Registration Dossier
Registration Dossier
Data platform availability banner - registered substances factsheets
Please be aware that this old REACH registration data factsheet is no longer maintained; it remains frozen as of 19th May 2023.
The new ECHA CHEM database has been released by ECHA, and it now contains all REACH registration data. There are more details on the transition of ECHA's published data to ECHA CHEM here.
Diss Factsheets
Use of this information is subject to copyright laws and may require the permission of the owner of the information, as described in the ECHA Legal Notice.
EC number: 914-129-3 | CAS number: 12336-95-7
- Life Cycle description
- Uses advised against
- Endpoint summary
- Appearance / physical state / colour
- Melting point / freezing point
- Boiling point
- Density
- Particle size distribution (Granulometry)
- Vapour pressure
- Partition coefficient
- Water solubility
- Solubility in organic solvents / fat solubility
- Surface tension
- Flash point
- Auto flammability
- Flammability
- Explosiveness
- Oxidising properties
- Oxidation reduction potential
- Stability in organic solvents and identity of relevant degradation products
- Storage stability and reactivity towards container material
- Stability: thermal, sunlight, metals
- pH
- Dissociation constant
- Viscosity
- Additional physico-chemical information
- Additional physico-chemical properties of nanomaterials
- Nanomaterial agglomeration / aggregation
- Nanomaterial crystalline phase
- Nanomaterial crystallite and grain size
- Nanomaterial aspect ratio / shape
- Nanomaterial specific surface area
- Nanomaterial Zeta potential
- Nanomaterial surface chemistry
- Nanomaterial dustiness
- Nanomaterial porosity
- Nanomaterial pour density
- Nanomaterial photocatalytic activity
- Nanomaterial radical formation potential
- Nanomaterial catalytic activity
- Endpoint summary
- Stability
- Biodegradation
- Bioaccumulation
- Transport and distribution
- Environmental data
- Additional information on environmental fate and behaviour
- Ecotoxicological Summary
- Aquatic toxicity
- Endpoint summary
- Short-term toxicity to fish
- Long-term toxicity to fish
- Short-term toxicity to aquatic invertebrates
- Long-term toxicity to aquatic invertebrates
- Toxicity to aquatic algae and cyanobacteria
- Toxicity to aquatic plants other than algae
- Toxicity to microorganisms
- Endocrine disrupter testing in aquatic vertebrates – in vivo
- Toxicity to other aquatic organisms
- Sediment toxicity
- Terrestrial toxicity
- Biological effects monitoring
- Biotransformation and kinetics
- Additional ecotoxological information
- Toxicological Summary
- Toxicokinetics, metabolism and distribution
- Acute Toxicity
- Irritation / corrosion
- Sensitisation
- Repeated dose toxicity
- Genetic toxicity
- Carcinogenicity
- Toxicity to reproduction
- Specific investigations
- Exposure related observations in humans
- Toxic effects on livestock and pets
- Additional toxicological data

Adsorption / desorption
Administrative data
Link to relevant study record(s)
- Endpoint:
- adsorption / desorption, other
- Remarks:
- measurement
- Type of information:
- read-across from supporting substance (structural analogue or surrogate)
- Adequacy of study:
- key study
- Justification for type of information:
- REPORTING FORMAT FOR THE ANALOGUE APPROACH
1. HYPOTHESIS FOR THE ANALOGUE APPROACH
Typically salts (ionic bonding) are electrovalent substances. Electrovalent substances are made up of ions in the solid state. The oppositely charged ions are held together by strong electrostatic (coulombic) force of attraction. Due to these forces the ions cannot move. When these substances are dissolved in water, the ions free themselves from this binding. Thus the break up of an electrovalent compound into free mobile ions when dissolved in water or when melted, is called electrolytic dissociation. In the liquid state the ions become free and mobile. But the oppositely charged ions always remain in close proximity of each other. Cr(OH)(SO4)(s) ---> Cr3+(aq) + SO42- (aq) + OH- (aq). This is a 100% dissociation. This multi-constituent substance contains numerous ionic species that form complex equilibria in water which change over an environmentally-relevant pH range. So, the chromium species of interest is chromium 3+, which is also available from other salts, e.g. chromium(III) chloride hexahydrate.
2. SOURCE AND TARGET CHEMICAL(S) (INCLUDING INFORMATION ON PURITY AND IMPURITIES)
Please refer to test material.
