Registration Dossier
Registration Dossier
Data platform availability banner - registered substances factsheets
Please be aware that this old REACH registration data factsheet is no longer maintained; it remains frozen as of 19th May 2023.
The new ECHA CHEM database has been released by ECHA, and it now contains all REACH registration data. There are more details on the transition of ECHA's published data to ECHA CHEM here.
Diss Factsheets
Use of this information is subject to copyright laws and may require the permission of the owner of the information, as described in the ECHA Legal Notice.
EC number: 215-222-5 | CAS number: 1314-13-2
- Life Cycle description
- Uses advised against
- Endpoint summary
- Appearance / physical state / colour
- Melting point / freezing point
- Boiling point
- Density
- Particle size distribution (Granulometry)
- Vapour pressure
- Partition coefficient
- Water solubility
- Solubility in organic solvents / fat solubility
- Surface tension
- Flash point
- Auto flammability
- Flammability
- Explosiveness
- Oxidising properties
- Oxidation reduction potential
- Stability in organic solvents and identity of relevant degradation products
- Storage stability and reactivity towards container material
- Stability: thermal, sunlight, metals
- pH
- Dissociation constant
- Viscosity
- Additional physico-chemical information
- Additional physico-chemical properties of nanomaterials
- Nanomaterial agglomeration / aggregation
- Nanomaterial crystalline phase
- Nanomaterial crystallite and grain size
- Nanomaterial aspect ratio / shape
- Nanomaterial specific surface area
- Nanomaterial Zeta potential
- Nanomaterial surface chemistry
- Nanomaterial dustiness
- Nanomaterial porosity
- Nanomaterial pour density
- Nanomaterial photocatalytic activity
- Nanomaterial radical formation potential
- Nanomaterial catalytic activity
- Endpoint summary
- Stability
- Biodegradation
- Bioaccumulation
- Transport and distribution
- Environmental data
- Additional information on environmental fate and behaviour
- Ecotoxicological Summary
- Aquatic toxicity
- Endpoint summary
- Short-term toxicity to fish
- Long-term toxicity to fish
- Short-term toxicity to aquatic invertebrates
- Long-term toxicity to aquatic invertebrates
- Toxicity to aquatic algae and cyanobacteria
- Toxicity to aquatic plants other than algae
- Toxicity to microorganisms
- Endocrine disrupter testing in aquatic vertebrates – in vivo
- Toxicity to other aquatic organisms
- Sediment toxicity
- Terrestrial toxicity
- Biological effects monitoring
- Biotransformation and kinetics
- Additional ecotoxological information
- Toxicological Summary
- Toxicokinetics, metabolism and distribution
- Acute Toxicity
- Irritation / corrosion
- Sensitisation
- Repeated dose toxicity
- Genetic toxicity
- Carcinogenicity
- Toxicity to reproduction
- Specific investigations
- Exposure related observations in humans
- Toxic effects on livestock and pets
- Additional toxicological data

Endpoint summary
Administrative data
Key value for chemical safety assessment
Genetic toxicity in vitro
Link to relevant study records
- Endpoint:
- in vitro gene mutation study in bacteria
- Type of information:
- experimental study
- Adequacy of study:
- weight of evidence
- Study period:
- not specified
- Reliability:
- 2 (reliable with restrictions)
- Rationale for reliability incl. deficiencies:
- study well documented, meets generally accepted scientific principles, acceptable for assessment
- Qualifier:
- no guideline followed
- Principles of method if other than guideline:
- Li, C.-H. et al. (2011) investigated on the mutagenic potential of ZnO NPs using the bacterial reverse mutation assay. The ZnO NPs were tested, up to the limit dose, in Salmonella tester strains TA 98, TA 100, TA 102, TA 1535, and TA 1537 both in absence and presence of a metabolic activation system. The assay was performed according to the plate incorporation assay. Three independent experiments were performed.
- GLP compliance:
- not specified
- Remarks:
- publication
- Type of assay:
- bacterial reverse mutation assay
- Specific details on test material used for the study:
- Uncoated, ZnO-NPs, Top Nano Technology Co., Ltd. (Taipei, Taiwan)
- Target gene:
- histidine operon genes
- Species / strain / cell type:
- S. typhimurium TA 1535, TA 1537, TA 98, TA 100 and TA 102
- Metabolic activation:
- with and without
- Metabolic activation system:
- S9 fraction*
*References:
- Cheng YW, Lee WW, Li CH, Lee CC, Kang JJ. 2004. Genotoxicity of motorcycle exhaust particles in vivo and in vitro. Toxicol Sci 81:103–111.
- Maron DM, Ames BN. 1983. Revised methods for the Salmonella mutagenicity test. Mutat Res 113:173–215. - Test concentrations with justification for top dose:
- 0.312, 0.625, 1.25, 2.5, and 5.0 mg/plate
- Vehicle / solvent:
- - Vehicle used: sterile water
- Untreated negative controls:
- not specified
- Negative solvent / vehicle controls:
- yes
- True negative controls:
- not specified
- Positive controls:
- yes
- Positive control substance:
- other: 4-Nitro-o-phenylenediamine
- Remarks:
- Positive control in w/o S9 plate: TA98, 2.5 µg/plate
- Untreated negative controls:
- not specified
- Negative solvent / vehicle controls:
- yes
- True negative controls:
- not specified
- Positive controls:
- yes
- Positive control substance:
- sodium azide
- Remarks:
- Positive control in w/o S9 plate: TA100 and TA1535, 5 µg/plate
- Untreated negative controls:
- not specified
- Negative solvent / vehicle controls:
- yes
- True negative controls:
- not specified
- Positive controls:
- yes
- Positive control substance:
- mitomycin C
- Remarks:
- Positive control in w/o S9 plate: TA102, 0.5 µg/plate
- Untreated negative controls:
- not specified
- Negative solvent / vehicle controls:
- yes
- True negative controls:
- not specified
- Positive controls:
- yes
- Positive control substance:
- 9-aminoacridine
- Remarks:
- Positive control in w/o S9 plate: TA1537, 50 µg/plate.
- Untreated negative controls:
- not specified
- Negative solvent / vehicle controls:
- yes
- True negative controls:
- not specified
- Positive controls:
- yes
- Positive control substance:
- other: 2-aminoanthrene
- Remarks:
- Positive control in w/w S9 plate: TA100/TA102/TA1535/TA1537, 5 µg/plate.
- Untreated negative controls:
- not specified
- Negative solvent / vehicle controls:
- yes
- True negative controls:
- not specified
- Positive controls:
- yes
- Positive control substance:
- benzo(a)pyrene
- Remarks:
- Positive control in w/w S9 plate: TA98, 5 µg/plate
- Details on test system and experimental conditions:
- AMES TEST
Salmonella typhimurium histidine auxotrophs, TA98, TA100, TA1537, TA1535, and TA102 were purchased from Molecular Toxicology Inc. (Moltox, USA). Mutagenicity was determined by incorporation method with the presence and absence of S9 metabolic activation. A 100 mL of each test bacterial culture (10^9 cells/mL), 2 mL of soft agar (0.6% agar, 0.5% NaCl, 5 mM histidine, and 50 mM biotin, pH 7.4, 40~50°C), 0.5 mL S9 mixture (if necessary), and ZnO-NPs were mixed well in a test tube. Subsequently, the sample was immediately poured onto a minimal agar plate (1.5% agar, Vogel–Bonner E medium containing 2% glucose). Plates were incubated in an incubator for 48 h at 37°C in the dark. The revertant colonies were counted macroscopically. - Evaluation criteria:
- A compound was considered positive for mutagenicity only when i) the number of revertants was at least double the spontaneous yield, ii) a statistical significance (p ≤ 0.05) was found, and iii) a reproducible positive dose-response was present.
- Statistics:
- An one-way ANOVA was perfomred to test for statistical difference in revertant colony number between treatment and solvent control groups.
