Registration Dossier
Registration Dossier
Data platform availability banner - registered substances factsheets
Please be aware that this old REACH registration data factsheet is no longer maintained; it remains frozen as of 19th May 2023.
The new ECHA CHEM database has been released by ECHA, and it now contains all REACH registration data. There are more details on the transition of ECHA's published data to ECHA CHEM here.
Diss Factsheets
Use of this information is subject to copyright laws and may require the permission of the owner of the information, as described in the ECHA Legal Notice.
EC number: 225-768-6 | CAS number: 5064-31-3
- Life Cycle description
- Uses advised against
- Endpoint summary
- Appearance / physical state / colour
- Melting point / freezing point
- Boiling point
- Density
- Particle size distribution (Granulometry)
- Vapour pressure
- Partition coefficient
- Water solubility
- Solubility in organic solvents / fat solubility
- Surface tension
- Flash point
- Auto flammability
- Flammability
- Explosiveness
- Oxidising properties
- Oxidation reduction potential
- Stability in organic solvents and identity of relevant degradation products
- Storage stability and reactivity towards container material
- Stability: thermal, sunlight, metals
- pH
- Dissociation constant
- Viscosity
- Additional physico-chemical information
- Additional physico-chemical properties of nanomaterials
- Nanomaterial agglomeration / aggregation
- Nanomaterial crystalline phase
- Nanomaterial crystallite and grain size
- Nanomaterial aspect ratio / shape
- Nanomaterial specific surface area
- Nanomaterial Zeta potential
- Nanomaterial surface chemistry
- Nanomaterial dustiness
- Nanomaterial porosity
- Nanomaterial pour density
- Nanomaterial photocatalytic activity
- Nanomaterial radical formation potential
- Nanomaterial catalytic activity
- Endpoint summary
- Stability
- Biodegradation
- Bioaccumulation
- Transport and distribution
- Environmental data
- Additional information on environmental fate and behaviour
- Ecotoxicological Summary
- Aquatic toxicity
- Endpoint summary
- Short-term toxicity to fish
- Long-term toxicity to fish
- Short-term toxicity to aquatic invertebrates
- Long-term toxicity to aquatic invertebrates
- Toxicity to aquatic algae and cyanobacteria
- Toxicity to aquatic plants other than algae
- Toxicity to microorganisms
- Endocrine disrupter testing in aquatic vertebrates – in vivo
- Toxicity to other aquatic organisms
- Sediment toxicity
- Terrestrial toxicity
- Biological effects monitoring
- Biotransformation and kinetics
- Additional ecotoxological information
- Toxicological Summary
- Toxicokinetics, metabolism and distribution
- Acute Toxicity
- Irritation / corrosion
- Sensitisation
- Repeated dose toxicity
- Genetic toxicity
- Carcinogenicity
- Toxicity to reproduction
- Specific investigations
- Exposure related observations in humans
- Toxic effects on livestock and pets
- Additional toxicological data

Biodegradation in water and sediment: simulation tests
Administrative data
Link to relevant study record(s)
Description of key information
In simulation tests, the biodegradation of sodium nitrilotriacetate (Na3NTA) or the acid H3NTA was measured in the laboratory using procedures and bacterial inoculates that simulate potential degradation in specific environmental compartments. Simulation tests for the biodegradation of Na3NTA or NTA are available for the environmental compartments freshwater, estuarine water and freshwater sediment, Information relating to anaerobic sediment is limited, therefore studies on similar conditions in water logged soil and activated sludge are included. In addition, there are references to biodegradation pathways of NTA in open literature.
Key value for chemical safety assessment
- Half-life in freshwater:
- 120 h
- at the temperature of:
- 14 °C
- Half-life in freshwater sediment:
- 215 h
- at the temperature of:
- 30 °C
Additional information
Besides studies on trisodium nitrilotriacetate, also studies relating to both nitrilotriacetic acid (NTA acid, H3NTA) and nitrilotriacetate are considered for the assessment of this endpoint:
NTA acid, Na3NTA, and nitrilotriacetate display the same behaviour in the environment: splitting of sodium ions or protons (in case of Na3NTA and NTA acid) and uptake of multivalent metal ions with subsequent formation of 1:1 or 1:2 complexes.
Since sodium salts are generally considered to be completely dissociating, a solution of Na3NTA in water yields the tribasic anion nitrilotriacetate. Nitrilotriacetic acid is a weak acid, and in such a solution, the NTA will therefore exist as an equilibrium mixture of several species:
NTA- - -<-> HNTA- -<-> H2NTA-<-> H3NTA <-> H4NTA+
with the last species occurring when, in a very acidic environment, the central nitrogen atom is protonated.
Due to pH differences, the NTA speciation equilibrium will be different for Na3NTA and for NTA acid, unless dissolved in a buffered solution (controlled pH). A solution of NTA acid will be (slightly) acidic, whereas a Na3NTA solution will be alkaline (‘basic’). Toxicologically, this is not assumed to be significant, since it can be presumed that ‘in vivo’ systems are buffered systems. The chelating behaviour of Na3NTA and NTA acid will be slightly different, but this is not a significant effect for the relevant endpoint under REACH with regard to environmental fate and behaviour, ecotoxicology and toxicology.
Therefore, also results on NTA acid and nitrilotriacetate are considered for the assessment of trisodium nitrilotriacetate using read-across. This is in line with the Canadian ‘Draft Screening Assessment for Nitrilotriacetic acid (CAS 139-13-9)’ from January 2010, which also considered information relating to Na3NTA and nitrilotriacetate in the assessment of NTA acid. This is due to the fact that the toxicological endpoints, as stated in the Canadian ‘Screening Assessment for Nitrilotriacetic acid’, of NTA acid and Na3NTA are similar. Moreover, the dissociation of NTA acid and Na3NTA leads to the common moiety nitrilotriacetate.
Data from studies with salts formed with various cations such as calcium, magnesium, aluminum, zinc and iron were not included. Canada and the European Union also similarly did not include these other NTA salts in the ‘Draft Screening Assessment for Nitrilotriacetic acid’ and the ‘Draft Risk Assessment Report (EURAR 2008)’, respectively.
Under freshwater conditions, Larson & Davidson (1982) found aerobic mineralisation half lives of 7 to 138 hours (initial 14C-Na3NTA, 50 and 5 μg/L) at 14 or 24 °C. Acclimation and degradation Na3NTA was encountered at the lowest tested concentrations in rivers not previously exposed to NTA, indicating no threshold effect. Degradation in water samples with prior NTA exposure required no acclimation period and were less variable (t1/2= 7-17 h). This suggests that river microflora are capable of acclimating to and degrading NTA at trace levels, however biodegradation is more efficient in natural waters with previous NTA exposure.
Temperature was found to have a marked effect upon biodegradation rates of NTA acid by Shannon et al (1974). In a simulated freshwater system with sediment present, first order degradation rate coefficients ranged between 0.036/h at 18 °C (t1/2 = 115 h) and 0.006/h at 2 °C (t1/2= 19 h). NTA acid degraded in freshwaters at low temperature, and was readily biodegradable at temperatures over 10°C. Additional field studies from a natural river environment downstream of a wastewater treatment plant (8-20 mg/L initial NTA acid input) indicated the effects of dilution and degradation gave downstream NTA aicd concentrations of less than 10 µg/L at higher summer temperatures (16.5-21 ºC), ranging up to 125 µg/L at lower winter temperatures (0.5-3.0 ºC).
Larson and Ventullo (1986) reported first-order kinetics and estimated mineralisation half-life of ~2 days in an estuarine water system with prior history of Na3NTA exposure. Degradation had no lag phase, indicating prior adaption of bacteria to Na3NTA. No consistent effect of salinity (4 – 19 %) or DOC (2-12 mg/l) on Na3NTA degradation rates was found under the range of conditions. Na3NTA degradation was rapid in estuarine waters over the range of salinities and DOC levels tested.
Hunter et al (1986) studied biodegradation of Na3NTA in an estuarine simulation with salinity gradient. Following an acclimation period of 11 days, >95 % mean NTA removal was observed in salinities up to 9.18 with low NTA levels (0.97-1.02 µg/L). NTA removal was reduced to 48-77% at higher salinities. At higher NTA doses (6.95-10.16 µg/L) over 99 % NTA removal was observed up to salinities of 5.05 %; at higher salinities, NTA removal ceased.
In an aerobic freshwater sediment system, McFeters et al (1990) measured the half life of NTA as c.1 day (at 30 °C) for samples from a river with persistent NTA loading and one order of magnitude slower for pure lab strain associated with sand. Lag time from sediment from a pristine site was 5 days followed by sudden NTA degradative activity. The temperature used was above typical environmental conditions therefore due to temperature effects, the “real-world” degradation rate may be lower than the experimental result.
No studies with anaerobic sediments are available. However Stephenson et al (1983a) found NTA acid to be biodegradable in anaerobic sludge in laboratory scale digesters at temperatures of 35 °C. Aerobically NTA-acclimatised waste activated sludge caused NTA acid concentrations to fall from 10-15 mg/L to less than 0.5 mg/L in 8-22 days with 4-16 d lag time. Degradation after further addition of NTA acid after up to 30 days of hiatus proceeded rapidly due to a memory effect.The rapid decrease of NTA acid concentration in sludge indicates that NTA acid was removed via biodegradation rather than adsorption onto solids; a higher residual concentration would be expected if this were not the case. No NTA acid biodegradation occurred after 40 days following introduction of unacclimatised waste activated sludge. Stephenson et al (1983a) also noted the effect of temperature on NTA acid degradation; >95 % removal was achieved in-situ by treatment plant under warmer summer conditions where as <50 % removal occurred during the winter period, this was independent of NTA acid dosage.
