Registration Dossier
Registration Dossier
Data platform availability banner - registered substances factsheets
Please be aware that this old REACH registration data factsheet is no longer maintained; it remains frozen as of 19th May 2023.
The new ECHA CHEM database has been released by ECHA, and it now contains all REACH registration data. There are more details on the transition of ECHA's published data to ECHA CHEM here.
Diss Factsheets
Use of this information is subject to copyright laws and may require the permission of the owner of the information, as described in the ECHA Legal Notice.
EC number: 205-599-4 | CAS number: 143-33-9
- Life Cycle description
- Uses advised against
- Endpoint summary
- Appearance / physical state / colour
- Melting point / freezing point
- Boiling point
- Density
- Particle size distribution (Granulometry)
- Vapour pressure
- Partition coefficient
- Water solubility
- Solubility in organic solvents / fat solubility
- Surface tension
- Flash point
- Auto flammability
- Flammability
- Explosiveness
- Oxidising properties
- Oxidation reduction potential
- Stability in organic solvents and identity of relevant degradation products
- Storage stability and reactivity towards container material
- Stability: thermal, sunlight, metals
- pH
- Dissociation constant
- Viscosity
- Additional physico-chemical information
- Additional physico-chemical properties of nanomaterials
- Nanomaterial agglomeration / aggregation
- Nanomaterial crystalline phase
- Nanomaterial crystallite and grain size
- Nanomaterial aspect ratio / shape
- Nanomaterial specific surface area
- Nanomaterial Zeta potential
- Nanomaterial surface chemistry
- Nanomaterial dustiness
- Nanomaterial porosity
- Nanomaterial pour density
- Nanomaterial photocatalytic activity
- Nanomaterial radical formation potential
- Nanomaterial catalytic activity
- Endpoint summary
- Stability
- Biodegradation
- Bioaccumulation
- Transport and distribution
- Environmental data
- Additional information on environmental fate and behaviour
- Ecotoxicological Summary
- Aquatic toxicity
- Endpoint summary
- Short-term toxicity to fish
- Long-term toxicity to fish
- Short-term toxicity to aquatic invertebrates
- Long-term toxicity to aquatic invertebrates
- Toxicity to aquatic algae and cyanobacteria
- Toxicity to aquatic plants other than algae
- Toxicity to microorganisms
- Endocrine disrupter testing in aquatic vertebrates – in vivo
- Toxicity to other aquatic organisms
- Sediment toxicity
- Terrestrial toxicity
- Biological effects monitoring
- Biotransformation and kinetics
- Additional ecotoxological information
- Toxicological Summary
- Toxicokinetics, metabolism and distribution
- Acute Toxicity
- Irritation / corrosion
- Sensitisation
- Repeated dose toxicity
- Genetic toxicity
- Carcinogenicity
- Toxicity to reproduction
- Specific investigations
- Exposure related observations in humans
- Toxic effects on livestock and pets
- Additional toxicological data

Toxicological Summary
- Administrative data
- Workers - Hazard via inhalation route
- Workers - Hazard via dermal route
- Workers - Hazard for the eyes
- Additional information - workers
- General Population - Hazard via inhalation route
- General Population - Hazard via dermal route
- General Population - Hazard via oral route
- General Population - Hazard for the eyes
- Additional information - General Population
Administrative data
Workers - Hazard via inhalation route
Systemic effects
Long term exposure
- Hazard assessment conclusion:
- DNEL (Derived No Effect Level)
- Value:
- 0.72 mg/m³
- Most sensitive endpoint:
- repeated dose toxicity
DNEL related information
- Overall assessment factor (AF):
- 5.31
- Modified dose descriptor starting point:
- NOAEC
Acute/short term exposure
- Hazard assessment conclusion:
- DNEL (Derived No Effect Level)
- Value:
- 9.4 mg/m³
- Most sensitive endpoint:
- acute toxicity
DNEL related information
- Overall assessment factor (AF):
- 0.53
- Modified dose descriptor starting point:
- NOAEC
Local effects
Long term exposure
- Hazard assessment conclusion:
- no data available: testing technically not feasible
- Most sensitive endpoint:
- repeated dose toxicity
Acute/short term exposure
- Hazard assessment conclusion:
- no data available: testing technically not feasible
DNEL related information
Workers - Hazard via dermal route
Systemic effects
Long term exposure
- Hazard assessment conclusion:
- DNEL (Derived No Effect Level)
- Value:
- 0.102 mg/kg bw/day
- Most sensitive endpoint:
- repeated dose toxicity
DNEL related information
- Overall assessment factor (AF):
- 5.31
- Modified dose descriptor starting point:
- NOAEL
Acute/short term exposure
- Hazard assessment conclusion:
- DNEL (Derived No Effect Level)
- Value:
- 3.03 mg/kg bw/day
- Most sensitive endpoint:
- acute toxicity
DNEL related information
- Overall assessment factor (AF):
- 33.09
- Modified dose descriptor starting point:
- LOAEL
Local effects
Long term exposure
- Hazard assessment conclusion:
- no data available: testing technically not feasible
- Most sensitive endpoint:
- repeated dose toxicity
Acute/short term exposure
- Hazard assessment conclusion:
- no data available: testing technically not feasible
Workers - Hazard for the eyes
Local effects
- Hazard assessment conclusion:
- high hazard (no threshold derived)
Additional information - workers
- For exposures to powders which are pH controlled, the exposure estimate from the TRA tool will be employed and compared to the DNEL for the applicable cyanide saltfor inhalation and dermal routes.