- Species / strain:
- S. typhimurium TA 1535
- Metabolic activation:
- with and without
- Vehicle controls validity:
- not specified
- Untreated negative controls validity:
- not specified
- True negative controls validity:
- not specified
- Positive controls validity:
- not specified
- Species / strain:
- S. typhimurium TA 1537
- Metabolic activation:
- with and without
- Vehicle controls validity:
- not specified
- Untreated negative controls validity:
- not specified
- True negative controls validity:
- not specified
- Positive controls validity:
- not specified
- Species / strain:
- S. typhimurium TA 98
- Metabolic activation:
- with and without
- Vehicle controls validity:
- not specified
- Untreated negative controls validity:
- not specified
- True negative controls validity:
- not specified
- Positive controls validity:
- not specified
- Species / strain:
- S. typhimurium TA 100
- Metabolic activation:
- with and without
- Vehicle controls validity:
- not specified
- Untreated negative controls validity:
- not specified
- True negative controls validity:
- not specified
- Positive controls validity:
- not specified
- Species / strain:
- S. typhimurium TA 102
- Metabolic activation:
- with and without
- Vehicle controls validity:
- not specified
- Untreated negative controls validity:
- not specified
- True negative controls validity:
- not specified
- Positive controls validity:
- not specified
- Additional information on results:
- AMES TEST
- No significant changes in the number of revertants were observed at any concentrations of ZnO-NPs treatments in the five Salmonella tester strains, when compared to solvent control (please refer to 'any other information on results incl. tables').
- The positive control substances induced statistically significantly and markedly increased revertant colony numbers, when compared to the solvent control values. - Remarks on result:
- not determinable because of methodological limitations
- Conclusions:
- Li, C.-H. et al. (2011) performed a Salmonella reverse mutation assay in order to evaluate the mutagenic potential of ZnO NPs . The assay was performed according to the plate incorporation method both in presence and absence of a metabolic activation system. Three independent experiments were performed. According to the authors, ZnO NPs induced a significant increase in the revertant colony number in any of the strains tested, when compared to the solvent control. The positive controls elicit a significant response as compared to the solvent controls.
The test material is insufficiently characterised, since information on purities and impurities is missing. Information on confounding factors, i.e. precipitation and cytotoxicity, in the main experiments is not provided. No confirmatory experiment was performed. Historical control data is missing.
Based on the above-mentioned shortcomings the reference is suitable for the weight of evidence analysis of the genetic toxicity of nano ZnO. - Endpoint:
- in vitro cytogenicity / chromosome aberration study in mammalian cells
- Remarks:
- Type of genotoxicity: chromosome aberration
- Type of information:
- experimental study
- Adequacy of study:
- weight of evidence
- Study period:
- From January 12, 2010 to June 30, 2010
- Reliability:
- 1 (reliable without restriction)
- Rationale for reliability incl. deficiencies:
- guideline study
- Qualifier:
- according to guideline
- Guideline:
- OECD Guideline 473 (In Vitro Mammalian Chromosome Aberration Test)
- Deviations:
- no
- GLP compliance:
- yes (incl. QA statement)
- Type of assay:
- in vitro mammalian chromosome aberration test
- Species / strain / cell type:
- Chinese hamster lung fibroblasts (V79)
- Additional strain / cell type characteristics:
- other: Doubling time 16 h
- Cytokinesis block (if used):
- Colcemid
- Metabolic activation:
- with and without
- Metabolic activation system:
- S9-mix
- Test concentrations with justification for top dose:
- Test substance: 0, 5, 10, and 20 µg/mL (4 h without S9-mix); 0, 1, 3, 10, and 15 µg/mL (24 h without S9-mix); 0, 12.5, 25, and 50 µg/mL (4 h with S9-mix)
Reference substance (Z-COTE® and micron-scaled ZnO): 1µg/mL (4 h without S9-mix), 12.5 µg/mL (4 h with S9-mix), and 3 µg/mL (24 h without 59-mix) - Vehicle / solvent:
- - Vehicle(s)/solvent(s) used: Phosphate buffer pH 7.4 with 100 µg/mL soy lecithin
- Untreated negative controls:
- yes
- Remarks:
- Growth medium
- Negative solvent / vehicle controls:
- yes
- Remarks:
- Phosphate buffer pH 7.4 with 100 µg/mL soy lecithin
- True negative controls:
- not specified
- Positive controls:
- yes
- Positive control substance:
- cyclophosphamide
- ethylmethanesulphonate
- Details on test system and experimental conditions:
- NUMBER OF REPLICATIONS:
- Number of cultures per concentration: triplicate
METHOD OF TREATMENT/ EXPOSURE:
- Cell density at seeding (if applicable): 10E6 per 25cm² flask
- Test substance added in medium
TREATMENT AND HARVEST SCHEDULE:
- Preincubation period, if applicable: 24 hres
- Exposure duration/duration of treatment: 4 hrs (+ and - S9) and 24 hrs (- S9)
- Harvest time after the end of treatment (sampling/recovery times): for the 4 hrs treatmentapprox. 1.5 cycle lengths recovery
FOR CHROMOSOME ABERRATION AND MICRONUCLEUS:
- Spindle inhibitor (cytogenetic assays): 2-3 hrs prior cell harvest 0.2mL colcemid stock solution (10 µg/mL) was added
- Methods of slide preparation and staining technique used including the stain used (for cytogenetic assays): 4slides per culture, faining with Giemsa (10% Giemsa freshly prepared)
- Number of cells spread and analysed per concentration (number of replicate cultures and total number of cells scored): 100 well spread metaphases with 22 +/- 2 centromeres/chromosomes were analysed per culture
- Criteria for scoring chromosome aberrations (selection of analysable cells and aberration identification): according to Buckton and Evans (1973), ISCN (1985), Savage (1976 and 1983)
METHODS FOR MEASUREMENT OF CYTOTOXICITY
- Method: mitotic index (MI)
- Any supplementary information relevant to cytotoxicity: 1000 cells were counted per concentration and number of metaphases recorded
METHODS FOR MEASUREMENTS OF GENOTOXICIY
- OTHER: - Evaluation criteria:
- - Gaps: lowest relevant aberrations
- Exchanges: Highest biologically relevant aberrations
- Pulverization or shattering: Toxic effect rather than clastogenic effect
- Positive result: Aberration (including gaps) in > 5% of the analyzed cells in each of the two technical replicates at one dose level
- Dose dependency may provide further evidence to clastogenicity
- Negative result: Only gaps in single test group without dose relation - Species / strain:
- Chinese hamster lung fibroblasts (V79)
- Metabolic activation:
- with and without
- Genotoxicity:
- negative
- Cytotoxicity / choice of top concentrations:
- cytotoxicity
- Remarks:
- Dose dependent without S9-mix and only at highest dose with S9-mix
- Vehicle controls validity:
- valid
- Untreated negative controls validity:
- valid
- Positive controls validity:
- valid
- Additional information on results:
- TEST-SPECIFIC CONFOUNDING FACTORS
- Effects of pH: No changes
- Effects of osmolality: No changes
- Evaporation from medium: Test substance is solid
- Water solubility: Insoluble
RANGE-FINDING/SCREENING STUDIES: Dose finding as pre-test measuring cytotoxicity
COMPARISON WITH HISTORICAL CONTROL DATA: Concurrent controls were in line with historical controls - Conclusions:
- An in vitro mammalian chromosome aberration test was performed with V79 cells to assess the potential of uncoated nanoscaled ZnO to induce structural (and numerical) chromosome aberrations in somatic mammalian cells according to the OECD guideline 473 in compliance with GLP.
V79 Chinese hamster lung fibroblast cells were exposed to the test substance for 4 h both in the absence and presence of metabolic activation system. An initial experiment on cytotoxicity was conducted to determine the concentrations of the test substance to be used in main experiment. Uncoated ZnO nanoparticlesone concentration per main experiment was used, with 10 µg/mL for the short term experiment without S9-mix, 12.5 µg/mL for the short term experiment with S9-mix, and 3.0 µg/mL for the long term experiment without S9-mix, respectively.
There was a decrease in M.I. for uncoated nanoscaled ZnO, primarily without S9-mix. Test performance and activity of the metabolizing system were satisfactory. Irrespective of its cytotoxic potential in the absence of S9-mix,uncoated nanoscaled ZnO, under all treatment modalities, did not increase significantly the aberration frequency, as compared to the respective vehicle controls. Besides some gaps, which were also evident in the negative and vehicle controls, a sum of only 4 chromosome breaks was observed in all 3 main experiments. The aberration frequencies (without gaps) in no case exceeded 5 % and they all fell within the range of the historical negative controls.