3. ANALOGUE APPROACH JUSTIFICATION
As the test substance contains chromium 3+, which is also present in the source substance and becasue chromium 3+ is the species of interest, to fulfil the data requirements of Regulation EC No 1907/2006 Annex VII, it is fully justified to adress this endpoint with data of an other chromium 3+ containig substance. - Reason / purpose for cross-reference:
- read-across source
- Sample No.:
- #1
- Type:
- Kd
- Value:
- 831 L/kg
- Matrix:
- loam
- % Org. carbon:
- 1.92
- Sample No.:
- #2
- Type:
- Kd
- Value:
- 2 625 L/kg
- Matrix:
- Sild
- % Org. carbon:
- 0.11
- Sample No.:
- #3
- Type:
- Kd
- Value:
- 5 283 L/kg
- Matrix:
- clay
- % Org. carbon:
- 3.75
- Transformation products:
- yes
- No.:
- #1
- Validity criteria fulfilled:
- not specified
- Conclusions:
- Conclusions
(1) Cr(III) reacts rapidly with soil solids. Within 6 hours of the addition of about 0.5 mg to a soil-water slurry (0.15 g of soil), no Cr(III) was detectable in any of the three EPA soil porewaters. It is removed by precipitation as various Cr(III) species or by sorption to various soil components.
(2) The lag time for detection of hexavalent chromium in porewaters formed by oxidation of Cr(III) depends on the organic carbon content of the soil. At low TOC, immediate formation of Cr(VI) was observed. Soils of medium to high TOC began to show Cr(VI) in their porewaters within 2 hours of addition. This indicates that higher levels of carbon may block mineral sites that oxidize Cr(III).
(3) Cr(III) solutions in water without soil showed no Cr(VI) formation, even after shaking for 168 hours. This excludes the possibility of direct oxidation by atmospheric oxygen. In addition, because Cr(III) adsorbs rapidly to the solid phase, the adsorbed Cr(III) forms, not the soluble forms, must be the species oxidized to Cr(VI), and the oxidants must be part of the soil solids.
( 4) The concentration of soluble Mn(II) ions in certain soil porewaters is elevated after addition of Cr(III), which indicates that Mn(VI) in soil minerals is responsible for oxidation of Cr(III) after its adsorption. The porewater Eh of soils containing Cr(III) gradually decreases due to reduction of those strong oxidants present in the soil solids. The pH of soil porewaters increases between 1 and 2 units in 1 week's time after the addition of Cr(III).
(5) The yield of Cr(VI) formed by oxidation of added Cr(III) can reach significant levels - after 6 hours about 2% of the added Cr appeared as Cr(VI).
(6) Different equilibration times and initial Cr(III) concentrations result in different total chromium Kd values. At early stages of mixing Cr(III) with the soil porewater slurries, adsorption reactions are not at
equilibrium; low Kd values are observed. Later, the Kd reaches a maximum value. For example, EPA no. 2 soil (#1) exhibited a maximum Kd after 6 hours of equilibration, whereas the Kd of EPA no. 9 (#2) reached a maximum after 24 hours.
(7) Cr(III) concentrations in soil porewaters increase as pH decreases, whereas Cr(VI) concentrations increase as pH increases; this is in accord with the cationic and anionic nature, respectively, of these species and the charge on the soil organic carbon, which is a function of pH. The pH influences the electrostatic interactions that serve to remove Cr from solution.
(8) The sorption of Cr(III) decreases with increased porewater concentration of cations, which compete for the adsorption sites on the soils. Increased porewater concentration of anions, however, has very slight effect on the sorption of Cr(VI) anions.
(9) The magnitude of the Kd values measured at a constant equilibration time and under the natural conditions of the soil-water slurries are much lower for Cr(VI), ranging from less than 1 to about 50 L/kg for the 3 soils studied. For Cr(III), the Kd values range from about 850 to 5,600 L/kg.The TOC concentration of the soil has an important effect on the Kd values of both Cr(III) and Cr(VI). - Executive summary:
The distribution of chromium species between soil and porewater was assessed by Hassan and Garrison in 1996 and the study was published in Chemical Specification and Bioavailability.