- For exposures to solid material in granular or brick form the exposure will be adjusted to presume 20% generation rate (w/w) of inhalable dust, on the basis of Stoffenmanager estimates. As above, the exposure will be compared to the DNEL for NaCN recognizing a 100% absorption and hydration rate and subsequent conversion to HCN in the body. The remaining 80% of estimated material is unavailable for exposure.
- For exposures to substance in solution, which is pH controlled, the exposure estimate from the TRA tool will be employed and compared to the DNEL for the applicable cyanide salt
- For exposures to any material, in solution, which is not pH controlled (pH less than 11 or intentially dropped to low pH levels of approximately 3), the exposure will be adjusted to presume conversion to HCN on a molar basis and compared to the HCN DNEL 5. For acute dermal exposures to NaCN, the more conservative value for chronic dermal exposures is used as per ECHA guidance (May 2010).
Cyanide salts can rapidly undergo a transformation to hydrogen cyanide (HCN) vapour when hydrated without pH control. As the pKa for HCN is 9.36, maintenance of the pH over a value of 11 insures that the cyanide remains in an ionic form, which is less toxic. At low pH, HCN predominates and is easily absorbed via dermal, inhalation and oral contact. Under most physiological and environmental conditions at pH 7-8, HCN is the predominant species.
In the workplace, strict attention is paid to controlling the pH of solutions of cyanide salts, and to minimizing the generation of HCN vapour. There are some exposure scenarios where vapour could theoretically be formed from solid cyanide salts. In these cases, molar weight adjustments are made for HCN, and the DNEL for hydrogen cyanide is used. This value is based on CN- anion concentration as presented in the sections of this document relating to chronic inhalation toxicity.
Part A: Acute systemic DNELs
According to Technical Guidance Document R.8, Guidance on information requirements and chemical safety assessment, May 2008, Appendix 8, Figure 8.5, p.111, acute DNELs for acute toxicity should be derived if an acute toxicity hazard has been identified and there is a potential for high peak exposures. High peak exposures are usually assessed for the inhalation route. ECHA recommends that acute DNELs should be derived from human data, if this data is available. This data consists of reports of poisonings, epidemiology, and case studies. Time-scaling is to be applied if applicable for inhalation exposures, based on Haber’s Law of Concentration x time), and an assessment factor (AF) for intraspecies (individual) variation is to be applied.
The draft Guidance on Derivation of DNEL/DMEL from Human Data, February 2010, Rev:1.1, further advises that, “for many chemicals, both human data and animal data are available….Therefore, an integrated approach is required” (p. 5). Figure R 8-x, Illustration of the process for DNEL/DMEL derivation from human data (p. 7), states that the process involves deriving DNELs/DMELs of Animal Data (AD) according to Chapter R.8, and obtaining the DNELs/DMELs from Human Data (HD), and then integrating the HD and AD and selecting the critical DNEL/DMEL.
The original guidance states that, if a valid science-based Occupational Exposure Level (OEL) or Short Term Exposure Limit (STEL) is available, this should be utilized as a DNEL. (Guidance R.8, Appendix 13, p. 142.)
1. Inhalation: Human Data
The short term OEL is used to derive the inhalation DNEL for the worker. The U.S. EPA (2009) has proposed the Acute Exposure Guideline Level (AEGL) for potassium cyanide, for 10 min, to be 6.6 mg/m3. The proposed 2009 SCOEL STEL for 15 minutes is 5 mg/m3for the CN- from HCN, KCN and NaCN.
Beginning with the proposed SCOEL STEL for the powder, and applying molecular weight corrections (2.50 for KCN and 1.89 for NaCN), yields the following:
KCN: 5.0 mg CN-/ m3x (65.12 KCN /26.02 CN-) = 12.5 mg/m3.
NaCN: 5.0 mg CN-/ m3x (49.01 NaCN /26.02CN-) = 9.42 mg/m3.
HCN: 5.0 mg CN-/ m3 = 5.0 mg/m3as per the SCOEL recommendations.