Under the test conditions, nanoscaled uncoated ZnO is considered not to induce structural chromosome aberrations in cultured mammalian somatic cells. - Endpoint:
- in vitro gene mutation study in mammalian cells
- Remarks:
- Type of genotoxicity: gene mutation
- Type of information:
- experimental study
- Adequacy of study:
- weight of evidence
- Reliability:
- 1 (reliable without restriction)
- Rationale for reliability incl. deficiencies:
- guideline study
- Qualifier:
- according to guideline
- Guideline:
- OECD Guideline 476 (In Vitro Mammalian Cell Gene Mutation Test)
- GLP compliance:
- yes (incl. QA statement)
- Type of assay:
- mammalian cell gene mutation assay
- Target gene:
- thymidine kinase (TK)
- Species / strain / cell type:
- mouse lymphoma L5178Y cells
- Details on mammalian cell type (if applicable):
- - Periodically checked for Mycoplasma contamination: yes
- Additional strain / cell type characteristics:
- not applicable
- Metabolic activation:
- with and without
- Metabolic activation system:
- S9-mix
- Test concentrations with justification for top dose:
- 1, 2, 4, 5, and 6 µg/ml (without S9-mix)
2.5, 5, 7.5, and 10 µg/ml (with S9-mix) - Vehicle / solvent:
- phosphate buffer + 100 µg/ml soy lecithin (1 part) and RPMI-1640 medium + 5% horse serum (9 parts)
- Untreated negative controls:
- yes
- Remarks:
- RPMI-1640 medium + 5% horse serum
- Negative solvent / vehicle controls:
- yes
- Remarks:
- phosphate buffer + 100 µg/ml soy lecithin (1 part) and RPMI-1640 medium + 5% horse serum (9 parts)
- True negative controls:
- not specified
- Positive controls:
- yes
- Positive control substance:
- methylmethanesulfonate
- Remarks:
- cyclophosphamide as positive control with S9-mix Migrated to IUCLID6: positive control without S9-mix
- Details on test system and experimental conditions:
- METHOD OF APPLICATION: in medium
DURATION
- Preincubation period: no preinubation performed
- Exposure duration: 4 hours
- Expression time (cells in growth medium): 2 days
- Selection time (if incubation with a selection agent): 14 -16 days
SELECTION AGENT (mutation assays): 5-fluorothymidine
NUMBER OF CELLS EVALUATED: 2,000 cells/well
DETERMINATION OF CYTOTOXICITY
- Method: relative total growth - Evaluation criteria:
- criteria for positive results:
- concentration-related or reproducible increase in mutant frequency (MF)
- biological relevance of the results is considered firs
- increase in MF occuring only at highly toxic concentrations of the test item (i. e. less than 10% of total growth) is not considered biologically relevant
- relevant increase in present study is stated if MF of test item amounted to more than [(MF of positive/vehicle control) + 125], 125 represents the historical control data for this type of method
- a test item is considered to be mutagenic if both criteria (relevant increase and dose-dependency) are met
- if only one criteria is met the test item is reported as equivocal
- in case of no relevant increase and no dose-dependency the test item is considered to be non-mutagenic in the present test system - Species / strain:
- mouse lymphoma L5178Y cells
- Metabolic activation:
- with and without
- Genotoxicity:
- ambiguous
- Remarks:
- relevant increase in mutant frequency always linked to cytotoxicity
- Cytotoxicity / choice of top concentrations:
- cytotoxicity
- Vehicle controls validity:
- valid
- Untreated negative controls validity:
- valid
- Positive controls validity:
- valid
- Additional information on results:
- TEST-SPECIFIC CONFOUNDING FACTORS
- Effects of pH: slight reduction of medium's pH directly after preparation but not after 4 hours of incubation
- Effects of osmolality: physiological range directly after preparation, lower physiologigal range after 4 hours of incubation
- Evaporation from medium: test item is solid
- Water solubility: insoluble
- Precipitation: turbidity did not change during 4 hours of incubation indicating stable treatment suspensions, slightly increased turbidity noted for Z-COTE HP1 at 10µg/ml (7.81 FAU), for Z-COTE at 7.5 µg/ml (8.31 FAU = formazine attenuation units) as well as microscaled ZnO (11.84 FAU) compared to negative and vehicle control (5.29 and 6.3 FAU). 50 µg/ml Z-COTE HP1 markedly increased turbidity (70.72 FAU).
RANGE-FINDING/SCREENING STUDIES: dose range study performed as pre-test, concentration of main test based on results of pre-test
ADDITIONAL INFORMATION ON CYTOTOXICITY: - Remarks on result:
- other: all strains/cell types tested
- Remarks:
- Migrated from field 'Test system'.
- Conclusions:
- Mouse lymphoma L5178Y/ TK±cells in suspension culture were treated for 4 hours with different concentrations of the test item with and without S9 -mix. Medium, medium with soy lecithine, methyl methanesulfonate and cyclophosphamide were used as negative control, vehicle control, positive control without S9 -mix and positive control with S9 -mix, respectively. The gene mutation potential of the test item Z-COTE HP1 (coated nanoscaled ZnO) was determined.
Z-COTE HP1 induced increases of MF with and without S9 -mix with a concentration-dependency in the presence of S9 -mix, which was significantly elevanted almost exclusively at the highest concentration. The increase mutant colonies were primarly characterised as small colonies, indicating a clastogenic event.
The significantly increased MF was always linked to pronounced cytotoxicity. For this reason and the limited significance of the test system for particles, the test results should more likely be judged as questionable in L5178Y/TK cells under the conditions and restrictions of this assay, implying a possible false positive result.
Consequently, the results are interpreted as equivocal, as the clastogenic findings were confounded with cytotoxicity.
Referenceopen allclose all
Table 1.Mutagenicity assay of ZnO NPs and ZnO MPs.
|
Without S9 metabolic activation |
With S9 metabolic activation |
||||||||
|
TA98 |
TA100 |
TA102 |
TA1535 |
TA1537 |
TA98 |
TA100 |
TA102 |
TA1535 |
TA1537 |
Negative |
27±3 |
113±9 |
124±10 |
17±1 |
6±1 |
35±2 |
113±6 |
256±4 |
20±2 |
7±1 |
Positive |
419±87* |
901±105* |
1825±139* |
904±57* |
685±142* |
240±8* |
549±50* |
1021±81* |
656±38* |
462±44* |
ZnO-NPs (mg/plate) |
||||||||||
0.312 |
24±3 |
171±6 |
175±13 |
23±2 |
10±1 |
41±5 |
115±4 |
272±2 |
26±3 |
13±3 |
0.625 |
27±5 |
181±4 |
186±16 |
22±3 |
12±2 |
43±4 |
133±31 |
260±23 |
17±1 |
13±1 |
1.25 |
34±1 |
168±18 |
148±7 |
23±3 |
6±1 |
43±4 |
157±22 |
285±6 |
22±1 |
12±2 |
2.5 |
33±1 |
161±16 |
144±6 |
30±1 |
6±1 |
39±3 |
154±17 |
264±21 |
24±1 |
11±1 |
5.0 |
26±1 |
188±10 |
134±12 |
26±5 |
10±0 |
44±3 |
179±21 |
261±5 |
23±1 |
14±3 |
Values were presented as Mean±S.D.(N³3). Solvent (Sterile water) was used as negative control. Positive control in w/o S9 plate: TA98, 4-Nitro-o-phenylenediamine 2.5 µg/plate; TA100 and TA1535, Sodium azide 5 µg/plate; TA102, Mitomycin C 0.5 µg/plate; TA1537, 9 -Aminoacridine 50 µg/plate. Positive control in w/w S9 plate: TA98, benzo(a)pyrene 5 µg/plate; TA100/TA102/TA1535/TA1537, 2-aminoanthrene 5 µg/plate.Statistical analysis: *p< 0.05, **p< 0.01, and ***p< 0.001, indicate a statistical difference with the control groupby One-way ANOVA.
Detail on results:
Estimation of cytotoxicity (pre-experiments): Cytotoxicity of the test substance and the particulate reference substances was evaluated in pre-experiments without S9-mix, using four concentrations for the test substance (4 h: 10, 30, 40, 50 µg/mL; 24 h: 5, 10, 20, 40 µg/mL) and also four concentrations for the particulate reference substances (4 h: 10, 30, 40, 50 µg/mL; 24 h: 1, 5, 10, 20 µg/mL). Using the M.I. as a measure for cell viability/cytotoxicity, there was a more or less concentration-dependent decrease in cell viability after 4 h of incubation with the test substance in the absence of S9-mix. At the highest concentration (50 µg/mL) M.I. was reduced to 50.4 % of the negative control and 52.0 % of the vehicle control, respectively. In contrast, the vehicle was nearly nontoxic, as compared to the negative control. For the main experiments the maximum concentration of test substance was reduced to 25 µg/mL to ensure both an appropriate high cytotoxicity of the maximum concentration (at least 50 % reduction in viability), but also enough metaphases for analysis of chromosome aberrations. In contrast to test substance, there was profound concentration-dependent cytotoxicity after 4 h of incubation for the particulate reference substances. At 40 and 50 µg/mL, M.I. was markedly reduced to 0.8 and 0.0 % of the negative control for uncoated nanoscaled ZnO and to 7.7 and 4.7% of the negative control for micron-scaled ZnO, respectively. Thus, it was decided to use no more than 10 µg/mL of the particulate reference substance as maximum concentration in the short term main experiment without S9-mix. As expected, cytotoxicity of the test substance was more intense after 24 h of incubation without S9-mix than after 4 h of incubation, perhaps due to potentially increased particle-like effects or test substance dissolution. M.I., at the highest concentration used (40 µg/mL), amounted to only 5.8 %, as compared to the negative control. The vehicle was, again, only marginally cytotoxic. To ensure a sufficient number of metaphases for chromosome analysis, but also to reach an appropriate high level of cytotoxicity for the highest concentration in the main experiment, 15 µg/mL of the test substance were chosen as the maximum concentration. In the long term pre-experiment, uncoated nanoscaled ZnO was highly cytotoxic. Already at a concentration as low as 1 µg/mL, M.I. was reduced to 5.8 % of the negative control. In this preexperiment, micron-scaled ZnO was less cytotoxicity up to a concentration of 10 µg/mL, but there was complete loss of metaphases at 20 µg/mL. As cytotoxicity of the uncoated ZnOs after 24 h of incubation was more pronounced than that of the test substance, it was decided to use 3 µg/mL as exposure concentration for the particulate reference substances, and not the highest concentration chosen for test substance (15 µg/mL), to enable comparison of the different particles.
Main experiments:
-Osmolality and pH: To estimate unphysiological changes of the cell culture environment due to addition of the test and particulate reference substances, osmolality and pH of the test substance and particulate reference substance-containing incubation media without S9-mix were evaluated at the highest concentrations, used for the main experiments. Concerning osmolality, there was a slight decrease from 342 mOSMOL/kg for the negative control medium to 309 mOSMOL/kg for the vehicle control medium (negative control medium + phosphate buffer with soy lecithin) after 4 h of incubation, and from 345 mOSMOL/kg to 311 mOSMOL/kg after 24 h of incubation, respectively. Osmolality of test substance containing incubation medium (50 µg/mL) was in the same range as the vehicle control medium with 307 mOSMOL/kg after 4 h of incubation. Osmolality of the particulate reference substance (12.5 µg/mL) both amounted to 309 mOSMOL/kg, indicating no test- or reference substance-mediated changes in osmolality. Only after 24 h of incubation, osmolality of the test substance (15 µg/mL) with 336 mOSMOL/kg exceeded that of the vehicle control value, but, as compared to the 4 h values, seemed to represent a false positive result. For the particulate reference substance (3 µg/mL) osmolality resembled that of the vehicle control. All osmolalities were still in the physiological range. Concerning pH, the vehicle, the test substance, and the particulate reference substance uncoated nanoscaled ZnO and micron-scaled ZnO did not significantly change the pH of the incubation media, both after 4 and 24 h of incubation. There was only a very slight tendency towards an increase in pH in the particle-containing incubation media after 4 h. However, after 24 h of incubation no such increase was observed.
-Mitotic index (M.I.):
Short term treatment without S9-mix: Cells were treated for 4 h with different concentrations of the test substance (5, 10, 20, and 25 µg/mL) and one concentration (10 µg/mL) of the reference substance without S9-mix. M.I. was subsequently evaluated for both of the duplicate cultures to estimate cytotoxicity. As compared to the negative controls, the vehicle controls exhibited slight reduction in the number of metaphases, with a reduction of the mean M.1. to 84.5 % of the negative controls. Concerning test substance, there was a clear, concentration-dependent cytotoxic effect of the test substance. At the highest concentration used (25 µg/mL) mean M.I. amounted to 2.20 (=24.0 % of the negative control, M.1. = 9.25), as compared to 7.8 for the vehicle control. The reference substance uncoated nanoscaled and micron-scaled ZnO at 10 µg/mL exhibited mean MI of 4.1 (=44.5% of the negative control) and 5.05 (=54.5% of the negative control), respectively, with a higher mean MI of 5.95 (= 64.5% of the negative control) for test substance at the same concentration.
Short term treatment with S9-mix: Cells were treated for 4 h with different concentrations of the test substance (12.5, 25, 40, and 50 µg/mL) and one concentration (12.5 µg/mL) of the reference substance in the presence of S9-mix. The M.I. was evaluated for both of the duplicate cultures to estimate cytotoxicity. Interestingly, in contrast to the short treatment without S9-mix and the respective pre-experiment on cytotoxicity, there was no reduction in viability due to the vehicle exposure and less cytotoxic action of the test substance. Only at the highest Z-COTE® HP1 concentration (50 µg/mL) mean M.I. was significantly reduced to 4.95 (= 44.5 % of the negative control), as compared to 11 .45 102.5 % of the negative control) for the vehicle control. The reference substances at 12.5 µg/mL each mediated only slight reduction in mean M.I., with Z-COTE® treated cultures exhibiting a mean M.I. of 9.8 (= 88.0% of the negative control) and micron-scaled ZnO treated cultures exhibiting a mean M.I. of 10.5 94.5% of the negative control). Interestingly, at 12.5 µg/mL Z-COTE® HP1 rather increased than decreased viability of the V79 cells.
Long term treatment without S9-mix: Cells were treated for 24 h with five different concentrations of the test substance (1, 3, 10, 15 and 30 µg/mL) and one concentration (3 µg/mL) of the reference substances Z-COTE® and micron-scaled ZnO in the absence of S9-mix. The M.I. was evaluated for both of the duplicate cultures to estimate influence of the test and reference substance on cell viability. After 24 h of incubation, Z-COTE® HP1 concentration-dependently decreased cell viability with a clear reduction in mean M.I. to 3.35 (= 46 % of the negative control) at 15 µg/mL, as compared to 6.9 (= 78.5 % of the negative control) for the vehicle control. At 30 µg/mL of the test substance no metaphases were present. After 24 h of incubation, the vehicle (phosphate buffer and soy lecithin) mediated a slight decrease in cell viability with a mean M.I. of 6.9, as compared to 8.75 for the negative control. This was in line with the marginal cytotoxicity of the vehicle in the pre-experiment. The reference substances Z-COTE® and micron-scaled ZnO (both 3 µg/mL) mediated slightly more reduction in viability than the test substance. Mean M.I. amounted to 5.9 (= 68.0 % of the negative control) for Z-COTE® and 6.0 (= 69% of the negative control) for micron-scaled ZnO, as compared to 7.45 (= 86% of the negative control) for the test substance Z-COTE® HP1 at the same concentration.
Chromosome analysis: Negative and vehicle controls exhibited spontaneous aberration frequencies within the normal range for V79 cells. As positive controls, cells treated with EMS (without S9-mix) or CP (with S9-mix), were analyzed for structural (and numerical) chromosome aberrations. The positive control cultures demonstrated that the system was operating satisfactorily. The marked increase in aberrations indicated that the target cells were very sensitive to the effect of known clastogens and that the used metabolizing system (S9-mix) was quite efficient. The chromosome aberrations observed in the positive control cultures consisted of: g, G, b (ctb, ccmin), B (f, nud, dmin, su), ex (qr, tr, ctr, cte, cUnv), EX (ex, csr, die), and mao For historical positive control data.
Short treatment without S9-mix: V79 cells, treated for 4 h in the absence of S9-mix with 5, 10, or 20 µg/mL of the test substance Z-COTE® HP1, or with 10 µg/mL of the reference substances Z-COTE® or micron-scaled ZnO, were analyzed for occurrence of chromosome aberrations. There was no evidence of a concentration-dependent induction of structural chromosome aberrations by treatment with Z-COTE® HP1, with the exception of chromatid gaps (g = ctg) at all concentrations analyzed, one chromosome gap (G = csg, at 20 µg/mL), and three B (f, 1 in each concentration). Chromatid gaps (g =ctg) were also observed in the negative and vehicle control cultures and one isochromatid gap (G =csg) was found in one of the negative control cultures. Aberration frequencies (gaps excluded) in the negative and vehicle control cultures amounted to 0.0 %, whereas the Z-COTE® HP1-treated cultures exhibited aberration frequencies (gaps excluded) of 0.5 % for all concentrations tested. The frequency of polyploid cells (py) was not significantly increased by Z-COTE® HP1, as compared to the vehicle control. Only chromatid (g = ctg) and isochromatid gaps (G = csg) were noted in the cultures treated with 10 µg/mL of the particulate reference substance. The particulate reference substances only demonstrated some gaps, which were also observed in the negative and vehicle control cultures.
Short treatment with S9-mix: V79 cells, treated for 4 h in the presence of S9-mix with 12.5, 25, and 50 µg/mL of the test substance Z-COTE® HP1 or 12.5 µg/mL of the particulate reference substances Z-COTE® or micron-scaled ZnO, were analyzed for the occurrence of chromosome aberrations. The respective negative and vehicle control cultures exhibited three (negative control) and four chromatid gaps (g =ctg, vehicle control), respectively. Concerning the Z-COTE® HP1treated cultures, there was evidence of six chromatid gaps (G =ctg) and one isochromatid gap (G =csg) (12.5 µg/mL), three chromatid gaps (g = ctg) and one isochromatid gap (G = csg) (25 µg/mL), and six chromatid gaps (g = ctg) and one isochromatid gap (G =csg) (50 [µg/mL). The aberration frequencies (gaps excluded) for all Z-COTE® HP1 concentrations tested as well as for the negative and vehicle control cultures both amounted to 0.0%. There was also no significant increase in the frequencies of polyploid cells (py) in Z-COTE® HP1-treated cultures, as compared to the vehicle controls. The particulate reference substances only demonstrated chromatid (g = ctg) and isochromatid gaps (G == csg), which were also observed in the negative and vehicle control cultures, and very slight elevation in polyploidy metaphases.
Long term treatment without S9-mix: V79 cell cultures, treated for 24 h without S9-mix with 1, 3, 10, and 15 µg/mL Z-COTE® HP1 or 3 µg/mL of the particulate reference substances Z-COTE® and micron-scaled ZnO, were analyzed for the occurrence of chromosome aberrations. The negative and vehicle control cultures only demonstrated occurrence of chromatid gaps (g = ctg) and one chromosome gap (G = csg, vehicle control). In the Z-COTE® HP1-treated cultures, only one chromatid gap (g = ctg) and one isochromatid gap (G = csg) (1 µg/mL), two chromatid gaps (g = ctg) (3 µg/mL, two chromatid gaps (g = ctg) (10 µg/mL), and six chromatid gaps (g = ctg), two isochromatid gaps (G = csg), and one chromosome break (B = 1 dmin) (15 µg/mL) were noted. The aberration frequencies (% cells with aberrations, gaps excluded) of the test substance-treated cells thus amounted to 0.0 % (1, 3, and 10 µg/mL) and 0.5 % (15 µg/mL), respectively. The aberration frequencies of the negative and vehicle control cultures both amounted to 0.0 %. The frequency of polyploid cells (py) in the test substance-treated cultures resembled that of the negative and vehicle control cultures for 1 - 10 µg/mL and was slightly elevated to six polyploidy metaphases, as compared to the negative and vehicle control cultures, which exhibited both one py. Like for the test substance, the particulate reference substances only demonstrated chromatid (g =ctg) and isochromatid gaps (G = csg), which were also observed in the negative and vehicle control cultures.
Reference items |
4 h / -S9-mix |
4 h / +S9-mix |
24 h / -S9-mix |
||||||
%AC |
|
%AC |
|
%AC |
|
||||
-g |
+g |
%M |
-g |
+g |
%M |
-g |
+g |
%M |
|
Negative control |
2.0 |
0.0 |
100 |
1.5 |
0.0 |
100 |
1.0 |
0.0 |
100 |
Vehicle control |
2.0 |
0.0 |
84.3 |
2.0 |
0.0 |
102.7 |
2.0 |
0.0 |
78.9 |
EMS 150 µg/ml |
55.5 |
36.5 |
45.9 |
- |
- |
- |
- |
- |
- |
EMS 50 µg/ml |
- |
- |
- |
- |
- |
- |
79.5 |
70.5 |
25.7 |
CP 2.5 µg/ml |
- |
- |
- |
45.0 |
31.5 |
98.7 |
- |
- |
- |
Z-COTE 3 µg/ml |
- |
- |
- |
- |
- |
- |
3.5 |
0.0 |
67.4 |
Z-COTE 10 µg/ml |
1.0 |
0.0 |
44.3 |
- |
- |
- |
- |
- |
- |
Z-COTE 12.5 µg/ml |
- |
- |
- |
2.0 |
0.0 |
87.9 |
- |
- |
- |
ZnO 3 µg/ml |
- |
- |
- |
- |
- |
- |
1.5 |
0.0 |
68.6 |
ZnO 10 µg/ml |
3.0 |
0.0 |
54.6 |
- |
- |
- |
- |
- |
- |
ZnO 12.5 µg/ml |
- |
- |
- |
3.0 |
0.0 |
94.6 |
- |
- |
- |
Z-COTE HP1 (µg/ml) |
|
||||||||
1 |
- |
- |
- |
- |
- |
- |
1.0 |
0.0 |
102.3 |
3 |
- |
- |
- |
- |
- |
- |
1.0 |
0.0 |
85.1 |
5 |
3.5 |
0.5 |
83.8 |
- |
- |
- |
- |
- |
- |
10 |
4.0 |
0.5 |
64.3 |
- |
- |
- |
1.0 |
0.0 |
72.6 |
12.5 |
- |
- |
- |
3.5 |
0.0 |
104.5 |
- |
- |
- |
15 |
- |
- |
- |
- |
- |
- |
4.5 |
0.5 |
38.3 |
20 |
4.0 |
0.5 |
47.0 |
- |
- |
- |
- |
- |
- |
25 |
- |
- |
- |
2.0 |
0.0 |
97.8 |
- |
- |
- |
50 |
- |
- |
- |
3.5 |
0.0 |
44.4 |
- |
- |
- |
%AC: % aberrationt cells; %M: M.I. in percent of the negative control (mean of two technical replicates); +g: with gaps; -g: without gaps; -: not tested
After 4 hours of incubation the highest Z-COTE HP1 concentration (10 µg/ml) did not alter pH and osmolarity of the treatment media but induced concentration-dependent cytotoxicity with and without S9 -mix. Vehicle, negative, and positive control exhibited mutant frequencies (MF) within the normal range. Based on the evaluation criteria of the present study Z-COTE HP1 induced relevant increases (marked with * in the following tables) of the MF in both replicates at 6 µg/ml without S9 -mix and 7.5 and 10 µg/ml with S9 -mix, and in one replicate at 5 µg/ml without S9 -mix. However, significantly increased MF was always linked to acute cytotoxicity. In the presence of S9 -mix relevant increases in MF were also obvious for the particulate reference items at 7.5 µg/ml. All the other particle-treated cultures exhibited MFs which were within the normal range for negative control.
4 h without S9-mix |
||
Treatment |
Relative total growth |
Mutant frequency |
Vehicle control |
1.00 |
90.3 |
Negative control |
0.93 |
105.7 |
Z-COTE®HP1: 1 µg/ml |
0.81 |
124.8 |
Z-COTE® HP1: 2 µg/ml |
0.81 |
108.3 |
Z-COTE® HP1: 4 µg/ml |
0.77 |
115.7 |
Z-COTE® HP1: 5 µg/ml |
0.62 |
181.1* |
Z-COTE® HP1: 6 µg/ml |
0.21 |
982.1* |
Z-COTE® : 4 µg/ml |
0.79 |
128.3 |
ZnO microscaled: 4 µg/ml |
0.82 |
149.4 |
Positive control (methyl methansulfonate 10 µg/ml) |
0.43 |
697.9* |
4 h with S9-mix |
||
Treatment |
Relative total growth |
Mutant frequency |
Vehicle control |
1.00 |
110.3 |
Negative control |
1.85 |
117.2 |
Z-COTE®HP1: 2.5 µg/ml |
1.31 |
123.1 |
Z-COTE® HP1: 5.0 µg/ml |
1.24 |
139.6 |
Z-COTE® HP1: 7.5 µg/ml |
0.50 |
463.1* |
Z-COTE® HP1: 10.0 µg/ml |
0.36 |
574.3* |
Z-COTE® : 7.5 µg/ml |
0.43 |
537.9* |
ZnO microscaled: 7.5 µg/ml |
0.52 |
433.3* |
Positive control (cyclophosphamide 2.5 µg/ml) |
1.49 |
468.7* |
*: relevant increase regarding evaluation criteria of the present study method
Endpoint conclusion
- Endpoint conclusion:
- no adverse effect observed (negative)
Genetic toxicity in vivo
Link to relevant study records
- Endpoint:
- in vivo mammalian somatic cell study: cytogenicity / erythrocyte micronucleus
- Type of information:
- experimental study
- Adequacy of study:
- weight of evidence
- Study period:
- 2009-10-05 to 2013-12-19
- Reliability:
- 1 (reliable without restriction)
- Rationale for reliability incl. deficiencies:
- guideline study
- Reason / purpose for cross-reference:
- reference to same study
- Qualifier:
- according to guideline
- Guideline:
- OECD Guideline 474 (Mammalian Erythrocyte Micronucleus Test)
- Version / remarks:
- adopted on July 21, 1997
- Deviations:
- no
- GLP compliance:
- yes (incl. QA statement)
- Remarks:
- GLP certificate signed on 2011-08-15
- Type of assay:
- mammalian erythrocyte micronucleus test
- Species:
- rat
- Strain:
- other: Crl:WU
- Details on species / strain selection:
- Wistar rats are commonly used in subchronic and chronic inhalation toxicity studies. They fulfil the criteria stated by a U.S. EPA Workshop (Vu et al., 1996)* such as (i) a low background rate of neoplasia, (ii) a low background rate of pulmonary disease, (iii) longevity, and (iv) a history of laboratory use. In this study the specified Wistar strain is preferred to the Fischer strain because young Fischer rats available in Germany sporadically show a slight latent inflammation of lungs which might interfere with the scheduled examinations.
*References:
- Vu, V., Barrett, J.C, Roycroft, J., Schuman, L., Dankovic, D. Workshop report: Chronic inhalation toxicity and carcinogenicity testing of respirable fibrous particles. Reg Tox Pharm 24, 202-212 (1996) - Sex:
- male/female
- Details on test animals or test system and environmental conditions:
- TEST ANIMALS
- Source: Charles River Deutschland (Sulzfeld/Germany)
- Age at study initiation: approx. 8 weeks
- Weight at study initiation: approx. 230 g (males), approx. 165 g (females)
- Assigned to test groups randomly: yes, under following basis: randomized and grouped by the PROVANTIS system on body weight basis
- Fasting period before study: No
- Housing: 2 rats per cage, absorbing softwood bedding
- Diet: Ssniff "V1534" (ssniff Spezialdiäten GmbH; Soest, Germany); ad libitum
- Water: tap water; ad libitum
- Acclimation period: 1 d followed by 3 weeks of training in nose-only tubes without exposure
ENVIRONMENTAL CONDITIONS
- Temperature: 22 ± 2°C
- Humidity: 55 ± 15%
- Air changes (per hr): Fully airconditioned
- Photoperiod: 12 hrs dark / 12 hrs light - Route of administration:
- inhalation: aerosol
- Vehicle:
- - Vehicle(s)/solvent(s) used: clean air
- Details on exposure:
- TYPE OF INHALATION EXPOSURE: nose only
GENERATION OF TEST ATMOSPHERE CHAMBER DESCRIPTION
- Exposure apparatus: Flow-past nose-only exposure system, individually exposure of each rat, exhaled air is immediately exhausted
- Method of holding animals in test chamber: Individual acrylic tubes
- Source and rate of air: Pressurized air, 1 L/min
- System of generating particulates/aerosols: Feeding system and high-pressure, high-velocity pressurized air dispersion with computerized control
- Temperature, humidity, pressure in air chamber: 22 ± 2°C, 55 ± 15%,
- Air flow rate: 1 L/min
- Method of particle size determination: Cascade impactor/ Marple impactor
- Treatment of exhaust air: Disposal in compliance with local, federal and state regulations
TEST ATMOSPHERE
- Brief description of analytical method used: Gravimetrically by filter samples, feed back loop to actual aerosol concentrations measured by an aerosol photometer
- Samples taken from breathing zone: Yes - Duration of treatment / exposure:
- 14 days (10 days exposure)
- Frequency of treatment:
- 5 consecutive days per week, 6 h per day
- Post exposure period:
- 1 day
- Dose / conc.:
- 7.93 mg/m³ air (analytical)
- Remarks:
- SD: ± 1.98 mg/m³ (gravimetric analysis); 8.02 ± 1.11 mg/m³ (photometer); target concentration: 8 mg/m³
- No. of animals per sex per dose:
- 5 male and 5 female rats per dose
- Control animals:
- yes, concurrent no treatment
- Positive control(s):
- cyclophosphamide (CP) monohydrate
- Route of administration: oral
- Doses / concentrations: single dose of 20 mg/kg (dissolved in tap water)
- Group size: 5 rats - Tissues and cell types examined:
- bone marrow tissue - polychromatic erythrocytes (PCE)
- Details of tissue and slide preparation:
- From suspensions of bone marrow tissue, cleaned of nucleated cells by a cellulose column procedure, smears were prepared on slides, fixed for 10 minutes in absolute methanol and stained with May-Grünwald- and Giemsa-solution. The slides were analyzed microscopically under 630-1000 x magnification. Microscopic analysis was conducted on a blind basis. Micronuclei were counted in 2000 polychromatic erythrocytes (PCE) per animal. The ratio of polychromatic to normochromatic erythrocytes (NCE) was determined in 500 red blood cells.
- Evaluation criteria:
- The micronucleus assay is judged as valid if the clean air controls demonstrate low spontaneous frequencies of micronucleus induction and if the positive controls demonstrate significantly higher frequencies of micronucleus induction as compared to the clean air controls. In addition, in test item treated animals the PCE ratio should not fall below 20% of the clean air control values to avoid unspecific effects due to excessive cytotoxicity in the bone marrow.
In the mammalian erythrocyte micronucleus test a genotoxic effect is claimed if a dose-related increase in the number of polychromatic erythrocytes or a statistically significant increase in the number of micronucleated cells in a single dose group at a single sampling time is observed, but biological relevance of the results is considered first. - Statistics:
- Differences between groups were considered statistically significant at p < 0.05. Data were analyzed using analysis of variance. If the group means differ significantly by the analysis of variance the means of the treated groups were compared with the means of the control groups using Dunnett's test.
The statistical evaluation of the histopathological findings was done with the two-tailed Fisher test by the P.L.A.C.E.S. system. - Sex:
- male/female
- Genotoxicity:
- negative
- Toxicity:
- no effects
- Vehicle controls validity:
- valid
- Negative controls validity:
- not examined
- Positive controls validity:
- valid
- Additional information on results:
- MN ASSAY
- Under the conditions of this assay, uncoated Z-COTE did not significantly enhance the number of micronuclei in polychromatic erythrocytes of the bone marrow (after 14 days of inhalation exposure) of both male and female Wistar rats (please refer to the field ‘any other information on results incl. tables’).
- The positive control CP significantly induced micronucleus formation in PCE of the bone marrow, with higher rates in male than in female animals.
- As assessed by differential cell counting of bone marrow smears, uncoated Z-COTE in the given dose (8.0 mg/m³) did not significantly influence red blood cell formation in male Wistar rats after 14 days of inhalation exposure. However, there was a high fluctuation rate in PCE numbers, in part, perhaps based on the combination of the micronucleus test with the hOGG1-modified comet assay ex vivo in the male animals. In female Wistars rats, number of PCE/500 RBC was significantly reduced to 143±23.0 (p ≤0.05) by uncoated Z‑COTE and to 124±23.1 (p ≤0.01) by the positive control CP, as compared to 179±18.0 for the clean air control.
TOXICITY
- General toxicity: Signs of general toxicity were not evident.
- Local effects: A NOAEL of 2 mg/m³ was derived based on a strong acute response characterised by cell infiltration and enzymatic response as well as histopathological alteration characterised by bronchiolo-alveolar hyperplasia and mononuclear infiltration. - Conclusions:
- A 14-day repeated dose inhalation toxicity study (Creutzenberg, 2013) was conducted with uncoated Z-COTE in rats exposed subacutely via nose-only exposure according to the OECD Guideline 412 in compliance with GLP. In the framework of this study also systemic clastogenic and aneugenic effects were investigated in male and female rats using the bone marrow micronucleus assay according to OECD Guideline 474. Five rats per sex and group were exposed at a concentration level of 7.93 mg/m³ with Z-COTE HP1. Fresh air treated animals served as concurrent control. Positive control animals were orally exposed to 20 mg/kg cyclophosphamide monohydrate.
There was no treatment-related reduction of body weights in any test group; no clinical signs were detected. However, high dose rats showed adverse local effects in the lungs. In male rats, the test item and the reference substances did not mediate significant repression of red blood cell formation. In females, the PCE/NCE was significantly reduced in the positive control as well as in females exposed to 8 mg/m³ uncoated Z-COTE. This effect might indicate that these test items reached the bone marrow as target organ. However, toxicokinetic data obtained in parallel showed the test material to be not available in the blood stream. There was no evidence of a significantly enhanced mean frequency of micronucleated erythrocytes due to Z‑COTE HP1 exposure in males or females, as compared to the vehicle control groups (clean air) at any dose level. The positive and vehicle controls gave valid results.
Inhaled Z-COTE is considered non-mutagenic in immature bone marrow erythrocytes (PCE) of Wistar rats under the conditions tested. - Endpoint:
- in vivo mammalian cell study: DNA damage and/or repair
- Type of information:
- experimental study
- Adequacy of study:
- weight of evidence
- Study period:
- 2009-10-05 to 2013-12-19
- Reliability:
- 2 (reliable with restrictions)
- Rationale for reliability incl. deficiencies:
- study well documented, meets generally accepted scientific principles, acceptable for assessment
- Remarks:
- The study presented herein is considered to be acceptable with restrictions. The experiment included in the study were not performed under GLP conditions, which was basically due to the fact that an OECD validated test guideline was not available at that time. The tail intensity was determined only using a single slide per animal; replicates were not included. Furthermore, the responses observed showed in several cases a huge variability. Thus, the robustness of the data may be limited. Moreover, the description of the methodology lacks detailed information on cell lysis, neutralisation, and electrophoresis. The positive controls were run only in an independent experiment, concurrent positive control animals were not included. Cytotoxicity was not sufficiently investigated and evaluated. The only parameter useful for an evaluation of cytotoxicity is the LDH activity level in BAL fluid. Evaluation criteria are not specified. Historical control data are not included. Only one dose level was tested, which precludes an evaluation of dose-response relationships.
- Reason / purpose for cross-reference:
- reference to same study
- Qualifier:
- no guideline followed
- Principles of method if other than guideline:
- A 14-day repeated dose inhalation toxicity study (Creutzenberg, 2013) was conducted to establish exposure dose-response relationships of the Z-COTE in rats after subacute nose-only exposure according to the OECD Guideline 412 in compliance with GLP. In the framework of this study, potential site of contact genotoxicity was evaluated as well.
- GLP compliance:
- no
- Type of assay:
- mammalian comet assay
- Species:
- rat
- Strain:
- other: Crl:WU
- Details on species / strain selection:
- Wistar rats are commonly used in subchronic and chronic inhalation toxicity studies. They fulfil the criteria stated by a U.S. EPA Workshop (Vu et al., 1996)* such as (i) a low background rate of neoplasia, (ii) a low background rate of pulmonary disease, (iii) longevity, and (iv) a history of laboratory use. In this study the specified Wistar strain is preferred to the Fischer strain because young Fischer rats available in Germany sporadically show a slight latent inflammation of lungs which might interfere with the scheduled examinations.
*References:
- Vu, V., Barrett, J.C, Roycroft, J., Schuman, L., Dankovic, D. Workshop report: Chronic inhalation toxicity and carcinogenicity testing of respirable fibrous particles. Reg Tox Pharm 24, 202-212 (1996) - Sex:
- male
- Details on test animals or test system and environmental conditions:
- TEST ANIMALS
- Source: Charles River Deutschland (Sulzfeld/Germany)
- Age at study initiation: approx. 8 weeks
- Weight at study initiation: approx. 230 g (males), approx. 165 g (females)
- Assigned to test groups randomly: yes, under following basis: randomized and grouped by the PROVANTIS system on body weight basis
- Fasting period before study: No
- Housing: 2 rats per cage, absorbing softwood bedding
- Diet: Ssniff "V1534" (ssniff Spezialdiäten GmbH; Soest, Germany); ad libitum
- Water: tap water; ad libitum
- Acclimation period: 1 d followed by 3 weeks of training in nose-only tubes without exposure
ENVIRONMENTAL CONDITIONS
- Temperature: 22 ± 2°C
- Humidity: 55 ± 15%
- Air changes: Fully airconditioned
- Photoperiod: 12 hrs dark / 12 hrs light - Route of administration:
- inhalation: aerosol
- Vehicle:
- - Vehicle(s)/solvent(s) used: clean air
- Details on exposure:
- TYPE OF INHALATION EXPOSURE: nose only
GENERATION OF TEST ATMOSPHERE CHAMBER DESCRIPTION
- Exposure apparatus: Flow-past nose-only exposure system, individually exposure of each rat, exhaled air is immediately exhausted
- Method of holding animals in test chamber: Individual acrylic tubes
- Source and rate of air: Pressurized air, 1 L/min
- System of generating particulates/aerosols: Feeding system and high-pressure, high-velocity pressurized air dispersion with computerized control
- Temperature, humidity, pressure in air chamber: 22 ± 2°C, 55 ± 15%,
- Air flow rate: 1 L/min
- Method of particle size determination: Cascade impactor/ Marple impactor
- Treatment of exhaust air: Disposal in compliance with local, federal and state regulations
TEST ATMOSPHERE
- Brief description of analytical method used: Gravimetrically by filter samples, feed back loop to actual aerosol concentrations measured by an aerosol photometer
- Samples taken from breathing zone: Yes - Duration of treatment / exposure:
- 14 days (10 days exposure)
- Frequency of treatment:
- 5 consecutive days per week, 6 h per day
- Post exposure period:
- 1 and 14 days
- Dose / conc.:
- 8.24 mg/m³ air (analytical)
- Remarks:
- SD: 1.27 mg/m³ (gravimetric analysis); 8.04 ± 0.75 mg/m³ (photometer); target concentration: 8 mg/m³
- No. of animals per sex per dose:
- 5 male rats per dose
- Control animals:
- yes, concurrent vehicle
- Positive control(s):
- Potassium bromate-treated L5178Y/TK+/- mouse lymphoma cells served as a technical positive control to assess both activity of the used hOGG1 enzyme batch and appropriate performance of slide preparation, electrophoresis, and staining.
- Tissues and cell types examined:
- BAL (comet assay) and lung epithelial (immunohistochemistry) cells
- Details of tissue and slide preparation:
- COMET ASSAY
DNA-strand breaks and oxidative DNA damage (8-OH-dG) were analysed in BAL cells of 5 males per group on day 1 and day 14 post exposure using the hOGG1-modified comet assay under non-GLP conditions.
BAL cell suspensions of male rats treated with clean air or the test and particulate reference items served as a new model system. Preparation time points for the comet assay were limited to two time points, 24 h after the last exposure (day 1 post exposure) and 14 days after the last exposure.
Two aliquots of each BAL cell suspension (a minimum of 20.000 cells in a volume of 1 mL) per animal of clean air or test item-exposed animals were centrifuged, resuspended in pre-heated 0.75% low melting agarose, applied to agarose pre-coated slides, and lysed overnight at 4°C to liberate the DNA. Based on the limited cell number, only two slides per animal could be generated. One of the two slides per animal was subsequently incubated for 10 min with 0.16 U/mL of hOGG1. The other slide only received enzyme buffer. In both cases, DNA-unwinding and electrophoresis was done on ice in 4°C cold electrophoresis buffer (pH > 13). DNA was finally stained with ethidium bromide and analysed using the Comet Assay III Software (Perceptive Instruments, UK). As the main end point tail intensity (proportion of DNA in tail) (tail length and tail moment determinations were included as supplemental information) of 100 nuclei per animal and slide treatment (with or without hOGG1 incubation) was determined (in total, 500 nuclei per group and slide treatment). An increase in TI (TL, TM) on the hOGG1-treated slides, as compared to the slides treated with enzyme buffer only, is indicative for the occurrence of the oxidative base lesion 8-OHdG.
Percent heavily damaged nuclei on the comet assay slides, both without and with hOGG1 incubation, were used as a surrogate endpoint for the cell integrity of the isolated BAL cells. Nuclei with a TI of ≥ 90 % were defined as “heavily damaged”. The percentage of “heavily damaged nuclei” was retrospectively calculated from the number of nuclei with a TI ≥ 90 % within the fraction of 100 nuclei, evaluated per animal. These nuclei were then retrospectively excluded from statistical analysis. Hedgehogs, which could not be scored by the image-analysis system, were a priori excluded from analysis. This is a generally agreed approach for evaluation of comet assay slides.
The ability of the hOGG1-modified comet assay to detect oxidative DNA-damage in BAL cells in vivo was independently confirmed in the same rat strain (not part of this study) by i.p. injection of 250 mg/kg bw KBrO3, with isolation of BAL cells 3 h after application.
IMMUNOHISTOCHEMICAL DETECTION OF OXIDATIVE DNA DAMAGE
Immunohistochemical detection of 8-OH-dG in lung tissue as marker of oxidative DNA damage was performed on samples prepared for histopathology (cf. section 7.5.2: Creutzenberg, 2013). Formalin-fixed tissue of the terminal bronchioles and lung parenchymal cells were examined for the formation of 8-OH-dG by an antibody labelling technique. - Evaluation criteria:
- not specified
- Statistics:
- The median derived mean TI was used for statistical analysis (mean derived TI was included as supplemental data). The Mann-Whitney Rank Sum Test was performed to compare the treatment groups with the concurrent negative controls. To compare the hOGG1-untreated slides with the hOGG1-treated slides, the paired t-test was used, as the tested samples were directly linked to each other and represent a “before and after” situation. The results obtained in the immunohistochemical analysis of 8-OH-dG positive nuclei were tested for statistical significance using the Tukey's studentised range test. Differences between groups/slides were considered statistically significant at p ≤ 0.05.
- Sex:
- male
- Genotoxicity:
- negative
- Toxicity:
- yes
- Vehicle controls validity:
- valid
- Negative controls validity:
- not examined
- Positive controls validity:
- valid
- Additional information on results:
- COMET ASSAY
- The exposure to Z-COTE did not result in statistically significantly increased median derived mean tail intensities, both with and without hOGG1 treatment, in BAL cells examined 1 and 14 days post exposure (please refer to table 1 in the field ‘any other information on results incl. tables’).
- The technical positive control cultures showed a statistically significant increase in the tail intensity after hOGG1 treatment, when compared to cells without hOGG1 treatment. Thus, the activity and the appropriate performance of the comet assay were demonstrated. Moreover, the in vivo positive control rats, tested in an independent experiment, showed a clear and statistically significant increase in the tail intensity with and without hOGG1 treatment, when compared to negative controls. Thus, the general sensitivity with regards to general and oxidative DNA damage was demonstrated.
- The number of heavily damaged (≥ 90%) nuclei was in general low and in most cases didn’t correlate with the respective TI values of the preparation.
- Hedgehogs were only sporadically observed.
- In an independent experiment performed in 2020, the positive reference for general DNA damage, EMS, induced a marked increase in the tail intensity, when compared to concurrent negative controls (please refer to table 3 in the field ‘any other information on results incl. tables’)
IMMUNOHISTOCHEMICAL DETECTION OF OXIDATIVE DNA DAMAGE
- At post exposure days 1 and 14, the number of 8-OH-dG positive nuclei per area was not statistically significantly increased in Z-COTE exposed animals, when compared to the vehicle control group (please refer to table 2 in the field ‘any other information on results incl. tables’).
TOXICITY
- General toxicity: Signs of general toxicity were not evident.
- Local effects: A strong acute response characterised by cell infiltration and enzymatic response as well as histopathological alteration characterised by bronchiolo-alveolar hyperplasia and mononuclear infiltration were observed after exposure to Z-COTE.
- Notably, 24 hours post exposure, the LDH activity and the total protein level in the BAL fluid were markedly and statistically significantly increased after exposure to Z-COTE, indicating cytotoxicity. - Conclusions:
- Creutzenberg (2013) investigated on the potential site-of-contact genotoxicity of Z-COTE in male Wistar rats after repeated nose-only inhalation. Five rats were exposed to Z-COTE aerosol at a concentration level of 8.24 mg/m³ for 5 days/week and 6 hours/day over a total period of two weeks. Vehicle control (clean air) rats were run concurrently. At post exposure days 1 and 14, BAL cells were obtained and tested for DNA damage using the modified alkaline comet assay. In this type of comet assay, lysed cells were either treated with buffer or with hOGG1 enzyme to detect general and oxidative DNA damage. A total of 100 cells per animal were scored for the tail intensity (% DNA in tail). As technical positive control served potassium bromate-treated L5178Y/TK+/- mouse lymphoma cells. Moreover, in an independent experiment, rats exposed to potassium bromate (250 mg/kg bw) via intraperitoneal injection served as in vivo positive controls. Apart from the comet assay, the authors examined the formation of 8-OH-dG in formalin-fixed tissue of the terminal bronchioles and lung parenchymal cells via immunohistochemistry.
Wistar rats exposed to Z-COTE did not show signs of general toxicity. However, the local adverse effects in the lung were evident in the highest dose group. Notably, the LDH activity in BAL fluid was statistically significantly increased in animals exposed to 8 mg/m³ indicating cytotoxic effects after 24 hours post exposure.
The BAL cells of rats exposed to Z-COTE HP1 did not show a statistically significant increase in the tail intensity after 1 and 14 days of the final treatment, when compared to the concurrent vehicle control. Moreover, the hOGG1 treatment did not reveal an increase of oxidative DNA damage. In contrast, the independent in vivo positive controls showed marked and statistically significantly both with and without hOGG1 treatment, when compared negative controls. Furthermore, independently generated positive control data from rats exposed to EMS showed a marked increase in the tail intensity, when compared to concurrent negative controls, in a conventional alkaline comet without hOGG1 treatment. Thus, the general sensitivity of the test system was demonstrated. Moreover, the technical positive control demonstrated the activity of the used hOGG1 enzyme and the appropriate performance of the comet assay methodology.
In addition, terminal bronchioles and lung parenchymal cells from Z-COTE exposed rats did not show statistically significantly increased numbers of 8-OH-dG positive nuclei, when compared to the vehicle control values. Thus, the lack of Z-COTE-induced oxidative DNA damage observed in the comet assay was further substantiated by the data obtained in the immunohistochemical assay using lung sections.
The study presented herein is considered to be acceptable with restrictions.
The experiment included in the study were not performed under GLP conditions, which was basically due to the fact that an OECD validated test guideline was not available at that time. The tail intensity was determined only using a single slide per animal; replicates were not included. Furthermore, the responses observed showed in several cases a huge variability. Thus, the robustness of the data may be limited. Moreover, the description of the methodology lacks detailed information on cell lysis, neutralisation, and electrophoresis. The positive controls were run only in an independent experiment, concurrent positive control animals were not included. Cytotoxicity was not sufficiently investigated and evaluated. The only parameter useful for an evaluation of cytotoxicity is the LDH activity and total protein level in BAL fluid. Evaluation criteria are not specified. Historical control data are not included. Only one dose level was tested, which precludes an evaluation of dose-response relationships.
The test material, Z-COTE, is considered to have no DNA damaging potential based on the absence of biological relevant increases in the median derived mean tail intensity under the conditions tested. - Endpoint:
- in vivo mammalian cell study: DNA damage and/or repair
- Type of information:
- experimental study
- Adequacy of study:
- key study
- Study period:
- 17 December 2021 to June 2022
- Reliability:
- 1 (reliable without restriction)
- Rationale for reliability incl. deficiencies:
- guideline study
- Remarks:
- The study presented herein is a guideline study without restrictions performed under GLP conditions.
- Reason / purpose for cross-reference:
- reference to same study
- Qualifier:
- according to guideline
- Guideline:
- OECD Guideline 489 (In vivo Mammalian Alkaline Comet Assay)
- Version / remarks:
- adopted 29 July 2016
- GLP compliance:
- yes (incl. QA statement)
- Type of assay:
- mammalian comet assay
- Species:
- rat
- Strain:
- Wistar
- