4EP-GSH), a 0.3-mL aliquot of the initial filtrate was transferred to an autosampler vial to which was added a laboratory-synthesized internal standard (d4-4EP-GSH). Samples were stored at –80°C while awaiting analysis.
- Details on dosing and sampling:
- Following the incubation and after addition of a so called kill solution, each solution was processed for two types of analysis. The first analysis, by gas chromatography with mass spectrometry (GC/MS), was conducted for the determination of EB and the major (volatile) metabolites, 1-PE and AcPh. The second analysis utilized a glutathione trapping technique (which is the reason that 10 mM GSH was added to the incubation solutions) as previously employed for the in vitro metabolism of 4-vinylphenol to hydroquinone and catechol. The GSH conjugates were analyzed by high performance liquid chromatography with multiple reaction monitoring mass spectrometry (HPLC/MRM/MS).
- Statistics:
- Descriptive statistics (i.e., mean ± standard deviation) for the depletion of parent EB and formation of metabolites were calculated using Microsoft Excel® spreadsheets in full precision mode (15 digits of accuracy). The rates of metabolism were calculated using standard methods (e.g., Eadie-Hofstee, Lineweaver-Burk plots).
- Metabolites identified:
- yes
- Details on metabolites:
- Microsomal Metabolism of 2- and 4-Ethylphenol to Dihydroxyethylbenzenes - In general a higher percent conversion was observed in the metabolism of 2EP to EHQ compared to the conversion of 4EP to ECat. The highest conversion occurred in incubations with mouse lung microsomes for both metabolic pathways; approximately 18% of the 2EP was metabolized to EHQ and ~7% of the 4EP was metabolized to ECat. Likewise, in incubations with liver microsomes, the highest percent conversion occurred in incubations with mouse liver microsomes. Compared across tissue types, the percent conversion from mouse lung incubations was roughly 2-fold greater than the conversion obtained from incubations with mouse liver microsomes. The percent conversion (of both metabolic pathways) was approximately equivalent from incubations with rat lung and liver tissues. Human liver microsomes converted more of the ethylphenols to the corresponding dihydroxyethylbenzene than the lung microsomes. Between the two pathways, human liver microsomes formed about 3-fold higher EHQ than ECat.
Molar conversion of 2EP to EHQ ranged from 6 to 9% in liver microsomes of the three species (mouse [8.9] > human [7.1] >rat [6.4]) and from 0.1 to 18% in lung microsomes (mouse [17.7] > rat [5.8] > human [0.1]). Conversion of 4EP to ECat ranged from 2 to 4% in liver microsomes (mouse [3.6] > human [2.1] ~ rat [2.0]) and from 0.3 to 7% in lung microsomes (mouse [7.1] > rat [1.4] > human [0.3]).
Microsomal Metabolism of Ethylbenzene to Dihydroxyethylbenzenes - Neither EHQ nor ECat was formed in any of the incubations (with mouse, rat, human; liver or lung microsomes) at levels exceeding the limit of detection (0.001 μmole per incubation vessel; corresponding to ~ 0.01% conversion.
Another secondary objective was to measure levels of 1-PE, 2EP, and 4EP and to compare those levels (from incubations in which EB was introduced using PG vehicle) with the levels of those metabolites measured in the preliminary study, in which EB was introduced as a vapor into the incubation vessels. The average percent conversions of EB to 1-PE in the experiments of this study (at a 7mM EB concentration) were 2.4%, 2.0% and 0.9% in liver
microsomal incubates of mouse, rat, and human, respectively. The average percent conversions of EB to 1-PE in the incubations with lung microsomes (of mouse and rat) were 6.1% and 1.7%, respectively. 1-PE was not detected in incubations of EB with human lung microsomes in either the preliminary or the current study (limits of detection ~0.0005 μmoles per vessel in preliminary study; ~0.001 μmoles per vessel in current study). 4EP was not detected in any of the incubations with EB introduced in PG vehicle (current study). In contrast, 2EP was formed at detectable levels, but only in incubations with mouse lung microsomes (of current study). The percent conversion of EB to 2EP in the current study was approximately 0.1%.
Comparative Levels of Metabolite Formation - Incubations of EB with human lung microsomes failed to produce detectable levels of any of the four metabolites. 1-PE represents the major metabolite formed during microsomal incubations with EB, regardless of species or tissue type. Except for incubations with human lung microsomes, 1-PE was formed at detectable levels in all in vitro incubations. There was more 1-PE formed in mouse lung tissue incubations than in incubations with mouse liver microsomes, but 1-PE formed in incubations with rat liver and lung microsomes were comparable. 1-PE levels were 2 to 5-fold higher in incubates of mouse lung compared to levels in rat lung incubation solutions. Levels of 1-PE formed in the liver microsome incubates followed the general rank order of mouse > rat > human.
Acetophenone was formed at much lower levels than 1-PE (it was not detectable in the majority of samples from the rat lung and human liver incubations), but otherwise followed trends similar to those observed for 1-PE.
Glutathione adducts of the reactive metabolites (i.e., quinones of ethylhydroquinone and ethylcatechol) were formed at much lower levels than that of 1-PE and AcPh. Molar conversions ranged from 0.0001% (4EP-GSH; rat lung) to 0.6% (2EP-GSH; mouse lung) compared to conversions of EB to 1-PE that ranged from 1% (rat lung; 7mM EB) to 58% (mouse lung; 0.22 mM EB). The GSH adducts of 2,5-ethylquinone were generally formed in higher amounts than GSH adducts of 3,4-ethylquinone; typically the differences were at least 10-fold. - Conclusions:
- It was concluded that although ring oxidised metabolites accounted for a relatively small fraction of overall ethylbenzene metabolism, its selective elevation in mouse lung microsomes was consistent with the mode of action attributing preferential formation of lung derived cytotoxic, ring-oxidized metabolites as driving the mouse lung specific toxicity of ethylbenzene.
- Executive summary:
Ethylbenzene was incubated at concentrations ranging from 0.22 to 7 mM, with liver and lung microsomes of mouse, rat and human to measure the formation of 1-phenylethanol (1-PE), acetophenone, 2,5-ethylquinone, and 3,4-ethylquinone. The latter two reactive metabolites were monitored via a glutathione (GSH) trapping technique.
Molar conversion to the four metabolites varied quite broadly depending on microsome species/tissue and substrate concentration. None of the metabolites were formed at detectable levels in incubations with human lung microsomes. Alkyl-hydroxylated metabolites (1-phenylethanol, acetophenone) were formed at much higher levels than the ring-hydroxylated metabolites (catechols, hydroquinones, quinones). Molar conversion to the major metabolite, 1-PE, ranged from 1% (rat lung at 7mM EB) to 58% (mouse lung at 0.22 mM EB). This was equivalent to the formation of 0.09 μmole 1-PE by rat lung and 0.13 μmole by mouse lung; a difference of ~2-fold. The mass of 1-PE increased with increasing substrate levels, although the percent conversion (relative to starting substrate concentration) decreased. There was more 1-PE formed in mouse lung tissue incubations than in incubations with mouse liver microsomes.
Levels of 1-PE formed in incubations with rat liver and lung microsomes were similar. The metabolism of EB to 1-PE, ranked according to species, was mouse > rat > human. 1-PE was formed at a level that was roughly an order of magnitude greater than acetophenone.
In a previous study in which EB was incubated with liver and lung microsomes, very little aromatic-oxidation to either 2-ethylphenol (2EP) or 4 -ethylphenol (4EP) was detected. It was surmised that the low levels of the mono-hydroxylated aromatic metabolites may have been due to further rapid oxidation to the di-hydroxylated catechol and quinone metabolites. It should be noted that in the earlier study, the GSH trapping technique used in this current study to afford greater sensitivity to quinones formed via catechols and hydroquinones was not employed. To investigate the potential for further oxidation, high concentrations of 2EP and 4EP were incubated with microsomes, and the formation of ethylcatechol (ECat) and ethylhydroquinone (EHQ) monitored. Conversion from the mono- to the dihydroxylated aromatics did occur, with molar conversion of 2EP to EHQ ranging from 6 to 9% in liver microsomes of the three species (mouse[8.9] > human[7.1] > rat[6.4]) and from 0.1 to 18% in lung microsomes (mouse[17.7] > rat[5.8] > human[0.1]). Conversion of 4EP to ECat ranged from 2 to 4% in liver microsomes (mouse[3.6] > human[2.1] ~ rat[2.0]) and from 0.3 to 7% in lung microsomes (mouse[7.1]>rat[1.4]>human[0.3]). In order to trap the reactive metabolites formed from 2EP and 4EP (i.e.,
the quinones derived from catechols and hydroquinones), experiments were conducted after adding excess GSH to each incubate.