2. Worker Inhalation: Animal Data
The Task Force on Cyanides, authors of the ECETOC JACC No. 53 (2007), explored in detail the relation between inhaled dose (concentration over time, C ´ t) and mortality, with the objective to relate acute animal inhalation toxicity data to hazard in humans. Three key animal studies provided critical information. The basic design of these studies was quite similar, groups of 2 to 10 animals were exposed to a range of concentrations and exposure periods. During an exposure period of varying duration, the atmospheric concentration was kept broadly constant. TheTask Forcethen addressed the relative sensitivity of animals and humans with a view to deriving C ´ t dependent estimates of LC50and LC01values for humans. Barcroft (1931), though dated, is the only paper providing directly comparable information on species sensitivity.
Barcroft (1931) exposed goats, monkeys, rabbits, rats, cats, mice and dogs to HCN vapour in a large closed chamber and studied survival at different concentrations and exposure periods. Exposure levels were not measured, but otherwise the paper contains sufficient details of study design and equipment. The exposure chamber was described as a closed gas chamber with a volume of 10 m3and equipped with an electric fan stirring the air. An operator, provided with a respirator, went inside (through an air lock of about 2 m3) with a calibrated amount of HCN in a glass tube and started the experiment by breaking the glass tube. The HCN evaporated immediately and the operator enhanced mixing of the air by waving a large piece of cardboard. The HCN concentration appeared to be decreased by 22% after 38 minutes. The period of observation of the animals after exposure was not mentioned.
Lapin and Mackay (1981) exposed rats in a chamber (volume 175 liters) that was continuously flowed through with HCN from a gas bottle, diluted with air. The front panel of the chamber was provided with exposure ports, in which animal holding tubes for nose-only exposure were fitted. The chamber atmosphere was measured continuously by infra-red spectrometry and validated by GC. Rats were exposed free-moving or under restraint (nose-only). Rats generally died during exposure. Survivors were followed for 7 days and body-weight gain was recorded.
Ballantyne (1994) generated different HCN vapour concentrations by varying the ratio of mixing solutions of NaCN and HCl and evaporating the HCN by means of an air stream. Exposure concentrations were monitored by aspirating chamber air through bubblers (5 ml NaOH solution) at a flow rate of 1 liter/min and determining cyanide in the alkaline washing liquid using a calibrated cyanide-specific electrode. The period of observation of the animals after exposure was not indicated. For deriving the concentration-time relationship for 50% mortality response, only those trials with an exposure period of more than one minute were used.
In order to check the quality of the data of Barcroft (1931) the concentration-time mortality response relationship for rats was derived. The relationship of Barcroft was compared with the relationship derived from the extensive data sets of Lapin and Mackay (1981) and of Ballantyne (1994) for rats. This analysis showed that there was no significant difference between the dose-mortality response of the free moving rats in all three studies; the data and conclusions from Barcroft are thus validated.
The restrained rats (Lapin and Mackay, 1981) appeared to be more sensitive than free moving rats. This may be explained by stress effects exacerbating the direct chemical effects of cyanide.
Derived concentration-time mortality response relationships for the 7 species studied by Barcroft (1931) are presented in the table below. Goats and monkeys appeared to be least sensitive and mice, cats and dogs most sensitive to inhaled HCN.
The derived data illustrate a trend of larger species showing lower sensitivity to inhaled cyanide than smaller species. However, dogs and cats do not fit into this trend well. The Task Force estimated the detoxifying capacity per kg body weight per minute from the LC50at 65 minutes and the LC50at 5 minutes exposure. The difference in absorbed amount of HCN between 65 and 5 minutes was assumed to be detoxified and was compared with the respiratory volume per kg body weight and hour for each species [data taken from Snipes (1989) except for goats where respiratory volume was calculated from the average tidal volume of 7.43 l/min reported by Santiago and Edelman (1976) and an assumed body weight of 30 kg for an adult goat]. The comparison shows that the derived detoxification rates are quite similar in different species with rates between 0.015 (cat) and 0.029 mg CN‾/kgbw/min (rat).
