Registration Dossier
Registration Dossier
Data platform availability banner - registered substances factsheets
Please be aware that this old REACH registration data factsheet is no longer maintained; it remains frozen as of 19th May 2023.
The new ECHA CHEM database has been released by ECHA, and it now contains all REACH registration data. There are more details on the transition of ECHA's published data to ECHA CHEM here.
Diss Factsheets
Use of this information is subject to copyright laws and may require the permission of the owner of the information, as described in the ECHA Legal Notice.
EC number: 202-476-7 | CAS number: 96-09-3
- Life Cycle description
- Uses advised against
- Endpoint summary
- Appearance / physical state / colour
- Melting point / freezing point
- Boiling point
- Density
- Particle size distribution (Granulometry)
- Vapour pressure
- Partition coefficient
- Water solubility
- Solubility in organic solvents / fat solubility
- Surface tension
- Flash point
- Auto flammability
- Flammability
- Explosiveness
- Oxidising properties
- Oxidation reduction potential
- Stability in organic solvents and identity of relevant degradation products
- Storage stability and reactivity towards container material
- Stability: thermal, sunlight, metals
- pH
- Dissociation constant
- Viscosity
- Additional physico-chemical information
- Additional physico-chemical properties of nanomaterials
- Nanomaterial agglomeration / aggregation
- Nanomaterial crystalline phase
- Nanomaterial crystallite and grain size
- Nanomaterial aspect ratio / shape
- Nanomaterial specific surface area
- Nanomaterial Zeta potential
- Nanomaterial surface chemistry
- Nanomaterial dustiness
- Nanomaterial porosity
- Nanomaterial pour density
- Nanomaterial photocatalytic activity
- Nanomaterial radical formation potential
- Nanomaterial catalytic activity
- Endpoint summary
- Stability
- Biodegradation
- Bioaccumulation
- Transport and distribution
- Environmental data
- Additional information on environmental fate and behaviour
- Ecotoxicological Summary
- Aquatic toxicity
- Endpoint summary
- Short-term toxicity to fish
- Long-term toxicity to fish
- Short-term toxicity to aquatic invertebrates
- Long-term toxicity to aquatic invertebrates
- Toxicity to aquatic algae and cyanobacteria
- Toxicity to aquatic plants other than algae
- Toxicity to microorganisms
- Endocrine disrupter testing in aquatic vertebrates – in vivo
- Toxicity to other aquatic organisms
- Sediment toxicity
- Terrestrial toxicity
- Biological effects monitoring
- Biotransformation and kinetics
- Additional ecotoxological information
- Toxicological Summary
- Toxicokinetics, metabolism and distribution
- Acute Toxicity
- Irritation / corrosion
- Sensitisation
- Repeated dose toxicity
- Genetic toxicity
- Carcinogenicity
- Toxicity to reproduction
- Specific investigations
- Exposure related observations in humans
- Toxic effects on livestock and pets
- Additional toxicological data

Endpoint summary
Administrative data
Link to relevant study record(s)
Description of key information
Key value for chemical safety assessment
Additional information
Absorption and pharmacokinetics of SO
The absorption of SO has not been extensively studied. There is evidence of its absorption through the respiratory tract. Urine from workers exposed to SO vapors contained large amounts of mandelic acid and phenylglyoxylic acid, both metabolites of SO (Hulz et al.1967, Ohtsuji and Ikeda 1970, cited in IARC 1976). SO also is absorbed slowly through the skin (Hine and Rowe 1963, cited in IARC 1976).Rappaport et al.(1996) reported the presence of SO-specific biomarkers, [aandb isomers of N2-(2-hydroxy-1-phenylethyl)-2’-deoxyguanosine-3'-phosphate DNA adducts and cysteine albumin adducts] in the blood of plastics workers exposed to both styrene per se and SO.Marczynski et al.(1997) studied the high molecular weight DNA fragmentation in white blood cells following SO incubation with human blood. The results indicate that SO exposure in blood may induce high molecular weight DNA fragmentation due to oxidative stress. Although humans may metabolize styrene monomer to SO, correlations between inhalation exposure to SO and biomarker formation were much stronger than correlations between exposure to styrene and biomarker formation.
Absorption of SO after oral and intraperitoneal administration to experimental animals has been demonstrated (reviewed in IARC 1994a). After intraperitoneal administration, SO is rapidly absorbed and cleared from the blood of mice (Bidoliet al.1980, cited in IARC 1994a).After a single intraperitoneal injection of 200 mg/kg of SO in corn oil, the peak SO plasma concentration (40±7µg/mL) was reached within 7 minutes, and the chemical was no longer detectable at 60 minutes. The area under the curve for the time course of blood concentration of SO was 329 min x µg/g. Langvardt and Nolan (1991, cited in IARC 1994a) studied SO pharmacokinetic parameters in male Fischer 344 rats after oral administration of SO at doses of 275 or 550 mg/kg. They reported highly variable blood concentrations ranging from 0.27 to 8.84 µg/mL in the low-dose animals and 2.1 to 32.4 µg/mL in the high-dose animals. Areas under the curve for the time course of blood concentration of SO after the low and high doses were 47 and 286 min x µg/g, respectively.