Percent conversion of EB to ring-hydroxylated metabolites was much lower than what was observed for the alky-hydroxylated metabolites, ranging from 0.0001% (4EP-GSH; rat lung) to 0.6% (2EP-GSH; mouse lung). 2EP-GSH concentrations were typically 10-fold higher than 4EP-GSH. At lower substrate concentrations, more 2EP-GSH formed during incubations with lung microsomes than liver microsomes, for both rats and mice. More 2EP-GSH was formed in incubations with mouse liver microsomes than in incubations with liver microsomes from rat and human.
The highest levels of ring-hydroxylated metabolites were formed in incubations with mouse lung microsomes. Interestingly, both mouse and rat lung microsomes (and to a lesser extent, mouse liver microsomes) exhibited decreasing amounts of ring-oxidized metabolite formation with increasing concentrations of ethylbenzene. This suggests the possibility of cytochrome P450 suicide inhibition by reactive ring-oxidized metabolite(s). The possible suicide inhibition appears to be isozyme-specific in that generation of alkyl-oxidized metabolites was not similarly decreased with increasing EB substrate concentrations. This observation is consistent with the hypothesis that reactive ring-oxidized metabolites are likely formed by cytochrome P450 2F2, while alkyl-oxidized metabolite formation is mediated predominantly through cytochrome P450 2E1.
Although ring-oxidized metabolites accounted for a relatively small fraction of overall ethylbenzene metabolism, its selective elevation in mouse lung microsomes is nonetheless consistent with the hypothesized mode of action attributing preferential formation of lung-derived cytotoxic, ring-oxidized metabolites as driving the mouse lung specific toxicity of ethylbenzene.
- Endpoint:
- basic toxicokinetics in vitro / ex vivo
- Type of information:
- read-across from supporting substance (structural analogue or surrogate)
- Adequacy of study:
- supporting study
- Study period:
- 10/2006-11/2007
- Reliability:
- 1 (reliable without restriction)
- Rationale for reliability incl. deficiencies:
- test procedure in accordance with generally accepted scientific standards and described in sufficient detail
- Remarks:
- GLP-study with well described method
- Objective of study:
- other: The objectives were to verify the structure of metabolite B, the 3,4-catechol of styrene and to directly characterize the CYPs responsible for epoxidation of 4-VP to form Metabolite E, the 4 VP-epoxide (4-VPO).
- Qualifier:
- no guideline available
- GLP compliance:
- yes
- Details on metabolites:
- The purpose of this study was to investigate selected aspects of the metabolism of 4-vinylphenol, a hepatotoxic and pneumotoxic metabolite of styrene. In a previous study, two oxidative metabolites of 4VP were identified during incubations of styrene with mouse lung microsomes (Metabolites B and E). The first objective of the current study was to verify the structure of metabolite B, the 3,4-catechol of styrene. The structure was verified. The second objective was to directly characterize the CYPs responsible for epoxidation of 4-VP to form Metabolite E, the 4 VP-epoxide (4-VPO). Experiments with 5-phenyl-1-pentyne (5P1P), an inhibitor of CYP 2F2, and diethyldithiocarbamate (DDTC), an inhibitor of CYP 2E1, were conducted and 4-VPO formation was evaluated in mouse, rat, and human donor lung microsomes. Both inhibitors decreased the yield of the 4-VPO trapped GSH conjugates across species and tissues tested. The inhibitor 5P1P had a greater inhibitory effect in the mouse lung than DDTC (85% vs. 57%), suggesting that 2F2 was primarily responsible for 4-VPO formation in the mouse lung. Not all of the apparent DDTC inhibitory effects seen in this study are due to direct inhibition of CYP 2E1, since DDTC was found to directly react with 4-VPO. Thus, further evidence was developed showing CYP 2F2 was responsible for the formation of 4-VPO in mouse lung. The third objective of this study was to evaluate the regioselectivity of glutathione (GSH) addition to 4-VPO. Using a synthetic standard of 4-VPO, two regio-isomers of 4-VPO-GSH conjugates were isolated and identified as R and S enantiomers of the C1-regioisomer of 4-VPO-GSH. The two enantiomers of the C2-4-VPO-GSH metabolites were isolated, but not fully characterized. There was no species or tissue difference in the regio- or stereoselectivity of 4VPO-GSH formation. This study provides better insight on the chemicals responsible for the lung toxicity seen with 4-VP and possibly styrene.
- Executive summary:
The purpose of this study was to investigate selected aspects of the metabolism of 4-vinylphenol, a hepatotoxic and pneumotoxic metabolite of styrene. In a previous study, two oxidative metabolites of 4VP were identified during incubations of styrene with mouse lung microsomes (Metabolites B and E). The first objective of the current study was to verify the structure of metabolite B, the 3,4-catechol of styrene. The structure was verified. The second objective was to directly characterize the CYPs responsible for epoxidation of 4-VP to form Metabolite E, the 4 VP-epoxide (4-VPO). Experiments with 5-phenyl-1-pentyne (5P1P), an inhibitor of CYP 2F2, and diethyldithiocarbamate (DDTC), an inhibitor of CYP 2E1, were conducted and 4-VPO formation was evaluated in mouse, rat, and human donor lung microsomes. Both inhibitors decreased the yield of the 4-VPO trapped GSH conjugates across species and tissues tested. The inhibitor 5P1P had a greater inhibitory effect in the mouse lung than DDTC (85% vs. 57%), suggesting that 2F2 was primarily responsible for 4-VPO formation in the mouse lung. Not all of the apparent DDTC inhibitory effects seen in this study are due to direct inhibition of CYP 2E1, since DDTC was found to directly react with 4-VPO. Thus, further evidence was developed showing CYP 2F2 was responsible for the formation of 4-VPO in mouse lung. The third objective of this study was to evaluate the regioselectivity of glutathione (GSH) addition to 4-VPO. Using a synthetic standard of 4-VPO, two regio-isomers of 4-VPO-GSH conjugates were isolated and identified as R and S enantiomers of the C1-regioisomer of 4-VPO-GSH. The two enantiomers of the C2-4-VPO-GSH metabolites were isolated, but not fully characterized. There was no species or tissue difference in the regio- or stereoselectivity of 4VPO-GSH formation. This study provides better insight on the chemicals responsible for the lung toxicity seen with 4-VP and possibly styrene.
- Endpoint:
- basic toxicokinetics
- Type of information:
- read-across from supporting substance (structural analogue or surrogate)
- Adequacy of study:
- supporting study
- Reliability:
- 2 (reliable with restrictions)
- Rationale for reliability incl. deficiencies:
- data from handbook or collection of data
- Remarks:
- Data published in the peer reviewed literature.
- Principles of method if other than guideline:
- Metabolism of styrene and ethylbenzene was reported.
- Details on metabolites:
- While lung tumours from bronchiolar cell cytotoxicity are theoretically possible in humans, it is unlikely that metabolism by CYP2F1 would produce levels of cytotoxic metabolites in human lungs sufficient to result in lung cytotoxic responses and thus tumours. Therefore, it is unlikely several chemicals that cause mouse lung tumours via CYP2F2 metabolism will cause lung tumours in humans.