Regression coefficients for free moving animals (Barcroft, 1931)and estimates ofrespiratory volume and detoxifying capacity
Species |
Regression coefficient |
LC50(mg/m3) |
Respiratory |
Detoxifying rate |
|||
b |
N |
a |
5 min |
65 min |
|||
Goat |
2.024 |
2.22 |
27.3 |
638 |
201 |
15 |
0.021 |
Monkey |
0.835 |
1.88 |
6.87 |
829 |
211 |
17 |
0.023 |
Rabbit |
0.744 |
4.33 |
15.6 |
411 |
227 |
16 |
0.028 |
Rat |
0.701 |
1.64 |
3.27 |
496 |
104 |
48 |
0.029 |
Cat |
0.741 |
2.81 |
8.26 |
325 |
131 |
16 |
0.015 |
Dog |
0.327 |
3.13 |
1.3 |
283 |
125 |
22 |
0.021 |
The detoxifying rate is dependent on the level of rhodanese enzyme in liver and muscles. Aminlari and Gilanpour (1991) reported that dog tissues and especially liver contained much less rhodanese activity than the same tissues of camel, horse, donkey, cattle and sheep, with brain tissue being the only exception. As a carnivorous species, the dog is not usually exposed to cyanogenic plants, hence there is little rhodanese activity in liver. The same applies to the cat. Using the same logic, it is to be expected that man is not as sensitive as the dog because man is omnivorous. The difference in sensitivity between dogs and humans was previously reported by Barcroft (1931), who exposed himself to HCN while being accompanied by a dog. The dog lost consciousness within in a few minutes, but Barcroft remained conscious and left the room without any assistance one minute after the dog had lost consciousness.
On the basis of this information it seems reasonable, as Barcroft did in his 1931 paper, to consider the goat and the monkey as one group of animals representative for humans. The following LC50 and LC01values, estimated from the combined goat and monkey data of Barcroft (1931), are therefore considered to be representative for man.
Estimated LC values for different exposure duration(ten Berge, 2003a, 2006)
Duration (min) |
LC50(mg/m3) |
LC01(mg/m3) |
5 |
676 (536 ‑ 833) |
296 (106 ‑ 415) |
10 |
483 (411 ‑ 569) |
211 (80 ‑ 288) |
15 |
396 (345 ‑ 465) |
173 (67 ‑ 234) |
20 |
345 (300 ‑ 408) |
151 (60 ‑ 202) |
25 |
309 (267 ‑ 372) |
135 (54 ‑ 181) |
30 |
283 (243 ‑ 346) |
124 (50 ‑ 166) |
60 |
202 (164 ‑ 267) |
88 (36 ‑ 120) |
120 |
144 (108 - 214) |
63 (26 - 88) |
240 |
103 (71 - 170) |
45 (18 - 66) |
480 |
73 (47 - 136) |
32 (13 - 50) |
The LC50estimates fit well with reported accidental and intentional exposure. Bonsall, (1984) reported on a worker who survived a 6 minute exposure in a 3500 gal tank containing a HCN vapour concentration of approximately 500 mg/m3.
The above data demonstrates that observed LD50 values from animal studies can be scaled for human exposure. From these, a LD01 (lethal concentration in 1% of the population) value can be estimated which is consistent with OEL level at 15 minutes. The LC01 can roughly be considered a Lowest Observable Effect Concentration (LOEC) for humans. A 15 minute exposure associated with the LC01 is 173 (67-234) mg CN-/ m3, from the above table.
NOEC [CN-] mg/kg = LC01 (mg/m3)
AFLOAEC× AFintraspecies
LC01 = 173 mg/ m3
AF (LOAEC to NOEC) (AF) = 2
AFintraspecies= 10
Dividing the LC01 value of 173 mg/ m3in half (LOAEC to NOEC) yields 86.5 mg/ m3, and by applying a 10-fold intraspecies assessment factor, a DNEL value of 8.7 mg CN-/ m3is estimated. This supports the proposed SCOEL occupational STEL of 5.0 mg/ m3.
3. Worker Dermal:
Rieders, 1971, reported that the human dermal LD50 of a cyanide salt was 100 mg/kg bw; the NOAEL would be lower. Ballantyne (1987) found that contact of NaCN on intact dry skin of rabbits did not result in the absorption of compound in amounts sufficient to product any signs of systemic toxicity. The LD50 for dry powdered NaCN in intact skin (200 mg/kg bw) is about 13-fold higher than that of NaCN solutions. In open abraded skin, NaCN is absorbed at levels equivalent to that of a NaCN solution, where the LD50 is 11-14 mg/kg bw. According to Dugard (1987), the amount and speed of absorption through human skin is dependent on the size of the contaminated skin area, the duration of skin contact, the concentration and the pH of the cyanide salt solution (a low pH resulting in rapid absorption of HCN, a high pH resulting in slower absorption of the ionized species). He reported that the ionized cyanide salts show a delay of over 90 minutes before absorption takes place.
A more theoretical DNEL is derived from the 100 mg/kg bw dermal poisoning value (LD50) of HCN as reported by Reeders (1971).
Two assessment factors are applied to modify the dose descriptor: AFLD50 to LOAEL= 3 and AFLOAEL to NOAEL= 2
NOAEL: 100 mg/kg= 16.7 mg HCN/kg bw.
3 x 2
The modified dose descriptor is divided by an intraspecies AF of 10.