Tabatabai and Bremmer (1975) found Na3NTA to be readily biodegradable under aerobic and waterlogged (anaerobic) soil conditions (99-101 % to 0-10 % Na3NTA recovery) after 10 days. The decomposition of Na3NTA was investigated by analysis of Na3NTA and inorganic nitrogen. Under waterlogged conditions, Na3NTA-nitrogen was recovered primarily as NH4+ and under aerobic conditions, Na3NTA-nitrogen was predominantly converted to nitrate. The formation of NO3- indicates that nitrate enrichment may occur as a by product of Na3NTA biodegradation in natural environments.
In contrast to the findings of Tabatabai and Bremner (1975),the results Dunlap et al. (1971) with anaerobic sand, loam, and clay-loam soil columns demonstrate slow and incomplete biodegradation of Na3NTA, indicating that Na3NTA infiltrating through saturated soil systems such as flooded septic tank percolation fields or under waste water lagoons, will likely undergo at most only slow degradation (half-lives were not reported; see IUCLID section 5.2.3).
In summary, the studies demonstrate that Na3NTA is readily degraded under aerobic conditions in fresh waters and freshwater sediments. Biodegradation is enhanced by increased temperature, although was still found to occur even at low temperatures, albeit at a slower rate. Acclimation of bacteria to Na3NTA was demonstrated even at low test concentrations. In saline/estuarine conditions, Na3NTA degradation was found to be rapid over a range of salinities (up to 19 %), however the rate of Na3NTA removal decreases at higher salinities and the effect of high salinity was found to be compounded under high Na3NTA dose conditions. This suggests that the role of microbes in degradation of Na3NTA may be limited in marine conditions if initial doses are high; dilution and other degradation processes may be dominant in the marine system. Data relating to anaerobic sediments is limited, and available data on anaerobic degradation in saturated soils are contradictory. However, Na3NTA was shown to be degraded in anaerobic sludges, which have been acclimatised. Na3NTA degradation rates in aerobic sludge are found to be reduced at low temperature; demonstrated in field rather than laboratory conditions.
Metabolic Pathways
Egli (1992) reviewed recent literature regarding the pathways of degradation of NTA acid. In an obligately aerobic Chelatobacter, a monooxygenase enzyme was responsible for NTA oxidation to iminodiacetate (IDA) and glyoxylate. A membrane-bound dehydrogenase (IDA-DH) was responsible for the subsequent oxidation of IDA to glycine and glyoxylate. In a denitrifying bacteria strain, a soluble NTA dehydrogenase/nitrase reductase enzyme complex was responsible for the initial oxidation of NTA and nitrate to form IDA, glyoxylate and nitrite. The reaction pathway supports the result of the standard tests that NTA is completely mineralised after primary degradation and accumulation of a stable metabolite is not expected.
Bacteria growth rate was found to increase where a supplementary carbon source was provided in addition to NTA; no NH4+was excreted where this was the case.
Chelatobacter and Chelatococcus bacteria were found to be ubiquitous in the natural environment. Indirect immunofluorescence indicated a ten-fold increase in positive reactions in activated sludge compared to river waters, suggesting an enrichment of NTA-degrading bacteria in treatment plants.
Numbers of NTA-degrading bacteria were not found to be significantly different between activated sludge of sewage plants with differing NTA elimination efficiencies, indicating NTA-degradation regulation by enzyme-production. Unadapted lab grown bacteria were able to utilize NTA within 6 -8 h post transfer to a sewage works chamber, suggesting induction or derepression of NTA catabolic enzymes as a mechanism.
In summary, studies have shown that NTA is degraded by microorganisms to form intermediates such as iminodiacetate (IDA), glyoxylate, glycine and ammonia, with final metabolic end products including ammonia, nitrates, and carbon dioxide.
Information on Registered Substances comes from registration dossiers which have been assigned a registration number. The assignment of a registration number does however not guarantee that the information in the dossier is correct or that the dossier is compliant with Regulation (EC) No 1907/2006 (the REACH Regulation). This information has not been reviewed or verified by the Agency or any other authority. The content is subject to change without prior notice.
Reproduction or further distribution of this information may be subject to copyright protection. Use of the information without obtaining the permission from the owner(s) of the respective information might violate the rights of the owner.
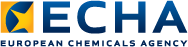