- Executive summary:
It is proposed that metabolism of several structurally-related chemicals by CYP2F isoforms of the cytochromes P450 family results in a cytotoxicity-driven mode of action in organs high in CYP2F; namely, CYP2F2 in nasal and lung tissue in mice and CYP2F4 in nasal tissues in rats. Importantly, the CYP2F1 isozyme expressed in humans appears to have a low capacity to metabolize these compounds. In mice, the resultant cytotoxicity and subsequent regenerative hyperplasia is hypothesized drive an increase in lung tumours that are mostly benign and are not life shortening. Although a complete picture of the mode of action has not been developed in any one model compound, data from the individual compounds can be combined to synthesize and reinforce confidence in the CYP2F toxicity hypothesis. For coumarin, naphthalene, and styrene, inhibition of toxicity with inhibition of CYP2F2 has been demonstrated. Rat CYP2F4 appears to be equally active in metabolizing these chemicals; however, CYP2F4 occurs to a much lower extent in rat Clara cells and levels of metabolites produced are not sufficient to cause lung cytotoxicity. Human lungs contain far fewer of Clara cells than rats or mice, and human lung microsomes failed to, or only marginally, metabolize these compounds. In addition, the human lung differs markedly from the mouse lung in the morphology of its Clara cells, which make humans much less sensitive than mice to toxicity due to reactive metabolites. The absence of a role for CYP2E1-generated metabolites (primarily alkyl oxidation vs. ring-oxidation) in mouse pulmonary effects was demonstrated by the lack of protection from styrene toxicity by CYP2E1 inhibitor, or reduction of toxicity in CYP2E1-knockout mice, and lack of lung toxicity of the primary metabolite of ethylbenzene. The chemicals used as examples of this mode of action generally are negative in standard genotoxicity assays. Apart from increased SCE, no consistent pattern in genotoxicity results was found among these chemicals. Thus, while lung tumours from bronchiolar cell cytotoxicity are theoretically possible in humans, it is unlikely that metabolism by CYP2F1 would produce levels of cytotoxic metabolites in human lungs sufficient to result in lung cytotoxic responses and thus tumours. Therefore, it is unlikely several chemicals that cause mouse lung tumours via CYP2F2 metabolism will cause lung tumours in humans.
- Endpoint:
- basic toxicokinetics in vivo
- Type of information:
- experimental study
- Adequacy of study:
- supporting study
- Study period:
- 1990
- Reliability:
- 2 (reliable with restrictions)
- Rationale for reliability incl. deficiencies:
- guideline study with acceptable restrictions
- Remarks:
- The study was not conducted according to guideline/s and there was no data on GLP but the report contains sufficient data for interpretation of study results.
- Objective of study:
- metabolism
- Principles of method if other than guideline:
- The disposition of mDVB was studied in male Fischer-344 rats after single doses of 40 mg of [14C]mDVB/kg (intravenous) and 40, 400 and 1200 mg of [14C]mDVB/kg (oral) and after repeated oral dosing at 400 mg of mDVB/kg/day for 11 days. In the repeated dosing study, [14C]mDVB was administered on days 3, 7 and 11. On other days, rats received unlabeled mDVB.
- GLP compliance:
- not specified
- Radiolabelling:
- yes
- Species:
- rat
- Strain:
- Fischer 344
- Sex:
- male
- Details on test animals or test system and environmental conditions:
- No data
- Route of administration:
- other: oral and intravenous
- Vehicle:
- not specified
- Duration and frequency of treatment / exposure:
- A single dose of 40 mg of 14C labeled m-DVB /kg (intravenous) and 40, 400, and 1200 mg of 14C labeled DVB/kg (oral) and after repeated oral dosing at 400 mg of m-DVB/kg/day for 11 days. In the repeated dosing study, 14C labeled m-DVB was administered on day 3, 7, and 11. On other days, rats received unlabeled m-DVB.
- Remarks:
- A single dose of 40 mg of 14C labeled m-DVB /kg (intravenous) and 40, 400, and 1,200 mg of 14C labeled DVB/kg (oral) and after repeated oral dosing at 400 mg of m-DVB/kg/day for 11 days. In the repeated dosing study, 14C labeled m-DVB was administered on day 3, 7, and 11. On other days, rats received unlabeled m-DVB. m-DVB/kg/day for 11 days. In the repeated dosing study, 14C labeled m-DVB was administered on day 3, 7, and 11. On other days, rats received unlabeled m-DVB.
- No. of animals per sex per dose / concentration:
- No data
- Control animals:
- not specified
- Positive control reference chemical:
- No data
- Details on study design:
- No data
- Details on dosing and sampling:
- No data
- Statistics:
- No data
- Details on excretion:
- In 72 hours, 72%, 76%, and 89% of the lowest, middle and highest single oral doses respectively, were excreted in urine.
- Metabolites identified:
- yes
- Details on metabolites:
- The major metabolite was isolated and purified by repeated chromatography. 13C-NMR analysis of purified fractions of this metabolite, is consistent with it being a mono-0-glucuronide conjugate of (3-ethenylphenyl)ethanediol.
- Conclusions:
- In 72 hours, 72%, 76%, and 89% of the lowest, middle and highest single oral doses respectively, were excreted in urine. Significant amounts of mDVB were secreted in bile after either oral or iv administration of mDVB. Repeated daily administration of oral doses of mDVB at 400 mg/kg produced evidence of induction of metabolizing enzymes by the third day. The major metabolite was isolated and purified by repeated chromatography. 13C-NMR analysis of purified fractions of this metabolite, is consistent with it being a mono-0-glucuronide conjugate of (3-ethenylphenyl)ethanediol.
- Executive summary:
Commercial mDVB is available as a mixture containing about 20% mDVB. mDVB for use in these studies was prepared by purification of commercial mDVB to a purity of > 90%. Carbon 14 labeled mDVB was obtained with a radiochemical purity of > 97%. Dose forms of mDVB were analyzed prior to each experiment. The disposition of mDVB was studied in male Fischer-344 rats after single doses of 40 mg of [14C]mDVB/kg (intravenous) and 40, 400 and 1200 mg of [14C]mDVB/kg (oral) and after repeated oral dosing at 400 mg of mDVB/kg/day for 11 days. In the repeated dosing study, [14C]mDVB was administered on days 3, 7 and 11. On other days, rats received unlabeled mDVB. In 72 h, 72%, 76% and 89% of the lowest, mid and highest single oral doses, respectively, were excreted in urine. Urinary excretion was most rapid for the lowest dose, least rapid for the highest dose. After intravenous administration, 82% of the dose was excreted in urine within 72 h. Excretion of carbon-14 in feces accounted for 10% of the intravenous dose, 20% of each of the two lower oral doses, but only 5%; of the highest oral dose. Less than 2% of the dose was excreted in breath for any dosing regimen. mDVB is well absorbed from the gut. The data suggest that either the 1200 mg/kg oral dose is more completely absorbed, and thus more completely excreted in urine than the lower doses or that more of the lower oral doses are secreted via bile back into the gut. Significant amounts of mDVB were secreted in bile after either oral or iv administration of mDVB. Repeated daily administration of oral doses of mDVB at 400 mg/kg produced evidence of induction of metabolizing enzymes by the third day. The rates of urinary excretion of the [14C]mDVB doses administered on days 3, 7 and 11 were all considerably higher than for a single dose. In the 12 h after dosing, rats excreted 56, 48 and 59%, respectively, of the doses on days 3, 7 and 11 as compared with 14% excreted in urime during the same time period after a single dose. Repeated dosing did not result in significant accumulation of the mDVB or its metabolites in tissues. Covalent binding of radiolabeled metabolites to cellular proteins may be expected if mDVB were epoxidized in the side chain in the same fashion as styrene. Investigation of protein binding by solvent extraction of tissues showed that 8-10% of radiolabel present in liver, kidney and lung is non-extractable and therefore may be covalently bound to cellular components of the tissue. HPLC analysis of the urine showed the presence of up to 12 mDVB metabolites, depending on dose and dosing route of mDVB. The major metabolite, accounting for 20-50% of the administered dose, was cleaved by treatment with beta-glucuronidase/sulfatase at pH 5. This conjugate also spontaneously hydrolyzed at pH 5. In general, the remaining urinary metabolites of mDVB were unaffected by beta-glucuronidase/sulfatase. The major metabolite was isolated and purified by repeated chromatography. 13C-NMR analysis of purified fractions of this metabolite, is consistent with it being a mono-0-glucuronide conjugate of (3-ethenylphenyl)ethanediol.
- Endpoint:
- basic toxicokinetics
- Type of information:
- experimental study
- Adequacy of study:
- supporting study
- Study period:
- 1996-1999
- Reliability:
- 2 (reliable with restrictions)
- Rationale for reliability incl. deficiencies:
- study well documented, meets generally accepted scientific principles, acceptable for assessment
- Remarks:
- Study published in the peer-reviewed literature.
- Objective of study:
- metabolism
- Principles of method if other than guideline:
- [14C]mDVB was incubated for up to 5 h with liver slices from male mice, rats, and humans.