Kessler et al.(1992, cited in IARC 1994a) confirmed highly variable absorption of SO after oral administration to Sprague-Dawley rats and B6C3F1mice. They also demonstrated poor bioavailability of SO after oral administration. In these experiments both species received oral or intraperitoneal doses of 200 mg/kg of SO. The areas under the curve after injection or oral administration were 18 and 0.76 h x µg/mL in rats and 12 and 0.01 h x µg/mL in mice, respectively. Reduced bioavailability of SO after oral administration was considered to reflect its hydrolysis in the acidic environment of the stomach.Acid-catalyzed hydrolysis of SO was previously demonstrated in vitro(Rosset al. 1982, cited in IARC 1994a).
Metabolism
Rats and guinea pigs can metabolize SO, as demonstrated by excretion of the SO metabolite 3,4-dihydroxy-3,4-dihydro-1-vinylbenzene after SO administration (Nakatsu et al.1983, cited in IARC 1985, 1994a). Microsomal and cytosolic enzymes mediate the mammalian metabolism of SO. The proposed metabolic scheme, based on isolated mammalian urinary metabolites, is shown in Figure 6-1 (Vainio et al.1984, cited in IARC 1985).
SO is primarily detoxified by metabolism to styrene glycol (phenylethylene glycol) (Carlson 1998). Conversion of SO to styrene glycol is catalyzed by both microsomal and cytosolic epoxide hydrolases and enzyme preparations from mammalian liver, kidney, intestine, lungs, and skin in vitro (Oesch 1973, cited in IARC 1985). Hepatic and pulmonary microsomal preparations from non-Swiss albino and CD-1 mice were compared for their abilities to metabolize racemic, S-, and R-SO to styrene glycol (Carlson 1998). The enzymatic activity was found to be higher in liver than in lung tissues. When human cytosolic and microsomal systems were compared with respect to SO metabolism, microsomal activity was greater than that residing in the cytosol (Schladt et al.1988, cited in IARC 1994a).
Human microsomal epoxide hydrolase (hmEH) was shown to protect Chinese hamster cells from SO-induced DNA damage. Herrero et al.(1997) inserted the cDNA of hmEH into V79 Chinese hamster cells, then challenged the cells with SO. Cells not containing hmEH responded to SO with DNA single-strand breaks and the generation of alkali-labile sites. One of the cell clones, designated 92hmEH-V79, was refractory to SO-induced DNA damage relative to mock-transfected cells. In extensions of the experiment, the protection against SO-induced genotoxicity afforded by the presence of hmEH was reversed by addition of valpromide, a selective inhibitor of microsomal epoxide hydrolase, to the incubation medium. Further, the observed protection against genotoxicity was specific for SO, as ethylene oxide-induced DNA damage was not affected by the presence of 92hmEH-V79. The metabolic product of the action of microsomal epoxide hydrolase on SO has been shown to be styrene glycol. This metabolic step is completed in the absence of NADPHgenerating system (Leibman and Ortiz 1970, cited in IARC 1976). Microsomal enzymes that carry out the initial metabolic step are inducible, as evidenced by the fact that in vitro metabolism of SO by hepatic enzymes from rats is enhanced by pretreatment of animals with phenobarbital or 3-methylcholanthrene. Enzyme activities engaged in the subsequent metabolism of styrene glycol to mandelic acid are not enhanced by the induction of microsomal enzymes (Oesch et al.1971, cited in IARC 1976, 1985). Early corroborative evidence for the styrene glycol metabolic path for SO came from by demonstration that administration of either SO or styrene glycol to rats resulted in the urinary excretion of phenylglyoxylic acid, mandelic acid, and hippuric acid. Injection of mandelic acid resulted in urinary excretion of phenylglyoxylic acid and hippuric acid. However, administration of phenylglyoxylic acid resulted in the appearance of only unchanged phenylglyoxylic acid in the urine (Ohtsuji and Ikeda 1971, cited in IARC 1976). Isolated, perfused rat liver rapidly metabolizes SO to styrene glycol and mandelic acid (Ryan and Bend 1977, Steele et al.1981, cited in IARC 1994a).
The respective figure is attached in a seperate document.
Figure 1. Metabolic pathways of SO
In addition to the oxidative metabolites, rats convert SO to glutathione conjugates, which
are excreted via the kidney.
(Maestriet al.1997).