16.7 mg HCN/kg bw = 1.67 mg HCN/kg bw.
10
For CN-,DNEL of 1.67 mg/kg bw *(26.02/27.03) = 1.61 mg/kg bw.
For KCN, DNEL of 1.61 mg/kg bw *(65.12/26.02) = 4.03 mg/kg bw.
For NaCN, DNEL of 1.61 mg/kg bw *(49.01/26.02) = 3.03 mg/kg bw.
These DNELs are derived as directed by the Regulation. However, Technical Guidance Document R.14 suggests that, to be precautionary, the chronic dermal DNEL should be used for risk assessment (p. 19, version 2.0, May 2010). Thus, the value of 0.10 mg/kg bw is used to generate risk characterisation ratios.
The cyanide industry has long demanded and enforced risk management measures which prevent dermal exposure of cyanide salts by workers. These include impermeable gloves, boots, protective clothing, face/eye shields. Workers are trained to seek medical attention immediately should a dermal exposure occur accidently. Thus, even though DNELs were derived, these should be considered as more theoretically as acute dermal exposure to cyanide salts is highly unlikely due to the extensive range of risk management measures implemented and strictly followed.
4. Worker Oral:
Workers are not exposed to cyanide salts via the oral route by voluntary ingestion of the substance.
It is possible that a low amount of oral ingestion could occur via mucociliary action of particulate material which enters the nasal passages and is subsequently swallowed. This represents a fraction of the material which would be absorbed in the moist respiratory tract, converted to hydrogen cyanide, and absorbed via the bloodstream into the systemic circulation, where it would exert its effects on inhibition of cellular respiration. The effect of gastric absorption is minimal compared to inhalatory absorption.
It is significantly more likely that workers would be exposed to cyanide by ingesting food which contains cyanogenic glycosides (cassava, lima beans, etc.) However, this risk assessment is outside the scope of REACH (Regulation (EC) No. 1907/2006).
Acute DNELs for oral exposure of workers are not derived.
5. General Population
Commercial cyanide is not supplied to the general population and there are no recognized downstream uses which incorporate cyanide in a form where it is released to the public. As discussed earlier, the more likely exposure to the general public occurs through voluntary cigarette smoking or by ingesting foodstuffs containing natural cyanide derivatives, applications where risk assessment is outside the scope of REACH.
Acute DNELs for the general population are not derived.
Part B: Chronic DNELs:
The leading health effect of repeat dose exposure to cyanides is development of goitre. This chronic toxicity of HCN was reviewed by ECETOC in the JACC report on Cyanides (No. 53, 2007) which concluded that an acceptable chronic level of exposure to CN- could be established using levels of cyanide and its metabolite, thiocyanate (SCN).
Barker, 1936, and Barker,et al(1941) treated human patients suffering from hypertension with potassium thiocyanate, ranging from 0.3 to 1 g/patient, daily. Serum concentrations of SCN- were found to range from 345 to 690 μmol/l (20 - 40 μg/ml). This can be selected as a human NOAEL for thiocyanate therapy. Cliffet al(1986) found that levels of 250 μmol SCN-/l serum (14.5 μg SCN-/ml) did not lead to an increased incidence of goitre in a cassava eating population that was not iodine deficient; iodine deficiency exacerbated thyroid toxicity.
Similar findings were reported by Banerjee (1997) for electroplating workers in which serum concentrations of 316 μmol/l (18 μg SCN-/ml) did not lead to goitre. Both of the latter populations had slight changes in thyroid hormone levels that can be considered as an adaptive response.
The ECETOC review recognised that due to background variations in co-exposure to cyanogenic sources (e.g., environmental (combustion), dietary (cyanogenic foods) and habitual (i.e. smoking)) and levels of dietary iodine, it is not possible to distinguish precisely the effects of exogenous CN- on thyroid hormone levels, the critical effect. For this reason, ECETOC selected the threshold for clinical diagnosis between adaptive and goitrogenic changes as a NOAEL rather than a NOEL.
The ECETOC Task Force further proposed that a level of 15 μg SCN-/ml can be used as a starting point to derive a safe concentration of cyanide for humans, using the following equation.
[CN-] mg/m3= [SCN-] mg/l × 0.25 l/kg bw × 70 kg bw × 26
10 m3 × 0.8 × 3.9 × 58
Where
15 = [SCN-] mg/l
0.25 l/kg bw = distribution volume of SCN
70 kg = body weight
26 = molecular weight CN
10 m3= respiratory volume during an 8-hour day
0.8 = conversion of cyanide to thiocyanate: ~ 80%
3.9 = elimination of thiocyanate, half-life = 2.7 d, residence time (t0.5/ln 2) = 3.9 d
58 = molecular weight SCN-
The derived safe inhalation concentration of cyanide anion for humans exposed for 8 hours at the workplace of 3.75 mg/m3can be used as a derived dose descriptor for the calculation of a DNEL for the worker..