- GLP compliance:
- not specified
- Radiolabelling:
- yes
- Species:
- other: rat, mice, human
- Strain:
- other: Fischer F-344, B6C3F1, n.a.
- Sex:
- male
- Details on test animals or test system and environmental conditions:
- Rodents
Source: Adult male Fischer (F-344) rats and B6C3F1 mice were purchased from Charles River Laboratories, Inc. (Raleigh, NC). Animals were quarantined at least one week before they were used in a study.
Diet: Certified Purina Rat Chow (5018) and tap water (City of Durham) were provided ad libitum
Housing: Prior to initiation of the experiments, animals were housed (maximum of 3 per cage) in polycarbonate cages with stainless steel bar lids accommodating a water bottle . Cage sizes were 19" x 10 1/2" x 8" high (143 sq. in floor space). Contact bedding was Ab-Sorb-Dri hardwood chips, Lab
Products, Maywood, NJ.
Environmental: Air circulation was 100% fresh filtered air with 10-15 air changes per hour. Fresh outside air was first drawn through an Am-Air 300 prefilter (American Air Filter; Louisville, Kentucky) then through a #6-52C20 High-Flow Filter (Cambridge, Inc ; Syracuse, NY) . Room temperature was maintained at 69-76'F and relative humidity at 40-70% and monitored at least once a day. Lighttdarkness was cycled at 12 h intervals . Environmental parameters were recorded automatically using a Barber- Colman Network 8000 HVAC Monitoring and Control System (Barber-Colman Company, Loves Park, IL).
Human Liver Slices
Human liver slices were received from the International Institute for the Advancement of Medicine. The first donor was a 45-year-old black male that died from a gunshot wound to the leg . He had renal cancer and used cocaine. Medications received during the hospital stay were DDAVP, Cefadyl and dopamine. The second donor was an 18-year-old white male that died from a blunt head injury. He used ethanol socially and smoked three packs of cigarettes a week. The latter subject received lasix, mannitol, neomycin, erythromycin, nystatin, pepcid, timentin, rocephine, motrin, tylenol, and ancef during his hospital stay. Human liver slices from a 50-year-old white male were received from Human Cell Culture Center, Inc (HCCC). This donor had gout as a medical condition and died from intracranial hemorrhage. He received propofol, neuromax, ephedrine and heparin during his hospital stay. - Route of administration:
- oral: gavage
- Vehicle:
- corn oil
- Details on exposure:
- In Vitro (Liver Slice Incubation )
Krebs-Henseleit (KH) buffer preparation (Sigma) was fortified by addition of CaC12 (Fisher), sodium bicarbonate (Fisher) and HEPES (Sigma). The pH was adjusted to 7.4 with 1 N NaOH and chilled to 40°C overnight. On the day of the incubation, amino acids were added to a portion of the modified KH buffer (KHaa). A solution of [14C]mDVB was prepared with the KHaa. Three male F-344 rats and four male B6C3F1 mice were terminally anesthetized with carbon dioxide. The livers were excised and placed in cold (40°C) modified KH buffer. All tissue handling and slicing operation were performed in modified KH buffer at 40°C . The liver was removed and 9 mm diameter cores were cut with a cork borer. Liver slices of approximately 380 lum thickness were prepared with a Krumdieck Tissue Slicer (K and F Research Corporation, Birmingham, AL). Human liver slices were obtained from IIAM or HCCC, and had been sliced to a thickness of 250 gm and were 8 mm in diameter. Slices from each liver were placed into individual wells of 12-well tissue culture plates in 1 mL cold modified KHaa. At the same time, 1 mL of a solution of [14C]mDVB (in KHaa) was added to each of eighteen 20 mL capped scintillation vials. The plates and the vials were placed onto an orbital agitator (115 -120 rpm) in a cabinet incubator to pre-incubate at 370°C for 30 min. Following pre-incubation, slices from each liver were transferred to the capped vials containing the [14C]mDVB solution . Slices from each liver (duplicates, except in the case of mouse 1 where only a single slice was available) were incubated for 1, 2 .25 and 5 h at ca . 370°C rotating at 115 - 120 rpm. At the end of the incubation time periods, the incubation mixtures were cooled to 40°C. After cooling, the liver slices were removed from the incubation media.
In Vivo (Rat Oral Dose )
The oral dose formulation contained [14C]mDVB dissolved in corn oil combined with additional nonradiolabeled mDVB at target dose level of 1200 mg mDVB per kg body weight. The formulation was administered in a dose volume of 5 mUkg to a 273 g male Fischer (F-344) rat . Following, dosing, the animal was housed in an all glass metabolism cage . Urine was collected at 9, 18, 24, 42 and 48 h intervals The weight of urine collected for each sample interval was measured. - Metabolites identified:
- yes
- Details on metabolites:
- Parent mDVB remained the predominant xenobiotic in the incubation mixture over the duration of the experiment. Maximum rates of metabolism of mDVB were 25, 38 and 24 nmoles per hour, respectively, for liver slices from mice, rats, and humans. Analysis of incubation media and liver slice extracts from each species by HPLC showed that each produced the same metabolites eluting in two major and one minor fraction. Liver slices from three human subjects produced the same metabolites of mDVB but at different rates, due possibly to differing lifestyles of the three individuals or to differing sections of the liver from which the slices originated. Comparison of the metabolites formed by the liver slices with metabolites isolated from urine has led to the identification of one of the major metabolites formed by liver slices as 1-(3-ethenylphenyl)ethane-1,2-dioI (mDVB-diol). Both positional isomers of the monoglucuronide conjugate of mDVB-diol were isolated from male Fischer (F-344) rat urine and cleaved to the conjugate mDVB-diol. The second major metabolite is not found among the mDVB metabolites excreted in urine. Since mDVB is excreted primarily in urine, it is unlikely that this second metabolite is formed in substantial amounts in vivo. The identity of the more polar major mDVB metabolite was not established because of the small mass formed by liver slices. Treatment of this metabolite with sulfatase caused slight polarity changes in the metabolite, but it is not a sulfate conjugate of mDVB-diol. Analysis of the metabolite by LC/MS/MS and its reaction product by GC/MS did not provide data from which the structure could be deduced.
- Executive summary:
[14C]mDVB, randomly labeled in the aromatic ring, was isolated from residual oral dose formulation prepared under the previous NIEHS contract (NOl-ES-65137). The radiochemical purity of the (14C]mDVB so isolated was determined to be greater than 97%. [14C]mDVB at concentrations of 0 3-03 mM was incubated for up to 5 h with liver slices from male mice, rats, and humans. For mice and rats, the overall recovery of carbon-14 content from the incubations was >90%, with ca. 80% recovered in the media, and means of 12% and 17% recovered from the liver slices from mouse and rat, respectively. The overall recovery of carbon-14 content from human liver slice incubations was also >90%, with means of 7%, 16% and 25% recovered from the liver slices for subjects 1, 2 and 3, respectively. Under the selected incubation conditions, parent mDVB remained the predominant xenobiotic in the incubation mixture over the duration of the experiment. Maximum rates of metabolism of mDVB were 25, 38 and 24 nmoles per hour, respectively, for liver slices from mice, rats, and humans. Analysis of incubation media and liver slice extracts from each species by HPLC showed that each produced the same metabolites eluting in two major and one minor fraction. Liver slices from three human subjects produced the same metabolites of mDVB but at different rates, due possibly to differing lifestyles of the three individuals or to differing sections of the liver from which the slices originated. To obtain sufficient quantities of potential metabolites for identification purposes, urine was collected from a single rat following a single oral dose of 1200 mg/kg of [14C]mDVB. Over 48 hours, 79% of the administered radioactivity was excreted in urine. The HPLC profile of the urinary metabolites is identical to that obtained in a previous study of mDVB in rats. Comparison of the metabolites formed by the liver slices with metabolites isolated from urine has led to the identification of one of the major metabolites formed by liver slices as 1-(3-ethenylphenyl)ethane-1,2-dioI (mDVB-diol). Both positional isomers of the monoglucuronide conjugate of mDVB-diol were isolated from male Fischer (F-344) rat urine and cleaved to the conjugate mDVB-diol. The second major metabolite is not found among the mDVB metabolites excreted in urine. Since mDVB is excreted primarily in urine, it is unlikely that this second metabolite is formed in substantial amounts in vivo. The identity of the more polar major mDVB metabolite was not established because of the small mass formed by liver slices. Treatment of this metabolite with sulfatase caused slight polarity changes in the metabolite, but it is not a sulfate conjugate of mDVB-diol. Analysis of the metabolite by LC/MS/MS and its reaction product by GC/MS did not provide data from which the structure could be deduced.