Batch experiments were carried out using three soils representing different geologic parts of the continental USA to identify processes influencing partitioning and interconversion of chromium species in soil porewaters. The partition coefficient (Kd) of each of the two main species, Cr(lll) and Cr(VI), was calculated for each soil using adsorption isotherms measured after an equilibration time of 6 hours for the former species and 48 hours for the latter at the natural conditions of the soils. Cr(VI) in porewater was measured directly by ion chromatography, while total chromium was measured by inductively coupled plasma- atomic emission spectrophotometry (ICP-AES). Total chromium in the soil solids was measured by ICP-AES after microwave assisted digestion. The magnitude of the Kd values are much lower for Cr(VI), ranging from less than 1 to about 50 L/kg. For Cr(III), the Kd values range from about 850 to 5,600 L/kg. The effects of the initial porewater concentration of the chromium species, the equilibration time, and the pH, Eh and ion concentration of the porewater on the Kd values were investigated; the results are discussed relative to the differences in physical and chemical properties of the soils. Cr(III) concentration in porewater increases as pH decreases, but Cr(VI) increases as pH increases. The sorption of Cr(III) decreases with increased porewater concentration of cations, but increased concentration of common anions has
slight effect on the sorption of Cr(VI) anions. The Kd values of both Cr(III) and Cr(VI) depend on equilibration time because of the kinetics of interconversion of species upon reaction with the soil and the resultant change in total chromium in the porewater with time. Cr(III) added to porewater reacts rapidly with the soil through adsorption and/or precipitation; some is then oxidized by Mn(IV)-containing minerals within a few hours, liberating both Cr(VI) and Mn(ll) into the porewater. The yield of Cr(VI) can reach significant levels relative to the 100 µg/L drinking water standard, and the Cr(VI) disappears from porewater at a very slow rate compared to the disappearance of Cr(III).
Reference
Effect of equilibration time on Cr(III) adsorption and oxidation to Cr(VI)
Effect of pH on the adsorption profile of Cr(lll)
Effect of Eh on the adsorption of Cr(III),
Effect of ionic strength on the adsorption of Cr(Ill)
Effect of TOC - adsorption isotherms
please refer to the attached publication for detailed description
Conclusions
(1) Cr(III) reacts rapidly with soil solids. Within 6 hours of the addition of about 0.5 mg to a soil-water slurry (0.15 g of soil), no Cr(III) was detectable in any of the three EPA soil porewaters. It is removed by precipitation as various Cr(III) species or by sorption to various soil components.
(2) The lag time for detection of hexavalent chromium in porewaters formed by oxidation of Cr(III) depends on the organic carbon content of the soil. At low TOC, immediate formation of Cr(VI) was observed. Soils of medium to high TOC began to show Cr(VI) in their porewaters within 2 hours of addition. This indicates that higher levels of carbon may block mineral sites that oxidize Cr(III).
(3) Cr(III) solutions in water without soil showed no Cr(VI) formation, even after shaking for 168 hours. This excludes the possibility of direct oxidation by atmospheric oxygen. In addition, because Cr(III) adsorbs rapidly to the solid phase, the adsorbed Cr(III) forms, not the soluble forms, must be the species oxidized to Cr(VI), and the oxidants must be part of the soil solids.
( 4) The concentration of soluble Mn(II) ions in certain soil porewaters is elevated after addition of Cr(III), which indicates that Mn(VI) in soil minerals is responsible for oxidation of Cr(III) after its adsorption. The porewater Eh of soils containing Cr(III) gradually decreases due to reduction of those strong oxidants present in the soil solids. The pH of soil porewaters increases between 1 and 2 units in 1 week's time after the addition of Cr(III).
(5) The yield of Cr(VI) formed by oxidation of added Cr(III) can reach significant levels - after 6 hours about 2% of the added Cr appeared as Cr(VI).
(6) Different equilibration times and initial Cr(III) concentrations result in different total chromium Kd values. At early stages of mixing Cr(III) with the soil porewater slurries, adsorption reactions are not at
equilibrium; low Kd values are observed. Later, the Kd reaches a maximum value. For example, EPA no. 2 soil (#1) exhibited a maximum Kd after 6 hours of equilibration, whereas the Kd of EPA no. 9 (#2) reached a maximum after 24 hours.
(7) Cr(III) concentrations in soil porewaters increase as pH decreases, whereas Cr(VI) concentrations increase as pH increases; this is in accord with the cationic and anionic nature, respectively, of these species and the charge on the soil organic carbon, which is a function of pH. The pH influences the electrostatic interactions that serve to remove Cr from solution.
(8) The sorption of Cr(III) decreases with increased porewater concentration of cations, which compete for the adsorption sites on the soils. Increased porewater concentration of anions, however, has very slight effect on the sorption of Cr(VI) anions.
(9) The magnitude of the Kd values measured at a constant equilibration time and under the natural conditions of the soil-water slurries are much lower for Cr(VI), ranging from less than 1 to about 50 L/kg for the 3 soils studied. For Cr(III), the Kd values range from about 850 to 5,600 L/kg.The TOC concentration of the soil has an important effect on the Kd values of both Cr(III) and Cr(VI).
Description of key information
The distribution of chromium species between soil and porewater was assessed by Hassan and Garrison in 1996 and the study was published in Chemical Specification and Bioavailability.
Batch experiments were carried out using three soils representing different geologic parts of the continental USA to identify processes influencing partitioning and interconversion of chromium species in soil porewaters. The partition coefficient (Kd) of each of the two main species, Cr(lll) and Cr(VI), was calculated for each soil using adsorption isotherms measured after an equilibration time of 6 hours for the former species and 48 hours for the latter at the natural conditions of the soils. Cr(VI) in porewater was measured directly by ion chromatography, while total chromium was measured by inductively coupled plasma- atomic emission spectrophotometry (ICP-AES). Total chromium in the soil solids was measured by ICP-AES after microwave assisted digestion. The magnitude of the Kd values are much lower for Cr(VI), ranging from less than 1 to about 50 L/kg. For Cr(III), the Kd values range from about 850 to 5,600 L/kg. The effects of the initial porewater concentration of the chromium species, the equilibration time, and the pH, Eh and ion concentration of the porewater on the Kd values were investigated; the results are discussed relative to the differences in physical and chemical properties of the soils. Cr(III) concentration in porewater increases as pH decreases, but Cr(VI) increases as pH increases. The sorption of Cr(III) decreases with increased porewater concentration of cations, but increased concentration of common anions has
slight effect on the sorption of Cr(VI) anions. The Kd values of both Cr(III) and Cr(VI) depend on equilibration time because of the kinetics of interconversion of species upon reaction with the soil and the resultant change in total chromium in the porewater with time. Cr(III) added to porewater reacts rapidly with the soil through adsorption and/or precipitation; some is then oxidized by Mn(IV)-containing minerals within a few hours, liberating both Cr(VI) and Mn(ll) into the porewater. The yield of Cr(VI) can reach significant levels relative to the 100 µg/L drinking water standard, and the Cr(VI) disappears from porewater at a very slow rate compared to the disappearance of Cr(III).
Key value for chemical safety assessment
Additional information
Discussion of adsorption coefficients for chromium
The estimation methods given in the main Technical Guidance Document for determining adsorption coefficients for soil, sediment and suspended sediment are not applicable to chromium compounds, as indicated in the Annex on assessment of metals. Measured values are available for a variety of soil and sediment types.
The table below summarises the published values for Kpwater-solids for both freshwater and marine environments. The values are reported according to a variety of methods and may not be directly comparable; however, they do give a general indication of the partitioning total chromium and/or chromium (III) for several environmental compartments. In general, chromium (III) is more likely to partition to solids in the sediment and soil. For the water column, chromium (III) has adsorption partition coefficients for suspended solids, which are greater than those found for sediment and soil.
Summary of measured partition coefficients (Kp) for chromium
Phase |
Kp (l/kg) |
Comments |
Reference |
||
Total Cr |
Cr(III) |
||||
Suspended sediment partition coefficients |
|||||
Kpsusp |
25,000-800,000 |
Freshwater and saltwater |
Braunschweiler et al. (1996) |
||
126,000-786,000 |
Freshwater, based on routine water quality data from The Netherlands |
Van Der Kooij et al. (1991) |
|||
30,100-1,059,600; mean 322,400 |
Freshwater River suspended sediments, United States - based on monitoring data |
Young et al. (1987) |
|||
324,000 |
Saltwater (0.1-0.5‰), based on routine water quality data from The Netherlands |
Van Der Kooij et al. (1991) |
|||
306,000-320,000 |
Saltwater (1-5‰), based on routine water quality data from The Netherlands |
||||
228,000 |
Saltwater (>10‰), based on routine water quality data from The Netherlands |
||||
140,000-570,000 |
Freshwater (Rhine-Meuse delta) |
Golimowski et al. (1990) |
|||
29,200-200,000 |
Saltwater (Tyrrhenian Sea), silty-clay sediment, organic carbon content 1.6%, salinity 3.8‰, pH 8.2-8.3. |
Ciceri et al. (1992) |
|||
Sediment-water partition coefficients |
|||||
Kpsed |
34,000 |
Saltwater, organic matter content ~2%, pH 7.8-8.0 |
Wang et al. (1997) |
||
Saltwater, organic matter content ~10%, pH 7.8-8.0 |
|||||
25,600-32,800 |
Freshwater, pH 8.3, organic carbon content 2.65% |
Young et al. (1987) |
|||
60-44,800; mean 7,100 |
Freshwater River sediments, United States -based on monitoring data |
||||
11,000 |
Freshwater, pH=4.5 |
Young et al. (1992) |
|||
120,000 |
Freshwater, pH >6 |
||||
Soil-water partition coefficients |
|||||
Kpsoil |
524-24,217 |
Dutch field soils, pH~3.8-7.9; 2-21.8% organic matter |
Janssen et al. (1997) |
||
298-788 |
Loam; pH 6; 1.92% organic carbon |
Hassan and Garrison (1996) |
|||
2,823-15,382 |
Loam; pH >6; 1.92% organic carbon |
||||
Loess; pH6; 0.11% organic carbon |
|||||
19,716-55,918 |
Loess; pH>6; 0.11% organic carbon |
||||
330-27,151 |
Clay; pH7; 3.75% organic carbon |
||||
Clay; pH>7; 3.75% organic carbon |
|||||
12-27 |
Sand, pH 4-8 |
Pérez et al. (1988) |
|||
21-197 |
Sandy soil, pH 4-8, 0.77% organic matter |
||||
116-608 |
Sandy loam, pH 4-8, 1.62% organic matter |
Chromium (III) is strongly adsorbed to soils and sediments; the adsorption of chromium (III) onto soil follows the pattern typical of cationic metals and increases with increasing pH (lowering pH results in increased protonation of the adsorbent leading to fewer adsorption sites for the cationic metal) and the organic matter content of the soil and decreases when other competing (metal) cations are present. Certain dissolved organic ligands may also reduce the adsorption of chromium (III) to the solid phase by forming complexes which enhance the solubility of chromium (III) in the aqueous phase. Based on the available measured values for the adsorption coefficients, the values indicated below will be used in the risk assessment. These values are not taken directly from specific tests, but have been chosen to be representative for acidic-neutral and neutral-alkaline environments. The values do not correspond to any specific individual test results, nor are they derived statistically from the available data (since these are insufficient to allow meaningful values to be derived). Instead they were selected by inspection of the data to reflect the available information under the two sets of conditions and to reflect the differences between these. Acid-neutral environments are considered to be those at pH 5 and below; neutral-alkaline environments are taken to be those at pH 6 and above.
Chromium (III) Acid conditions Alkaline conditions
Kpsusp = 30,000 l/kg Kpsusp = 300,000 l/kg
Kpsed = 11,000 l/kg Kpsed = 120,000 l/kg
Kpsoil = 800 l/kg Kpsoil = 15,000 l/kg
The equivalent values for the dimensionless form of the partition coefficient using the methods given in the Technical Guidance document are:
Chromium (III) Acid conditions Alkaline conditions
Ksusp-water = 7,500 m³/m³ Ksusp-water = 75,000 m³/m³
Ksed-water = 5,500 m³/m³ Ksed-water = 60,000 m³/m³
Ksoil-water = 1,200 m³/m³ Ksoil-water = 22,500 m³/m³
Information on Registered Substances comes from registration dossiers which have been assigned a registration number. The assignment of a registration number does however not guarantee that the information in the dossier is correct or that the dossier is compliant with Regulation (EC) No 1907/2006 (the REACH Regulation). This information has not been reviewed or verified by the Agency or any other authority. The content is subject to change without prior notice.
Reproduction or further distribution of this information may be subject to copyright protection. Use of the information without obtaining the permission from the owner(s) of the respective information might violate the rights of the owner.
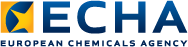