Registration Dossier
Registration Dossier
Data platform availability banner - registered substances factsheets
Please be aware that this old REACH registration data factsheet is no longer maintained; it remains frozen as of 19th May 2023.
The new ECHA CHEM database has been released by ECHA, and it now contains all REACH registration data. There are more details on the transition of ECHA's published data to ECHA CHEM here.
Diss Factsheets
Use of this information is subject to copyright laws and may require the permission of the owner of the information, as described in the ECHA Legal Notice.
EC number: 231-131-3 | CAS number: 7440-22-4
- Life Cycle description
- Uses advised against
- Endpoint summary
- Appearance / physical state / colour
- Melting point / freezing point
- Boiling point
- Density
- Particle size distribution (Granulometry)
- Vapour pressure
- Partition coefficient
- Water solubility
- Solubility in organic solvents / fat solubility
- Surface tension
- Flash point
- Auto flammability
- Flammability
- Explosiveness
- Oxidising properties
- Oxidation reduction potential
- Stability in organic solvents and identity of relevant degradation products
- Storage stability and reactivity towards container material
- Stability: thermal, sunlight, metals
- pH
- Dissociation constant
- Viscosity
- Additional physico-chemical information
- Additional physico-chemical properties of nanomaterials
- Nanomaterial agglomeration / aggregation
- Nanomaterial crystalline phase
- Nanomaterial crystallite and grain size
- Nanomaterial aspect ratio / shape
- Nanomaterial specific surface area
- Nanomaterial Zeta potential
- Nanomaterial surface chemistry
- Nanomaterial dustiness
- Nanomaterial porosity
- Nanomaterial pour density
- Nanomaterial photocatalytic activity
- Nanomaterial radical formation potential
- Nanomaterial catalytic activity
- Endpoint summary
- Stability
- Biodegradation
- Bioaccumulation
- Transport and distribution
- Environmental data
- Additional information on environmental fate and behaviour
- Ecotoxicological Summary
- Aquatic toxicity
- Endpoint summary
- Short-term toxicity to fish
- Long-term toxicity to fish
- Short-term toxicity to aquatic invertebrates
- Long-term toxicity to aquatic invertebrates
- Toxicity to aquatic algae and cyanobacteria
- Toxicity to aquatic plants other than algae
- Toxicity to microorganisms
- Endocrine disrupter testing in aquatic vertebrates – in vivo
- Toxicity to other aquatic organisms
- Sediment toxicity
- Terrestrial toxicity
- Biological effects monitoring
- Biotransformation and kinetics
- Additional ecotoxological information
- Toxicological Summary
- Toxicokinetics, metabolism and distribution
- Acute Toxicity
- Irritation / corrosion
- Sensitisation
- Repeated dose toxicity
- Genetic toxicity
- Carcinogenicity
- Toxicity to reproduction
- Specific investigations
- Exposure related observations in humans
- Toxic effects on livestock and pets
- Additional toxicological data

Bioaccumulation: aquatic / sediment
Administrative data
Link to relevant study record(s)
- Endpoint:
- bioaccumulation in aquatic species: fish
- Type of information:
- read-across from supporting substance (structural analogue or surrogate)
- Adequacy of study:
- key study
- Justification for type of information:
- Substance considered to fall within the scope of the read-across 'Silver metal: Justification of a read-across approach for environmental information requirements' (document attached in IUCLID section 13).
- Reason / purpose for cross-reference:
- read-across source
- Type:
- BCF
- Value:
- 70 dimensionless
- Basis:
- whole body w.w.
- Time of plateau:
- 30 d
- Calculation basis:
- steady state
- Remarks on result:
- other: Conc.in environment / dose:0.14-0.28 ppb
- Details on kinetic parameters:
- Steady state achieved after ~30d, exposure concentration approximately 0.14 to 0.28 ppb, BCF ~70 at steady state.
- Metabolites:
- No data reported
- Results with reference substance (positive control):
- Not applicable
- Details on results:
- The maximum 110mAg concentration factor was extrapolated to be 106 and would be reached after 180 days. The liver showed the highest 110mAg concentration factor at the end of the exposure phase.