- Endpoint:
- basic toxicokinetics in vivo
- Type of information:
- experimental study
- Adequacy of study:
- supporting study
- Study period:
- 1989
- Reliability:
- 2 (reliable with restrictions)
- Rationale for reliability incl. deficiencies:
- study well documented, meets generally accepted scientific principles, acceptable for assessment
- Remarks:
- Study published in the peer-reviewed literature.
- Objective of study:
- metabolism
- Principles of method if other than guideline:
- Animals were dosed i.p. with a single injection of the test substance in olive oil. Urine samples were collected after 24 and 48 hours.
- GLP compliance:
- not specified
- Radiolabelling:
- not specified
- Species:
- rat
- Strain:
- Wistar
- Sex:
- female
- Details on test animals or test system and environmental conditions:
- Adult female Wistar rats (VELAZ, Czechoslovakia), weighing 220-280 g, were kept in glass metabolic cages (Kavalier, Czechoslovakia) with pelleted food (VELAZ) and drinking water ad libitum. Animals were divided into four groups, five animals in each, and dosed i.p. with a single injection of 100, 200 or 300 mg/kg of 1,4-diethenylbenzene in olive oil; the control group received olive oil only. Urine samples were collected after 24 and 48 hours. Urine was rinsed from the walls of metabolic cages and diluted with water to 15 mL or more, if needed, and stored at -18°C. Another 15 rats were used for isolation of the metabolites; these animals were given a single i.p. dose of the diethenylbenzene (300 mg/kg) under the same conditions as described above.
- Route of administration:
- intraperitoneal
- Vehicle:
- olive oil
- Details on exposure:
- Animals were divided into four groups, five animals in each, and dosed i.p. with a single injection of 100, 200 or 300 mg/kg of 1,4-diethenylbenzene in olive oil; the control group received olive oil only. Urine samples were collected after 24 and 48 hours. Urine was rinsed from the walls of metabolic cages and diluted with water to 15 mL or more, if needed, and stored at -18°C. Another 15 rats were used for isolation of the metabolites; these animals were given a single i.p. dose of the diethenylbenzene (300 mg/kg) under the same conditions as described above.
- Duration and frequency of treatment / exposure:
- single administration
- Dose / conc.:
- 100 other: mg/kg
- Dose / conc.:
- 200 other: mg/kg
- Dose / conc.:
- 300 other: mg/kg
- No. of animals per sex per dose / concentration:
- 5 animals per dose
- Control animals:
- yes, concurrent vehicle
- Positive control reference chemical:
- No data
- Details on study design:
- Animals were divided into four groups, five animals in each.
- Details on dosing and sampling:
- Animals were divided into four groups, five animals in each, and dosed i.p. with a single injection of 100, 200 or 300 mg/kg of 1,4-diethenylbenzene in olive oil; the control group received olive oil only. Urine samples were collected after 24 and 48 hours. Urine was rinsed from the walls of metabolic cages and diluted with water to 15 mL or more, if needed, and stored at -18°C. Another 15 rats were used for isolation of the metabolites; these animals were given a single i.p. dose of the diethenylbenzene (300 mg/kg) under the same conditions as described above.
- Statistics:
- No data
- Metabolites identified:
- yes
- Details on metabolites:
- DVB was oxidized to an electrophilic oxirane which was attached by glutathione to form conjugates which were further metabolized through the mercapturic-acid pathway to form mercapturic acids. Another important pathway involved the epoxide-hydrolase catalyzed hydration of the oxirane to form a glycol which was further oxidatively metabolized to vinyl mandelic, vinylphenylglyoxylic and vinylbenzoic acids. A minor metabolic pathway involved the rearrangement of the oxirane to a vinyl phenylacetaldehyde which was further oxidized to vinylphenyl acetic acid and its conjugate with glycine. The structures of the identified metabolites indicate that the main reactive intermediate in the metabolism of p-DVB is p-vinylphenyloxirane.
- Conclusions:
- The structures of the identified metabolites indicate that the main reactive intermediate in the metabolism of p-DVB (1,4-diethenylbenzene) is p-vinylphenyloxirane. The first step in the biotransformation of p-DVB, formation of an oxirane, is very similar to the metabolic activation of styrene.
- Executive summary:
Biotransformation of 1,4 -diethenylbenzene in rat was studied. Six urinary metabolites were isolated and identified: N-acetyl-S-[2-(4-ethenylphenyl)-2-hydroxyethyl]-L-cysteine, N-acetyl-S-[1-(4-ethenylphenyl)-2-hydroxyethyl]-L-cysteine, N-acetyl-S-[1-(4 -formylphenyl)-2-hydroxyethyl]-L-cysteine, 1-(4 -ethenylphenyl)ethane-1,2-diol, 4-ethenylbenzoic acid and 4-ethenylbenzoyl-glycine. In addition, g.l.c.-mass spectral analysis of the methylated urine extract allowed the identification of four other metabolites as: 4-ethenylphenylacetic acid, 4-ethenylphenylacetylglycine, 4-ethenylmandelic acid, and 4-ethenylphenylglyoxylic acid. The structures of the identified metabolites indicate that the main reactive intermediate in the metabolism of 1,4-diethenylbenzene is 4-ethenylphenyloxirane. The first step in the biotransformation - the formation of an oxirane - is very similar to the metabolic activation of styrene. However, subsequent steps lead not only to analogues of styrene metabolites but also to oxidation of the second ethenyl group leading to compound(s) which may contribute to the toxicity of 1,4-diethenylbenzene, e.g. to the N-acetyl-S-[1-(4-formylphenyl)-2-hydroxyethyl]-L-cysteine. Rats dosed with a single i.p. dose of 1,4-diethenylbenzene excreted nearly 5 -6% of the dose as glycine conjugate, irrespective of the dose. In contrast, the total thioether fraction decreased significantly with increasing dose, being 23, 17 and 12% of the dose at 100, 200 and 300 mg/kg, respectively.
- Endpoint:
- basic toxicokinetics in vivo
- Type of information:
- experimental study
- Adequacy of study:
- supporting study
- Study period:
- 1990
- Reliability:
- 2 (reliable with restrictions)
- Rationale for reliability incl. deficiencies:
- study well documented, meets generally accepted scientific principles, acceptable for assessment
- Remarks:
- Study published in the peer-reviewed literature.
- Principles of method if other than guideline:
- The metabolism of 1,4-diethenylbenzene in the rat was followed by gas chromatographic-mass spectrometric analysis of urine.
- Details on metabolites:
- Fifteen metabolites were found in the urine of rats dosed with a single intraperitoneal injection of 1,4-diethenylbenzene (300 mg/kg). Nine of them were identified in a previous study [Lindhart I et al. (1989). Xenobiotica, 19:645], but the other six have not previously been reported. New metabolites, namely, 1-ethenyl-4-(1-hydroxyethyl)benzene, 4-(1,2-dihydroxyethyl)benzoic acid, (4-carboxymethylphenyl)acetylglycine, N-acetyl-S-[2-carboxy-1-(4-ethenylphenyl)ethyl]-L-cysteine, and two isomeric beta-D-glucosiduronates derived from 1-(4-ethenylphenyl)ethane-1,2-diol, were identified by mass spectrometry of their derivatives and comparison of them with the spectra of analogous metabolites of styrene and 4-methylstyrene.
