Registration Dossier
Registration Dossier
Data platform availability banner - registered substances factsheets
Please be aware that this old REACH registration data factsheet is no longer maintained; it remains frozen as of 19th May 2023.
The new ECHA CHEM database has been released by ECHA, and it now contains all REACH registration data. There are more details on the transition of ECHA's published data to ECHA CHEM here.
Diss Factsheets
Use of this information is subject to copyright laws and may require the permission of the owner of the information, as described in the ECHA Legal Notice.
EC number: 292-334-0 | CAS number: 90604-40-3
- Life Cycle description
- Uses advised against
- Endpoint summary
- Appearance / physical state / colour
- Melting point / freezing point
- Boiling point
- Density
- Particle size distribution (Granulometry)
- Vapour pressure
- Partition coefficient
- Water solubility
- Solubility in organic solvents / fat solubility
- Surface tension
- Flash point
- Auto flammability
- Flammability
- Explosiveness
- Oxidising properties
- Oxidation reduction potential
- Stability in organic solvents and identity of relevant degradation products
- Storage stability and reactivity towards container material
- Stability: thermal, sunlight, metals
- pH
- Dissociation constant
- Viscosity
- Additional physico-chemical information
- Additional physico-chemical properties of nanomaterials
- Nanomaterial agglomeration / aggregation
- Nanomaterial crystalline phase
- Nanomaterial crystallite and grain size
- Nanomaterial aspect ratio / shape
- Nanomaterial specific surface area
- Nanomaterial Zeta potential
- Nanomaterial surface chemistry
- Nanomaterial dustiness
- Nanomaterial porosity
- Nanomaterial pour density
- Nanomaterial photocatalytic activity
- Nanomaterial radical formation potential
- Nanomaterial catalytic activity
- Endpoint summary
- Stability
- Biodegradation
- Bioaccumulation
- Transport and distribution
- Environmental data
- Additional information on environmental fate and behaviour
- Ecotoxicological Summary
- Aquatic toxicity
- Endpoint summary
- Short-term toxicity to fish
- Long-term toxicity to fish
- Short-term toxicity to aquatic invertebrates
- Long-term toxicity to aquatic invertebrates
- Toxicity to aquatic algae and cyanobacteria
- Toxicity to aquatic plants other than algae
- Toxicity to microorganisms
- Endocrine disrupter testing in aquatic vertebrates – in vivo
- Toxicity to other aquatic organisms
- Sediment toxicity
- Terrestrial toxicity
- Biological effects monitoring
- Biotransformation and kinetics
- Additional ecotoxological information
- Toxicological Summary
- Toxicokinetics, metabolism and distribution
- Acute Toxicity
- Irritation / corrosion
- Sensitisation
- Repeated dose toxicity
- Genetic toxicity
- Carcinogenicity
- Toxicity to reproduction
- Specific investigations
- Exposure related observations in humans
- Toxic effects on livestock and pets
- Additional toxicological data

Biotransformation and kinetics
Administrative data
- Endpoint:
- biotransformation and kinetics
- Type of information:
- migrated information: read-across based on grouping of substances (category approach)
- Adequacy of study:
- supporting study
- Reliability:
- 2 (reliable with restrictions)
- Rationale for reliability incl. deficiencies:
- other: The referenced source presents reliable data which has been subject to peer-review and in which the original data sources are traceable.
Data source
Reference
- Reference Type:
- review article or handbook
- Title:
- Unnamed
- Year:
- 2 010
- Report date:
- 2010
Materials and methods
Results and discussion
Any other information on results incl. tables
Degradation by micro-organisms and algae
Alcohols in range C6 to C22 are readily biodegradable in reliable standard studies (P&G, 2009) supported by many other studies for C6-16. Other available studies for C18-22 do not replicate the ready biodegradability pass, a finding which may reflect issues associated with low solubility in the test system in the older studies. The P&G results demonstrate that even these alcohols are intrinsically susceptible to very rapid degradation by activated sludge micro-organisms. Available data on degradation in natural environments (P&G, pers. comm.) verify this, showing that degradation is rate-limited by physical factors of low water solubility and significant adsorption at higher chain lengths, but very rapidly degraded in solution.
These alcohols may be generated as a by-product in the degradation of hydrocarbons and waxes. Conversely the alcohols may also be produced under anoxic conditions by algae and sediment micro-organisms as a by-product of the metabolism of fatty acids (Mudge, 2008).
Linear aliphatic alcohols in the range C10-15 were found to be rapidly metabolised by algae present as a food source in chronic Daphnia reproduction tests (Schafers, 2005a-d). These tests were performed to a very high standard and ensured that the fate of substance was closely monitored. Some removal seen in later stages of the 21-day tests was attributed to bacterial degradation by microbes adsorbed onto the carapace of the test daphnids despite daily cleaning of the animals, but the algae were sterilised and the entire test system (test vessels and culture medium) was kept as sterile as could practically be achieved.