- Reported statistics:
- No data reported
- Validity criteria fulfilled:
- not applicable
- Conclusions:
- The reported BCF at a steady state was ~70.
- Executive summary:
The reported BCF at a steady state was ~70. This study does not follow a standard guideline and only tests one concentration. However, the method is acceptable and therefore this study is considered suitable for use for this endpoint.
Reference
Description of key information
Read across from ionic silver
Plus supporting published data from several studies included in the REACH dossier as Endpoint Study Records with various sizes of nanoparticles and coating types, showing that fish would appear more susceptible to accumulation of ionic silver, rather than nanosilver
Key value for chemical safety assessment
Additional information
Summary of available data for uncoated and coated nanosilver
Bioaccumulation in fish
Data relevant to the bioaccumulation of uncoated and coated nanosilver are available from 12 studies. However, whilst none of these studies are consistent with standard assessments of bioaccumulation as outlined in REACH endpoint specific guidance, i.e. studies conducted according to the principles outlined in OECD guidance document 305 (exposure up to 28 days followed by 14 days depuration with measurement of whole body concentrations), the study by Griffitt et al. (2013) provides useful and relevant information for the comparative assessment of the relative bioaccumulation potential of ionic and nanosilver obtained from a study that most closely resembles a standard method.
Griffitt et al. (2013) undertook a comparative assessment of the accumulation of silver in zebra fish (Danio rerio), alongside concurrent effects on gene expression, after exposure to either ionic or nanosilver. Adult zebra fish were subject to a 32 day flow-through exposure (0-28 days with silver) to four concentrations of commercially obtained nanosilver (5, 15, 25 and 50 µg/L) and a single concentration of silver nitrate (5 µg/L). Each treatment comprised four replicates of 20 fish. Nanosilver was characterised in stock solutions to have a particle size of 3.1 ± 2.23 nm or 1.7 nm by TEM and DLS methods, respectively. Measured silver concentrations in exposure media stock solutions were 40-50 % of nominal concentrations. Accumulation of silver was measured in gills and carcass tissue at days 7, 14, 21 and 28. Mean BCF values (with 95% confidence intervals) for silver after exposure to nanosilver and ionic silver were calculated using data on individual carcass burden (µg/L wet weight) versus water concentrations presented in the paper. The mean BCF value for nanosilver was 6.61 (4.68 – 9.12), whilst the mean BCF value for ionic silver was 95.50 (66.07-138.04). BCF was significantly greater in ionic silver exposures than in nanosilver exposures (p<0.0001). The maximum BCF value reported for nanosilver based on an individual measurement of carcass burden versus water concentration was 46.82.
In addition, three studies (Scown et al. 2010, Gaiser et al. 2012, Schäfers and Weil 2013) contain sufficient information to calculate tissue-specific BCF values for silver nanomaterials, which can be used as “worst-case” surrogates for comparison with whole-body BCF data available for ionic silver.
Scown et al. (2012) investigated the fate and effects of three sizes of commercially available silver particles (including two nanoparticles: 10 and 35 nm) and ionic silver (as silver nitrate) using 10 day exposures with Oncorhynchus mykiss (rainbow trout). Uptake into the gills, liver and kidneys was quantified by inductively coupled plasma-optical emission spectrometry (ICP-OES). Maximum tissue-specific BCF values for nanomaterials in gills were 17.2 (10 nm) and 27.7 (35 nm), whilst maximum tissue-specific BCF values in liver were 26 (10 nm) and 42 (35 nm). Concentrations of silver in kidneys were below the analytical limit of detection, which precluded calculation of tissue-specific BCF values.
Gaiser et al. (2012) investigated the accumulation and effects of nanosilver and bulk silver (35 and 600–1,600 nm) in 21 day sub-chronic exposures with Cyprinus carpio. The authors report that an equivalent mass dose of silver nanoparticles was more toxic than larger micro-sized material. In addition, silver was found to accumulate in the liver, gallbladder and gills of carp exposed via the water. Greater accumulation was observed in fish exposed to 0.1 mg/L than 0.01 mg/L. Maximum tissue-specific BCF values for the 35 nm nanosilver particles were calculated as 6.18 and 2.92 for liver and gills, respectively. These measurements are based on total silver and no characterisation of silver nanoparticles in these tissues is reported. As described by Scown et al. (2010), silver was not detected above the analytical limit of detection in kidneys. Silver was also detected in the intestines of C. carpio, resulting in a maximum tissue-specific BCF value of 997. However, no depuration phase was reported in the study and it is not clear if intestine samples would include material present within the gut lumen, rather than accumulated material in mesentery. The former scenario would result in the calculation of greatly enhanced BCF values that are not consistent with true accumulation.