Assessment Factors:
In considering the overall level of uncertainty, typically the following aspects should be considered:
· interspecies differences;
· intraspecies differences;
· differences in duration of exposure;
· issues related to dose-response; and,
· quality of available human database including: completeness, consistency, reliability, healthy worker effect, study size.
Interspecies differences: Relevant when deriving a DNEL from animal studies. The value of this parameter is 1 when deriving the DNEL from human data.
Intraspecies differences: As recognized previously, sensitive sub-populations would include individuals with insufficient dietary iodine, insufficient thiosulphate supply (e.g., in the case of malnutrition) or impaired renal function. Whereas the human studies relied upon for development of the critical value typically included several hundred to several thousand individuals from as young as 5 to 80 years old, they were likely representative of the general population. As such, it is acknowledged that an intraspecies assessment factor may be viewed as necessary. Typically, a factor no greater than 10 is used in this regard. It is recognized that there is residual uncertainty regarding developmental effects due to iodine insufficiency and its impact on thyroid hormone levels and this is addressed under completeness of the database (below).
Differences in duration of exposure: In terms of differences in duration of exposure, the clinical studies by Barker et al. (1941) included 45 and 246 patients that received thiocyanate therapy for 1 to 4, or 4 to 10 years. The study in a cassava-eating population by Cliff et al., (1986) included 276 individuals (subset of 27 for some measurements) between 5 and 60 years old that had lived on this staple diet all their life (control group aged 19 – 85). The other supporting studies of Barrère et al. (2000, 2002) included 2987 subjects aged between 36 and 60 years; Bourdoux et al. (1978) included 66 individuals aged 9 to 16 yrs, 386 aged 0 to 80 yrs and 988 aged 15 to 30 yrs; Jackson (1988) studied 73 individuals aged 15 to 80 yrs; Knudsen et al., (2000, 2002) involved 2656 individuals aged from 41 to 71 years and Dahlberg et al., 1984 included 37 individual 16 to 54 yrs. From the multitude of studies, it should be readily apparent that the duration of exposure in the human population studies was sufficient to address any latency of the critical effect.
Issues related to dose-response: The dose response of the critical effect (goitre) is relatively well known in terms that it comprises a continuum from the true NOEL through the NOAEL for goitre development. The determined NOAEL is established principally by the studies of Knudsen et al. (2000) and the much larger study of Barrère et al. (2000) supported by Knudsen et al. (2002) and Cliff et al. (1986). The size of the cohorts used for the determination, particularly that of Barrère et al. (2987 subjects aged between 36 and 60 years), suggests a high confidence in the assessment and no need to apply any additional factor to account for the dose-response of the critical effect.
Quality of available human database (and animals) including:
a) In terms of Completeness, the combined database on the health effects of cyanides is one of the most complete known. In addition to the well established mechanism of action for both acute and chronic toxicity, there are three OECD guideline sub-chronic studies, that include more than one species, by different routes as well as extensive clinical experience and large epidemiology studies from more than one continent. Furthermore, the acute toxicity of cyanide, together with its steep dose response curve and proximity between onset of repeat-dose effects and acute lethality, makes the conduct of chronic studies in animals technically very challenging, if not impossible to conduct. Taking the weight-of-evidence approach, the database for developing the dose descriptor is sufficiently complete.
b) In terms of Consistency, the NOAELs from the 90 day studies were broadly consistent; serum thiocyanate levels in the studies of Leuschner et al. (1991) and Monsanto (1985) in rats and Tewe and Maner (1980) in pigs were very similar to the human NOAEL for thiocyanate therapy and the level that did not lead to an increased incidence of goitre in a cassava-eating population that was not iodine deficient (Cliff et al., 1986) as well as electroplating workers without goitre (Banerjee, 1997). As there is a high level of consistency between the various animal and human studies, no additional factor to account for consistency is necessary.
c) In terms of Reliability, several animal studies are full OECD guideline studies of high reliability. The clinical data were sufficient to support use of thiocyanate as a therapeutic agent for over a decade and the epidemiology studies well conducted by multiple independent investigators using recognized approaches and published in peer reviewed journals. As such, the data are considered reliable and no additional factor to account for reliability is necessary.
d) In terms of Healthy worker effect, the only study relied on for the dose descriptor that could be subject to the healthy worker effect is that of Banerjee (1997) on electroplaters. As the other studies were conducted on individuals drawn from the public and many were outside the age range typically identified as representing the working age, this is not considered relevant. Hence, no additional factor to account for the healthy worker effect is necessary.
e) In terms of Study size, the human studies vary from several hundred to several thousand individuals. Collectively they represent a sufficiently large study size. Hence, no additional factor to account for study size is necessary.