Metabolic pathways for degradation
The biochemical evidence for biotransformation has been reviewed in various literature sources. Both alcohol and aldehyde dehydrogenase enzymes (used in conversion to fatty acids) are ubiquitous in the plant and animal kingdoms but the location of the relevant enzyme system, the chemical specificity and the rate at which the reaction occurs differ between phyla and species.
Mudge (2008) discusses in some detail the metabolism of alcohols by microbes. Fatty alcohols are metabolised as part of the alkane hydroxylase/rubredoxin/rubredoxin reductase system by which alkanes are metabolised. The ß-cleavage steps reportedly occur within the cell, producing several molecules of ATP. Mudge reports evidence that alcohols in the range C10-20 have been demonstrated to be assimilated in bacterial chlorophyll-c.
The relevant metabolic pathways have been summarised as follows (Veenstra et al., 2009). The initial step in the mammalian metabolism of primary alcohols is oxidation to the corresponding carboxylic acid, with the corresponding aldehyde being a transient intermediate. These carboxylic acids are susceptible to further degradation via acyl-CoA intermediates by the mitochondrial ß-oxidation process. This mechanism removes C2 units in a stepwise process; transformation of linear acids is more efficient in this regard than the corresponding branched acids. An alternative pathway for metabolism of aliphatic acids that is particularly relevant for branched alkyl chains, exists through microsomal degradation via ¿ or ¿-1 oxidation followed by ß-oxidation (citing Verhoeven et al., 1998). Aliphatic acids produced from initial oxidation of longer-chained aliphatic alcohols may be incorporated into phospholipids and neutral lipids (citing Bandi et al., 1971 and Mukherjee et al., 1980).
Mankura (1987) reports that upon dietary force-feeding of radiolabelled (1-14C)-oleyl alcohol in carp (Cyprinus carpio) that the alcohol was largely oxidised to the acid in the intestinal tissue and incorporated into various forms. The analysis of distribution of radioactivity in this study reports the recovered radiolabelled tissue lipid form distribution for each of the major tissue types in the fish. After 72 h only 3.2% alcohol in total was recovered in the form of free fatty alcohol (total 3.2% across all tissues; total recovery across all tissue types and all incorporated tissue lipid forms 99.0%). Free fatty acid comprised 2.0% across all tissues. Among the tissue lipid forms the most significant appear to be the triglycerides (44.7%) and phosphatidylcholines (31.2%). The only tissue types in which radiolabelled free alcohol remained at significant levels (>10% of recovered radioactivity from the tissue sample) after 72 h are adipose tissue (free alcohol 16.2% in sample) and blood corpuscles (free alcohol 19.8%), though these comprise together only ca. 0.5% of the overall total radiolabelled residues. It is apparent that within 72 h of exposure the extent of biotransformation is extremely high in fish, and also that the accumulated parent alcohol is a very small proportion. The rate of in vivo metabolism demonstrated here, which is for a relatively lipophilic member of the category, is equivalent to a half-life of the order of hours.
An in vitro study of the biotransformation of linear and multiple-branched fatty alcohols (C12, C14) has been conducted (Menzel et al., 2001), with the intention to examine the pathway and relative rate of metabolism of linear compared to branched alcohol structures. The in vitro test system used either pig liver or fish (rainbow trout) liver homogenate as the source of the metabolic enzymes, and substance-specific GC analysis to follow the conversion of the alcohols into the corresponding fatty acids over a ten day period. The results demonstrated that in pig liver homogenate an intensive metabolism of the alcohols to the corresponding fatty acids takes place, with half-lives 3.8 d for C12, 6.7 d for C14 and 8 d for C12 branched alcohols. Photometric measurements of the esterase activity indicated that the fish liver homogenate was at least ca. ten times less active; this was ascribed to the overall lower metabolic activity of cold-blooded animals though the possible role of method of sample preparation is noted, though the exploration of these matters was not the objective of this study. The conclusion of this study was that linear C12 alcohol was degraded more rapidly than the C12 multiply-branched product, by a factor of ca. 2.5. This more rapid degradation of linear structures compared to multiply-branched structures is in line with the more complex metabolic pathway as discussed by Veenstra et al.
Applicant's summary and conclusion
Information on Registered Substances comes from registration dossiers which have been assigned a registration number. The assignment of a registration number does however not guarantee that the information in the dossier is correct or that the dossier is compliant with Regulation (EC) No 1907/2006 (the REACH Regulation). This information has not been reviewed or verified by the Agency or any other authority. The content is subject to change without prior notice.
Reproduction or further distribution of this information may be subject to copyright protection. Use of the information without obtaining the permission from the owner(s) of the respective information might violate the rights of the owner.
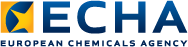