In an supplementary study to a conventional early life-stage toxicity test with nanosilver, Schäfers and Weil (2013) exposed juvenile zebra fish (Danio rerio) to 25 and 100 µg/L NM-300K nanosilver for 21 days (semi-static exposure regime with renewal of test dispersions on days seven and 14) and determined differential tissue uptake of silver in: 1) head, skin and gills; 2) intestines and stomach; and 3) remaining fish (fillet, organs, bones). Tissue-specific BCF values in head, skin and gills were 8.76 and 8.31 after exposure to 15.3 and 67.3 µg/L NM-300K, respectively. Tissue-specific BCF values in intestines and stomach were 327.0 and 302.0 after exposure to 15.3 and 67.3 µg/L NM-300K, respectively. Tissue-specific BCF values in the remainder of the fish were 7.58 and 6.17 after exposure to 15.3 and 67.3 µg/L NM-300K, respectively. The values for head, gills and skin and the remainder of the fish agree well with the BCF values for nanosilver calculated from the data presented in Griffitt et al. (2013). However, as observed in Gaiser et al. (2012), BCF values in intestines and stomach were higher than in other tissues. As discussed above, it is not clear if intestine samples would include material present within the gut lumen, rather than accumulated material in mesentery, which would confound BCF value calculation.
In addition, several additional studies describe the behaviour of nanosilver relevant to an assessment of bioaccumulation but which do not report BCF data or data from which either whole-body or tissue-specific BCF values can be calculated.
Kwok et al. (2012) report that hyperspectral imaging of Japanese medaka embryos exposed to nanosilver for 21 days revealed the presence of nanosilver and their aggregates in tissues of fish. Gill distribution was ubiquitous, while small amounts were also found in the liver and brain. No nanosilver particles were detected in/on the epidermis, spine, skeletal muscle, kidney or gonad of fish. Nanosilver particles were observed in the gut lumen but rarely in mural elements or mesentery. The authors conclude that gills were the principal sites of nanosilver uptake, although ingestion was common.
Farkas et al. (2011) report the results of a comparative assessment of the uptake of nanosilver and ionic silver in cultured rainbow trout (Oncorhynchus mykiss) gill cells. The largest amount of silver was found in gill cells exposed to ionic silver. However, citrate and PVP coated nanosilver were both observed to be taken up by gill cell monolayers, although uptake was significantly less for the slightly smaller PVP coated nanosilver particles. In contrast, once accumulated, PVP coated nanosilver particles were transported across cultured multilayers to a greater extent than citrate coated nanosilver particles and ionic silver, although the authors of the study conclude that additional work is required to confirm these observations.
Lee et al. (2012c/d) undertook studies of the real-time uptake of various sizes of nanosilver particles (41.6 nm and 11.6 nm average diameter) in early developing (cleavage-stage) zebrafish embryos. Single nanosilver particles were observed to passively diffuse into embryos through chorionic pores via random Brownian motion. Dose and size dependent effects on embryonic development were observed. Nanosilver particles were observed in eye (retina), brain, heart and tail. Deformed zebrafish were found to have accumulated higher numbers of larger particles than control fish. In addition, at the same molar concentrations, larger nanosilver particles (41.6 nm) were more toxic than the smaller nanosilver particles (11.6 nm).
Importantly, where silver is accumulated after exposure to nanosilver, the current fish studies are insufficient to distinguish if nanosilver particles are accumulated or if ionic silver has dissolved from a nanosilver particle, which is then accumulated.