- Executive summary:
The metabolism of 1,4-diethenylbenzene in the rat was followed by gas chromatographic-mass spectrometric analysis of urine using three different derivatization procedures: (i) methylation-acetylation; (ii) methylation-trimethylsilylation; (iii) methylation followed by conversion into trimethylsilyloximes. Fifteen metabolites were found in the urine of rats dosed with a single intraperitoneal injection of 1,4-diethenylbenzene (300 mg/kg). Nine of them were identified in our previous study [I. Lindhart et al., Xenobiotica, 19 (1989) 645], but the other six have not previously been reported. New metabolites, namely, 1-ethenyl-4-(1-hydroxyethyl)benzene, 4-(1,2-dihydroxyethyl)benzoic acid, (4-carboxymethylphenyl)acetylglycine, N-acetyl-S-[2-carboxy-1-(4-ethenylphenyl)ethyl]-L-cysteine, and two isomeric beta-D-glucosiduronates derived from 1-(4-ethenylphenyl)ethane-1,2-diol, were identified by mass spectrometry of their derivatives and comparison of them with the spectra of analogous metabolites of styrene and 4-methylstyrene. Acetylation of methylated urine extracts seems to be the most suitable derivatization procedure, but a combination of at least two procedures is needed if the virtually complete metabolic pattern of diethenylbenzene is to be obtained. Possible routes of biotransformation leading to the newly identified metabolites are discussed.
- Endpoint:
- basic toxicokinetics
- Type of information:
- experimental study
- Adequacy of study:
- supporting study
- Study period:
- 1992
- Reliability:
- 2 (reliable with restrictions)
- Rationale for reliability incl. deficiencies:
- study well documented, meets generally accepted scientific principles, acceptable for assessment
- Remarks:
- Study published in the peer-reviewed literature.
- Principles of method if other than guideline:
- Biotransformation of the test substance was reported.
- Details on metabolites:
- Biotransformation of 1,3-diethenylbenzene in rat gave four major metabolites, namely, 3-ethenylphenylglyoxylic acid; 3-ethenylmandelic acid; N-acetyl-S-[2-(3-ethenylphenyl)-2-hydroxyethyl]-L-cysteine; and N-acetyl-S-[1-(3-ethenylphenyl)-2-hydroxyethyl]-L-cysteine. Four minor metabolites, 3-ethenylbenzoic acid; 3-ethenylphenylacetic acid; 3-ethenylbenzoylglycine; and 2-(3-ethenylphenyl)ethanol were identified.
- Executive summary:
Biotransformation of 1,3-diethenylbenzene (1) in rat gave four major metabolites, namely, 3-ethenylphenylglyoxylic acid (2), 3-ethenylmandelic acid (3), N-acetyl-S-[2-(3-ethenylphenyl)-2-hydroxyethyl]-L-cysteine (4) and N-acetyl-S-[1-(3-ethenylphenyl)-2-hydroxyethyl]-L-cysteine (5) were isolated from urine and identified by n.m.r. and mass spectrometry. Four minor metabolites, 3-ethenylbenzoic acid (6), 3-ethenylphenylacetic acid (7), 3-ethenylbenzoylglycine (8) and 2-(3-ethenylphenyl)ethanol (9) were identified by g.l.c.-mass spectrometric analysis of urine extract derivatized in two different ways. All identified metabolites are derived from 3-ethenylphenyloxirane (10), a reactive metabolic intermediate. No product of any metabolic transformation of second ethenyl group has been identified. However, several minor unidentified metabolites were detected by g.l.c.-mass spectrometry. Total thioether excretion in 24 h urine after a single i.p. dose of 1 amounted to 28.3 ± 3.5 dose (mean ± SD). No significant differences in the thioether fraction were observed in the dose range 100-300 mg/kg. Thioether metabolites consisted mainly of mercapturic acids 4 and 5. The ratio of metabolites 5 to 4 was 62:38. Each mercapturic acid consisted of two diastereomers. Their ratio, as determined by quantitative 13C-n.m.r. measurement was 95:5 and 79:21 for mercapturic acids 4 and 5, respectively.
- Endpoint:
- basic toxicokinetics
- Type of information:
- experimental study
- Adequacy of study:
- supporting study
- Reliability:
- 2 (reliable with restrictions)
- Rationale for reliability incl. deficiencies:
- study well documented, meets generally accepted scientific principles, acceptable for assessment
- Remarks:
- Data published in the peer-reviewed literature.
- Principles of method if other than guideline:
- A simple ion-suppression separation on reversed-phase columns, which is applicable for both analytical and semi-preparative work, was described.
- Metabolites identified:
- yes
- Details on metabolites:
- Six urinary metabolites of 1,3-diethenylbenzene (I), namely 1-(3-ethenylphenyl)-1,2-dihydroxyethane beta-D-glucosiduronates (two isomers, II and III), N-acetyl-S-[1-(3-ethenylphenyl)-2-hydroxyethyl]cysteine (IV), N-acetyl-S-[2-(3-ethenylphenyl)-2-hydroxyethyl]cysteine (V), 3-ethenylphenylmandelic acid (VI) and 3-ethenylphenylglyoxylic acid (VII), were isolated.
- Executive summary:
A simple ion-suppression separation on reversed-phase columns, which is applicable for both analytical and semi-preparative work, is described. Six urinary metabolites of 1,3-diethenylbenzene (I), namely 1-(3-ethenylphenyl)-1,2-dihydroxyethane beta-D-glucosiduronates (two isomers, II and III), N-acetyl-S-[1-(3-ethenylphenyl)-2-hydroxyethyl]cysteine (IV), N-acetyl-S-[2-(3-ethenylphenyl)-2-hydroxyethyl]cysteine (V), 3-ethenylphenylmandelic acid (VI) and 3-ethenylphenylglyoxylic acid (VII), were isolated (Fig. 1). Four of them, IV-VII, have been identified in previous work; the two glucosiduronates were identified for the first time by 1H NMR spectroscopy, fast atom bombardment mass spectrometry, and enzymic hydrolysis yielding 1-(3-ethenylphenyl)-1,2-dihydroxyethane as an aglycone. The method was reproducible the concentration range 0.05-5 mg/mL, the coefficient of variation being less than 7% (n = 5). Excretion of II-VI within 24 hours in the urine of rats dosed with a single intraperitoneal injection of 100, 300 and 600 mg/kg I was determined quantitatively. The utility of the method is discussed in comparison with gas chromatographic-mass spectrometric techniques used previously.
- Endpoint:
- basic toxicokinetics in vivo
- Type of information:
- experimental study
- Adequacy of study:
- supporting study
- Reliability:
- 2 (reliable with restrictions)
- Rationale for reliability incl. deficiencies:
- study well documented, meets generally accepted scientific principles, acceptable for assessment
- Remarks:
- The study was not conducted according to guideline/s and there was no data on GLP but the report contains sufficient data for interpretation of study results.
- Objective of study:
- metabolism
- Principles of method if other than guideline:
- Rats were exposed to the test substance with a single ip injection at 100, 300 and 500 mg/kg in sunflower oil (2 mL/kg).
- GLP compliance:
- not specified
- Radiolabelling:
- not specified
- Species:
- rat
- Strain:
- Wistar
- Sex:
- male
- Route of administration:
- intraperitoneal
- Vehicle:
- other: sunflower oil
- Details on exposure:
- A single ip injection; 100, 300 and 500 mg/kg in sunflower oil (2 mL/kg).
- Duration and frequency of treatment / exposure:
- Single dose
- Dose / conc.:
- 100 other: mg/kg
- Dose / conc.:
- 300 other: mg/kg
- Dose / conc.:
- 500 other: mg/kg
- No. of animals per sex per dose / concentration:
- 5 male rats at100 or 300 mg/kg
6 male rats at 500 mg/kg - Control animals:
- yes, concurrent vehicle
- Positive control reference chemical:
- No data
- Metabolites identified:
- yes
- Details on metabolites:
- The key reactive metabolic intermediate appeared to be o-vinylphenyloxirane, which is further extensively metabolized by two major pathways, namely
(1) epoxide hydrolyse-catalysed hydrolysis to diol
(2) glutathione conjugation. A minor pathway leading to vinylphenaceturic acid. - Conclusions:
- Like other DVB isomers, the o-DVB shows a metabolic pattern similar to that of styrene. The key reactive metabolic intermediate appeared to be o-vinylphenyloxirane, which is further extensively metabolized by two major pathways, namely
(1) epoxide hydrolyse-catalysed hydrolysis to diol and
(2) glutathione conjugation. A minor pathway leading to vinylphenaceturic acid.
The total excretion of urinary thioethers after exposure to o-DVB was the lowest of all DVB isomers and styrene. - Executive summary:
Like other DVB isomers, the o-DVB shows a metabolic pattern similar to that of styrene. The key reactive metabolic intermediate appeared to be o-vinylphenyloxirane, which is further extensively metabolized by two major pathways, namely
(1) epoxide hydrolyse-catalysed hydrolysis to diol and
(2) glutathione conjugation. A minor pathway leading to vinylphenaceturic acid.