In conclusion, there is an overall high confidence in the database and there is no need to apply additional uncertainty factors over and above an intraspecies uncertainty factor of 10 for the worker.
1. Inhalation-worker: Human data
By applying a combined AF of 10 (10 to account for intraspecies differences in sensitivity (dietary iodide and renal function) and 1 for interspecies uncertainty (as mainly derived from human data) to the derived dose descriptor of 3. 75 mg/m3, a DNELinh-worker– inhalation of 0.38 mg CN-/ m3 is therefore derived. This value is used for the occupational scenario where inhalation of dry powder may occur (not considering the mandatory use of respirators). In this case, 100% of the powdered substance is inhaled into and potentially retained by the nasal cavity and/or respiratory tract, and the CN- moiety can be converted to HCN in the body. Accounting for the molecular weight of NaCN, this value is 0.72 mg/m3.
There is an alternate scenario where HCN may be generated from granules, bricks or solutions of cyanide salts. In this case, HCN is theoretically generated external to the body, and is available to be respired in the lung as vapour. The ECETOC Task Force indicated the need to apply a correction factor to account for a 50% efficiency of absorption by the lung (JACC Report #53, p.353). Consequently, the dose descriptor of 3.75 mg/m3becomes 7.50 mg/m3.
2. Inhalation-worker: Animal data
The 90-day inhalation toxicity study reviewed in ECETOC, 2007 (acetone cyanohydrin ) is informative for extrapolation of a DNEL from animal studies. The NOAEC in this study (6 h per day, 5 days per week) was 66 mg CN-/m3(216 mg ACH/m3). Considering the 8 hour workday and a higher respiration volume (10 m3during light activity vs 6.7 m3during rest) for workers, the dose descriptor is 33.17 mg/ m3.
NOAEC =66 mg/ m3* 6 h* 6.7 m3 = 33.17 mg/ m3.
8 h * 10 m3
Assessment factors include:
1. interspecies= 10
2. intraspecies= 10
3. subchronic-chronic= 2
4. dose-response= 1
5. database completeness= 1
6.
DNELinh= 33.17 mg CN-/m3 = 0.17 mg/m3
200
This compares favorably with the DNELinh-workerderived from human SCN exposure, 0.38 mg CN-/m3.
3.
DNELdermal-workercan also be derived from acceptable thiocyanate levels:
[CN-] mg/kg= [SCN-] mg/l × 0.25 l/kg bw × 26
0.8 × 3.9 × 58
Where
15 = [SCN-] mg/l
0.25 l/kg bw = distribution volume of SCN
26 = molecular weight CN
0.8 = conversion of cyanide to thiocyanate: 80%
3.9 = elimination of thiocyanate, half-life = 2.7 d, residence time (t0.5/ln 2) = 3.9 d
58 = molecular weight SCN-
This equation results in a value of 0.54 mg/kg bw/d, and is the derived safe dermal concentration of cyanide for humans exposed for 8 hours at the workplace. By applying an AF of 10 (10 to account for intraspecies differences in sensitivity (dietary iodide and renal function) and 1 for interspecies uncertainty (as mainly derived from human data)) to the derived dose descriptor of 0.54 mg CN-/kg bw, the DNELdermal-workerof 0.054 mg/kg bw/d is obtained. Accounting for the molecular weight of NaCN, this value is 0.102 mg/kg bw/d.
4. Dermal-worker: Animal data
There are several key oral subchronic studies undertaken in rats which provide useful information in the derivation of a DNEL from animal studies. The guideline study by Hebert (1993), in the US National Toxicology Program, investigated 90-day administration of NaCN in the drinking water of F344 rats. The NOAEL in this study, as reviewed by the ECETOC Task Force on Cyanides, was the high dose of 12.5 mg CN-/kg bw/d in rats, and 25.6 mg CN-/kg bw/d in mice. While there has been some question raised about reproductive effects in male rats and mice in this study concerning organ weight effects of the cauda epididymis and the sperm motility effects, these are not considered biologically significant, reproducible or associated in a causal relationship to cyanide exposure. These effects are more likely due to restricted water intake and stress.
A second important study by Leuschner (1989) which has recently become available was a 90-day experiment in Sprague-Dawley rats with KCN administered in the drinking water at concentrations 10-fold higher than those in the Hebert study. As cyanide salts in the drinking water result in decreased water consumption by rodents, presumably due to unpalatable taste, the study included an extra control group which was given the same amount of water which was consumed by the high-dose cyanide group. Findings which occurred in the cyanide-exposed group were also frequently observed in this drinking-water-controlled group; these included organ weight changes and clinical chemistry effects. No reproductive organ changes were observed which were attributable to cyanides. The NOAEL in this study was 40 mg KCN/kg bw/d or 16 mg CN-/kg bw/d.
Tewe and Maner (1984) exposed rats for 56 days to KCN in the diet at doses of 40 mg CN-/kg bw/d (100 mg KCN/kg bw/d.) The rats tolerated this dosage of cyanide without signs of toxicity.
The ECETOC Task Force established a consensus NOAEL of the repeat-dose oral subchronic toxicity studies in rats, without the Leuschner (1989) study, of 12.5 mg CN-/kg bw/d. Including the Leuschner (1989) study suggests that a higher NOAEL may be established.
Assessment factors include:
1. interspecies= 10
2. intraspecies= 10
3. subchronic-chronic= 2
4. dose-response= 1
5. database completeness= 1
6.
DNELdermal-worker= 12.5 mg CN-/m3 = 0.062 mg/m3
200
This compares favorably with the DNELdermal-workerderived from human SCN exposure, 0.056 mg CN-/m3.
Part C: Qualitative Assessment for Skin and Eye Irritation and Skin Sensitisation:
Local, irritation and sensitization effects of cyanides are less relevant compared to the systemic effects (which can include lethality) after dermal absorption of the compounds. The undertaking of OECD guideline studies using animal models is inappropriate as the doses required result in lethality of the animals. While some authors have reported anecdotal human skin and eye irritation (Rieders, 1987, Ballantyne, 1987), this is likely complicated by effects of the vehicle. Liquid cyanide salts are distributed as alkaline solutions in water at a pH of approximately 11, and the caustic properties of these may contribute to the occurrence of irritation and dermal/ocular burns. A qualitative risk assessment is therefore appropriate for the endpoints of dermal irritation and sensitization.
Furthermore, risk management measures include a requirement for gloves and other protective clothing such as rubber boots and impervious apron/clothing and face masks. These measures are equivalent to those required if cyanides were considered irritants, and are sufficient to protect workers from any potential risk of irritancy. If exposure inadvertently occurs, it is recommended that any exposed skin be washed immediately and that medical attention be sought as soon as possible.
Notes on application of DNELs to Exposure Scenarios:
General Population - Hazard via inhalation route
Systemic effects
Long term exposure
- Hazard assessment conclusion:
- exposure based waiving
Acute/short term exposure
- Hazard assessment conclusion:
- exposure based waiving
DNEL related information
Local effects
Long term exposure
- Hazard assessment conclusion:
- exposure based waiving
Acute/short term exposure
- Hazard assessment conclusion:
- exposure based waiving
DNEL related information
General Population - Hazard via dermal route
Systemic effects
Long term exposure
- Hazard assessment conclusion:
- exposure based waiving
Acute/short term exposure
- Hazard assessment conclusion:
- exposure based waiving
DNEL related information
Local effects
Long term exposure
- Hazard assessment conclusion:
- exposure based waiving
Acute/short term exposure
- Hazard assessment conclusion:
- exposure based waiving
General Population - Hazard via oral route
Systemic effects
Long term exposure
- Hazard assessment conclusion:
- exposure based waiving
Acute/short term exposure
- Hazard assessment conclusion:
- exposure based waiving
DNEL related information
General Population - Hazard for the eyes
Local effects
- Hazard assessment conclusion:
- high hazard (no threshold derived)
Additional information - General Population
This substance is not placed on the consumer market in Europe, and the manufacturer of this substance is unaware of downstream applications which would release the substance in detectable amounts to the consumer. Thus, the likelihood of exposure by the general public to the registered substance from a commercial source is negligible. It is significantly more likely that persons would be exposed to cyanide compounds by ingesting food which contains cyanogenic glycosides (cassava, lima beans, etc.) or by electively smoking cigarettes. This risk assessment is outside the scope of REACH.
Cyanide salts are not bioaccumulative in the food chain , and hence there is no need to consider intake by the general population in meat and milk, or in other foodstuffs. This can be considered qualitatively in the risk assessment.
It is possible that cyanide released from industrial sites into the environment may make its way into the water supply and be could available for consumption by the general public. However, the Drinking Water Directive (Directive 98/83/EC) has addressed this issue, setting a limit of 0.05 mg/L. Further evaluation in this matter is outside of the scope of REACH.
Information on Registered Substances comes from registration dossiers which have been assigned a registration number. The assignment of a registration number does however not guarantee that the information in the dossier is correct or that the dossier is compliant with Regulation (EC) No 1907/2006 (the REACH Regulation). This information has not been reviewed or verified by the Agency or any other authority. The content is subject to change without prior notice.
Reproduction or further distribution of this information may be subject to copyright protection. Use of the information without obtaining the permission from the owner(s) of the respective information might violate the rights of the owner.
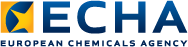