With the exception of the BCF value calculated for intestines using the data presented by Gaiser et al. (2012) and Schäfers and Weil (2013), which may be an artefact of experimental design, none of the studies where BCF or tissue-specific BCF values can be calculated report BCF values for nanosilver in excess of the value of 70 used in the CSR for silver, which is based on a whole body BCF calculated after exposure to ionic silver (silver nitrate). Based on these data, fish would appear more susceptible to accumulation of ionic silver rather than nanosilver and an assessment of the bioaccumulative properties of nanosilver based on the properties of ionic silver would be protective.
Bioaccumulation in aquatic invertebrates
Whilst bioaccumulation in fish drives the assessment of bioaccumulation under REACH, several studies that describe the relative bioaccumulation potential of silver from ionic and nanosilver exposures in aquatic invertebrates are available and these data should be included in a comparative assessment of the hazard properties of nanosilver and ionic silver.
Croteau et al. (2011) compared silver bioavailability and toxicity in Lymnaea stagnalis (a freshwater gastropod) after exposure to ionic silver and to nanosilver particles coated with either citrate (17 ± 5 nm by TEM in test media) or humic acid (13 ± 3 nm by TEM in test media). Lymnaea stagnalis effectively accumulate silver from both ionic and nanoparticulate exposures via aqueous or dietary routes. However, for both routes, uptake rates were faster for ionic silver than for nanosilver particles. Whilst uptake was slower for nanosilver particles, loss rates of silver after waterborne exposure were also faster for nanosilver particles than dissolved silver. The authors conclude that ingestion of silver associated with particulate materials appears to be the most important vector of uptake.
In a related study, Khan et al. (2012) undertook waterborne exposures with the estuarine snail Peringia ulvae and concluded that dissolved silver is twice as bioavailable as nanosilver (citrate coated 16.5 ± 4.5 nm by TEM). Uptake rates in saline waters were slower than those reported for Lymnaea stagnalis by Croteau et al. (2011). Aggregation of nanosilver particles was observed to occur in estuarine (17 ppt salinity = 79 ± 13 nm) and marine (33 ppt salinity = 162 ± 21 nm) media. The authors hypothesise that aggregation of nanosilver particles reduces, but does not eliminate, the bioavailability of silver from nanosilver particles.
Buffet et al. 2013 investigated the biochemical and behavioural responses of the endobenthic bivalve Scrobicularia plana to nanosilver (40-45 nm diameter, obtained from JRC stablised in lactate) and ionic silver in seawater and microalgal food after a 14 day semi-static exposure. Measured bioaccumulation in the whole soft tissue after waterborne exposure to ionic silver (10 µg/L) was significantly higher (992 ± 382 ng/g ww) than in controls (483 ± 253 ng/g ww). However, there was no significant difference between bioaccumulation after exposure to 10 µg/L nanosilver (753 ± 467 ng/g ww) and the control. There was no significant difference between soft tissue concentrations of silver after dietary exposure to either ionic silver or nanosilver. Waterborne exposure to either form of silver did not affect feeding rate. Dietary exposure to either ionic silver or nanosilver resulted in reduced feeding rate after 10 days exposure.
Literature cited
Farkas J, Christian P, Gallegro-Urrea JA, Roos N, Hassellov M, Tollefsen KE, Thomas KV. 2011. Uptake and effects of manufactured silver nanoparticles in rainbow trout. Aquatic toxicology. 101: 117-125.
Lee KJ, Nallathamby PD, Browing LM, Osgood CJ, Xu, XN. 2007c. In vivo imaging of transport and biocompatibility of silver nanoparticles in early development of zebrafish embryos. ACS Nano. 1: 133-143.
Lee KJ, Browning LM, Nallathamby PD, Desai T, Cherukuri PK, Xu XN. 2012d. In vivo quantitative study of size-dependent transport and toxicity of single silver nanoparticles using zebrafish embryos. Chemical research in toxicology. 25: 1029-1046.
Information on Registered Substances comes from registration dossiers which have been assigned a registration number. The assignment of a registration number does however not guarantee that the information in the dossier is correct or that the dossier is compliant with Regulation (EC) No 1907/2006 (the REACH Regulation). This information has not been reviewed or verified by the Agency or any other authority. The content is subject to change without prior notice.
Reproduction or further distribution of this information may be subject to copyright protection. Use of the information without obtaining the permission from the owner(s) of the respective information might violate the rights of the owner.
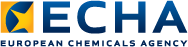