The total excretion of urinary thioethers after exposure to o-DVB was the lowest of all DVB isomers and styrene.
Biotransformation of 1,2-diethenylbenzene (1) in rat was studied. Five urinary metabolites were isolated by extraction of acid hydrolysed urine and identified by nmr and mass spectroscopy, namely, 1-(2-ethenylphenyl)ethane-1,2-diol (2) 2-ethenylmandelic acid (3), 2-ethenylphenylglyoxylic acid (4), 2-ethenylphenylacetylglycine (5) N-acetyl-S-[1-(2-ethenylphenyl)-2-hydroxyethyl]cysteine (6) and N-acetyl-S-[2-(2-ethenylphenyl)-2-hydroxy-ethyl]cysteine (7). In addition, minor metabolites, namely, 2-ethenylbenzoic acid (8) and 2-ethenylphenyl-acetic acid (9) were identified by glc-mass spectral analysis of the hydrolysed urine extract treated subsequently with diazomethane, hydroxylamine and a trimethyl-silylating reagent. Several compounds, which could arise from biotransformation of both ethenyl groups in the molecule of 1, were detected but not identified unequivocally. A glucuronide was detected by tlc analysis of urine as a blue spot after spraying with naphthoresorcinol. Compounds showing molecular fragments indicating the glucuronide moiety were also detected by glc-mass spectroscopy in non-hydrolysed urine samples. The total thioether excretion amounted to 5.3 ± 2.4, 5.1 ± 3.4 and 5.0 ± 1.9% of the dose at 500, 300 and 100 mg/kg, respectively (mean ± SD; n = 5). Like styrene and other diethenylbenzene isomers, 1,2-diethenylbenzene is metabolically activated to a reactive epoxide intermediate, 2-ethenylphenyloxirane (10), which is further converted to the urinary metabolites mentioned above. The main detoxification pathways are hydrolysis to the glycol 2 followed by several oxidation steps, and conjugation with glutathione. The latter reaction is both regioselective and stereoselective. The ratio of mercapturic acids 6:7 was 83:17. Each regioisomer consists of two diastereomers which show distinct resonance signals in the 13C-nmr. The diastereomer ratio was 82:28 and 79:21 for 6 and 7 respectively.
Referenceopen allclose all
Table 1: Conversion of 2-Ethylphenol to Ethylhydroquinone after Incubation with Liver and Lung Microsomes from Mouse, Rat, and Human Tissues
Table 2: Conversion of 4-Ethylphenol to Ethylcatechol after Incubation with Liver and Lung Microsomes from Mouse, Rat, and Human Tissues
Table 3: Results of Time-Course Experiments to Assess Linearity of Metabolite Formation (conversion of EB to 1-Phenylethanol and Acetophenone
Table 4: Results of Time-Course Experiments to Assess Linearity of MetaboliteFormation (conversion of EB to 2,5- and 3,4-ethylquinone via GSH trap
Table 5: Percent Conversion of Metabolites Formed in Incubations of Ethylbenzene with Liver Microsomes
Table 6: Percent Conversion of Metabolites Formed in Incubations of Ethylbenzene with Lung Microsomes
Table 7. Km and Vmax Values Derived for Metabolites whose Rates of Conversion Increase with Increasing Substrate Concentration: Values for Formation of 1 -Phenylethanol and Acetophenone (Top), and 2EP-GSH and 4EP-GSH (Bottom)
Table 8: Maximum and Minimum Rates of Formation (μmole/mg protein/minute) for Metabolites whose Rates of Conversion Decrease with Increasing Substrate Concentration
Styrene: the first step in the major metabolic pathway is oxidation to S-styrene-7,8-oxide (S-SO); this accounts for at least 80% of the metabolism of styrene in rats and mice (Sumner and Fennell, 1994; Cruzan et al., 2002). It should be noted that oral administration of styrene-7,8-oxide to mice at 275 mg/kg/day did not result in increased lung tumours (Lijinsky, 1986), even though PBPK models indicate this dose of SO would result in a higher lung level of SO than from metabolism of styrene at 40 ppm by inhalation (Sarangapani et al., 2002), a concentration that has resulted in pulmonary tumours in mice. Further, Hofmann et al. (2006) demonstrated that ex vivo exposure to styrene in rat lungs at 1000 ppm (non-tumorigenic) produced 2.5 nmol styrene oxide/g lung vs. 0.25 from mouse lungs at styrene concentration of 40 ppm (tumorigenic). This led the authors to conclude that styrene-7,8-oxide is not the agent responsible for mouse lung cytotoxicity from styrene exposure. In mouse lung, two alternate metabolic paths are prevalent; one involves formation of R-styrene-7,8-oxide and the other involves oxidation of the benzene ring (Cruzan et al., 2002; Bartels et al., 2005). Using selective inhibitors, Carlson determined that CYP1A, 2B, and 2E1 had little, if any, impact on Clara cell cytotoxicity of styrene, implying they are not involved in metabolic activation of styrene in the lung (Carlson, 1997; Carlson et al., 1998). Inhibition of 2E1, or the use of 2E1-knockout mice demonstrated that 2E1 plays some role in the acute liver cytotoxicity of styrene but has no impact on the lung toxicity (Carlson, 2004; Vogie et al., 2004). In studies of styrene, the inhibition of CYP2F2 by 5-phenyl- 1-pentyne (5P1P) inhibited both the lung and nasal cytotoxicity in CD-1 mice (Green et al., 2001a,b). 4-Vinylphenol (4-VP, 4-hydroxystyrene), although a minor urinary metabolite of styrene, has been examined for its lung toxicity potential. 4-VP is 10 times as toxic to mouse lung as styrene and five times as toxic as styrene-7,8-oxide (Carlson et al., 2002). Inhibition of CYP2F2 by 5P1P also inhibited the cytotoxicity (66–87%) of 4-VP (Carlson, 2002), indicating that there is a subsequent metabolite of 4-VP that is responsible for cytotoxicity. The metabolite(s) responsible for cytotoxicity from these compounds in the olfactory epithelium or terminal bronchioles have not been identified.
Ethylbenzene: in vitro studies examining comparative mouse, rat and human lung and liver microsomal metabolism of ethylbenzene have confirmed extensive metabolism in all three species to alkyl-oxidized metabolites, e.g., 1-phenylethanol (mouse > rat ~ human; Saghir et al., 2006, 2009). 1-Phenylethanol was not pneumotoxic or tumorigenic when tested in high-dose oral subchronic and chronic rat and mouse bioassays (NTP, 1990). No detectable lung toxicity was found from exposure to 1-phenylethanol, 2-phenylethanol, or phenylacetaldehyde in mice (Carlson et al., 2002). However, in vitro studies suggested possible formation of potentially lung-toxic reactive ring-oxidized metabolite(s) (Saghir et al., 2009). Use of GSH-trapping to detect putative cytotoxic catechol and hydroquinone metabolites confirmed the in vitro formation of these metabolites in mouse, rat and human liver microsomes, and in mouse and rat, but not human, lung microsomes. Similar to the generation of alkyl-oxidized metabolites, mouse lung microsomes exhibited substantially higher metabolic activity (mouse lung GSH-derived metabolites approximately 10x > rat lung; human lung not detectable; mouse lung GSH metabolites approximately 2x > mouse liver; mouse liver approximately 10x > rat and human liver). Although ring-oxidized metabolites accounted for a relatively small fraction of overall ethylbenzene metabolism, their selective elevation in mouse lung microsomes is nonetheless consistent with the hypothesized mode of action attributing preferential formation of lung-derived cytotoxic, ring-oxidized metabolites as driving the mouse lung specific toxicity of ethylbenzene. Interestingly, both mouse and rat lung microsomes exhibited decreasing amounts of ring-oxidized metabolite formation with increasing concentrations of ethylbenzene, suggesting the possibility of cytochrome P450 suicide inhibition by reactive ring-oxidized metabolite(s). This observation would also be consistent with the hypothesis of the formation of reactive cytotoxic metabolites in mouse lung. 4-Hydroxyethyl-benzene is the only metabolite of ethylbenzene that has been demonstrated to cause mouse lung cytotoxicity in 3-day studies (Kaufmann et al., 2005); no cytotoxicity occurred when 1-phenylethanol was administered.
The major routes in the metabolism of diethenylbenzene are analogous to those of styrene. Thus, is extensively oxidised to 4-ethenylphenyloxirane, a reactive metabolic intermediate. The electrophilic oxirane is attacked non-regioselectively with glutathione. The resulting glutathione conjugates are further metabolized through the mercapturic acid pathway. The resulting mercapturic acids bear two asymmetric carbon atoms in their molecules and therefore consist of two diastereomers. The ratio of diastereomers as determined by quantitative 13C-NMR measurement was 53:47 and 66:34 in the major mercapturic acids N-acetyl-S-[2 -(4 -ethenylphenyl)-2-hydroxyethyl]-L-cysteine and N-acetyl-S-[1-(4-ethenylphenyl)-2-hydroxyethyl]-Lcysteine, respectively. In the mercapturic acid N-acetyl-S-[1-(4-formylphenyl)-2-hydroxyethyl]-L-cysteine the ratio of diastereomers was not determined. Another important metabolic pathway is that of epoxide-hydrolase-catalyzed hydration of the oxirane leading to formation of 1-(4 -ethenylphenyl)ethane-1,2-diol which is further oxidatievly metabolized to 4-ethenylmandelic acid, 4-ethenyl-glyoxylic acid and 4-ethenylbenzoic acid. A rearrangement of the oxirane to 4-ethenyl-phenylacetaldehyde is a minor metabolic pathway. The intermediary aldehyde is metabolized oxidatively to 4-ethenyl-phenylacetic acid. The acids 4-ethenylbenzoic acid and 4-ethenylbenzoyl-glycine are conjugated with glycine to form vinyl analogues of hippuric and phenaceturic acids and respectively. The major metabolites N-acetyl-S-[2-(4-ethenylphenyl)-2-hydroxyethyl]-L-cysteine, N-acetyl-S-[1-(4-ethenylphenyl)-2-hydroxyethyl]-L-cysteine, 1-(4 -ethenylphenyl)ethane-1,2-diol, 4-ethenylbenzoic acid and 4-ethenylbenzoyl-glycine were isolated from rat urine and identified using NMR and mass spectra. In addition, some minor metabolites, i.e. carboxylic acids were identified by g.l.c.-mass spectral analysis of the urine extracts. All these metabolites are vinyl analogues of known styrene metabolites.
Like other DVB isomers, the o-DVB shows a metabolic pattern similar to that of styrene. The key reactive metabolic intermediate appeared to be o-vinylphenyloxirane, which is further extensively metabolized by two major pathways, namely
(1) epoxide hydrolyse-catalysed hydrolysis to diol and
(2) glutathione conjugation. A minor pathway leading to vinylphenaceturic acid.
The total excretion of urinary thioethers after exposure to o-DVB was the lowest of all DVB isomers and styrene.
Description of key information
Several in vivo absorption, distribution, metabolism, elimination (ADME) studies, as well as one comparative in vitro metabolism study have been conducted with different isomers of divinylbenzene. In addition, read-across data with ethylbenzene and styrene are available.
Key value for chemical safety assessment
Additional information
Several in vivo absorption, distribution, metabolism, elimination (ADME) studies, as well as one comparative in vitro metabolism study have been conducted with different isomers of divinylbenzene. In addition, several read-across studies with ethylbenzene and styrene are available. Divinylbenzene (diethenylbenzene, DVB) consists primarily of two isomers: 1,4- and 1,3- also defined as pDVB and mDVB, respectively. The 1,2- (oDVB) isomer is a minor component in commercial DVB. These DVB isomers are structurally related to styrene (vinylbenzene) and ethylbenzene.
Several in vivo absorption, distribution, metabolism, elimination (ADME) studies, as well as one comparative in vitro metabolism study have been conducted with DVB. Linhart et al. identified numerous metabolites arising from oxidation and subsequent conjugation of one of the vinyl moieties of 1,4-DVB (Linhart, Hanus et al. 1989; Linhart and Novak 1990). These metabolites, except for one unique aldehyde metabolite, were all vinyl analogues of known styrene metabolites. Additional studies with the 1,3- and 1,2-DVB isomers also afforded similar results (Jeffcoat A.R. 1990; Linhart, Mitera et al. 1992; Linhart, Vosmanska et al. 1992; Linhart, Weidenhoffer et al. 1996). An in vitro study comparing the relative rates and routes of metabolism of 1,3-DVB was conducted (Jeffcoat A.R. 1999). Radiolabelled 1,3-DVB was incubated with liver tissue slices from rat, mouse and human donors. Metabolites were characterized by HPLC analysis at 1, 2.25 and 5 hr. Three common metabolites were identified in incubates from all three species. The least polar metabolite was identified as the 1,2-diol of 1,3-DVB, also identified as an in vivo metabolite in rat urine, and consistent with the analogous 1,2-glycol metabolite of styrene. The remaining two metabolites were unidentified, but the most polar one was characterized as a possible sulphate conjugate. The rate, or amount of metabolism was fairly comparable between species, with 12.4, 23.2 and 25.3% of the substrate metabolized within 5 hr in mouse, rat and human tissue, respectively. These studies with DVB represent primarily hepatic metabolism. No reports of non-hepatic ADME experiments have been found. However, related studies with styrene and ethylbenzene have been conducted to evaluate the metabolic fate and enzyme systems responsible for major and reactive metabolite formation of these two compounds in lung tissue. Data on the metabolic fate of 4-hydroxystyrene (4-vinylphenol; 4-VP) in liver tissue of mouse as well as lung tissue of mouse, rat and human donors was presented in the EU risk assessment report for styrene and was published by Cruzan et al 2009. Isomers of the reactive, side-chain epoxide metabolite of 4-VP (4-VPO), trapped as glutathione (GSH) conjugates, were identified and quantitated. Microsomes of mouse lung were approximately two-fold more active in formation of 4-VPO than rat. 4-VPO formation in rat lung microsomes was also 2-5-fold higher than human. Co-incubation with specific enzyme inhibitors showed that 4-VPO arose primarily via CYP-2F2 oxidation, with lesser amounts arising from the CYP-2E1 isoform. Data on ethylbenzene (EB) metabolism has also been presented in the EU risk assessment report for ethylbenzene and was published by Cruzan et al 2009. The major metabolites arising from incubation with liver or lung microsomes of mouse, rat and human donors were determined. EB was preferentially metabolized to 1-phenylethanol by liver microsomes, with conversion in the order of mouse >> rat ~ human. Both mouse and rat lung microsomes were more active in metabolizing EB than liver microsomes. Similar to liver, the major metabolite of EB was 1-phenylethanol in both mouse and rat lung microsomes, with mouse tissue having higher activity. A subsequent study was then conducted, in which dihydroxylated metabolites of EB were quantitated, as GSH adducts, in microsomal incubations of liver or lung tissue from mouse, rat and human donors. As with styrene, the formation rate of GSH-trapped reactive metabolites in lung microsomes was found to be mouse > rat >> human. Although CYP isoforms responsible for this reactive metabolite formation were not determined, the authors postulate that CYP-2F2 was primarily responsible for ring-hydroxylation of EB and CYP-2E1 for side-chain oxidation. In conclusion, studies have been conducted to show that the major routes of DVB hepatic metabolism are comparable to styrene, both in vivo and in vitro. Based on these similarities in hepatic metabolism, it is expected that the routes of non-hepatic metabolism of DVB would be also consistent with those observed for styrene as well as ethylbenzene. It would also be expected that rates of non-hepatic metabolism of DVB isomers to minor, reactive metabolites, capable of covalent binding to tissue macromolecules, would follow the order of: mouse > rat > human.
Information on Registered Substances comes from registration dossiers which have been assigned a registration number. The assignment of a registration number does however not guarantee that the information in the dossier is correct or that the dossier is compliant with Regulation (EC) No 1907/2006 (the REACH Regulation). This information has not been reviewed or verified by the Agency or any other authority. The content is subject to change without prior notice.
Reproduction or further distribution of this information may be subject to copyright protection. Use of the information without obtaining the permission from the owner(s) of the respective information might violate the rights of the owner.
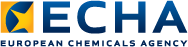