Conjugation of SO with glutathione is catalyzed by glutathione S-epoxide transferase (GST) (James and White 1967, cited in IARC 1976). Among mammalian species, GST exists in multiple forms, which are classified under four multigene families: alpha, mu, pi, and theta. (The GST families are alternatively designated as GSTA, GSTM, GSTP,
and GSTT.) The families have different but sometimes overlapping substrate specificities
(Gopalan-Kriczky et al.1994). Human liver cytosolic GST activity has been shown to
occur in two forms,ìandá, with theìform being more active in SO metabolism
(Pacifici et al.1987, cited in IARC 1994a).
Maestri et al.(1997) reported urinary excretion of small amounts ofN-acetyl-S-(1- phenyl-2-hydroxyethyl)-cysteine and N-acetyl-S-(2-phenyl-2-hydroxyethyl)-cysteine by humans occupationally exposed to styrene. The conversion of SO to mercapturic acids by humans is generally considered to be a minor metabolic pathway. Despite its purportedly minor role in hepatic metabolism of SO in humans, it is noteworthy that GST may play an important role(s) in preventing DNA adduct formation by reactive metabolites, including styrene epoxide, by catalyzing glutathione conjugate formation. Genes encoding GSTM and GSTT are polymorphic in humans, and the polymorphisms result in deletion of the genes in some individuals. In fact, about 50% of the Caucasian population is deficient in GSTM, while the GSTT gene is absent in 10% to 20% of the population (Seidegard et al.1988; Hirvonen et al.1993; Pemble et al.1994; and Nelson et al.1995).Ollikainen et al.(1998) reported that GSTT affords some level of protection against induction of SCE in cultured human lymphocytes exposed to SO. Lymphocytes from humans genetically deficient in GSTT and lymphocytes from GSTT positive humans were cultured in the presence of SO at a concentration of 50 µM or 150 µM. Although the presence of SO caused SCEs in all lymphocytes, significantly more appeared in GSTT-deficient lymphocytes than in GSTT-positive lymphocytes (by factors of 1.7 and 1.4 at SO concentrations of 50 µM and 150 µM, respectively). Similar experiments with lymphocytes from GSTM-deficient donors (Uuskula et al.1995) demonstrated that the presence or absence of GSTM had no effect on the induction of SCEs by SO. However, the presence of GSTM did protect lymphocytes against induction of SCEs by 1,2-epoxide-3-butene. Observations that GSTs may protect against epoxide-induced adduct formation deserve serious follow-up in pursuit of information about the mechanism of SO carcinogenesis. The enzymes that metabolize SO are stereoselective, with the S enantiomer favored over the R enantiomer in hydrolysis by epoxide hydrolase (Watabe et al.1981, cited in IARC 1994a). In contrast, glutathione S-transferases favor the R isomer (Hiratsuka et al.1989, cited in IARC 1994a). H
Human liver has been compared with liver from Fischer 344 and Sprague-Dawley rats and B6C3F1mice with respect to activities of cytochrome P-450 monooxygenase, microsomal and cytosolic forms of epoxide hydrolase, and glutathione S-transferase in the in vitro metabolism of SO (Mendrala et al.1993, cited in IARC 1994a). The affinities of the monooxygenases (inverse Kmvalues) were essentially similar across species: 0.09 mmol in humans and 0.05 mmol in mice. The Vmaxvalues were similar in rats and mice (9.3 to 13 nmol/mg protein per minute) but lower in the human liver samples (2.1 nmol/mg protein per minute). The Kmvalues for epoxide hydrolase were low in humans (0.01 mmol), intermediate in rats (0.13 to 0.23 mmol), and highest in mice (0.74 mmol) but the Vmaxvalues for epoxide hydrolase were similar among all species. Humans had the lowest glutathione S-transferase activity towards SO. These findings are consistent with the indirect observations of SO metabolism (identification of urinary metabolites) in these species.
Elimination
The primary route of excretion for SO metabolites in mammalian species is via urine. In rabbits, about 80 % of a single oral dose was excreted in the urine (James and White 1967, cited in IARC 1994a). Excretion of acidic metabolites derived from glutathione conjugates varies qualitatively among species. In rats, the only glutathione conjugation products detected in urine are the mercapturic acids, whereas in guinea pigs, the major metabolites are mercaptoacetic acids together with mercaptolactic, mercaptopyruvic, and mercapturic acids.
Information on Registered Substances comes from registration dossiers which have been assigned a registration number. The assignment of a registration number does however not guarantee that the information in the dossier is correct or that the dossier is compliant with Regulation (EC) No 1907/2006 (the REACH Regulation). This information has not been reviewed or verified by the Agency or any other authority. The content is subject to change without prior notice.
Reproduction or further distribution of this information may be subject to copyright protection. Use of the information without obtaining the permission from the owner(s) of the respective information might violate the rights of the owner.
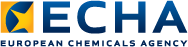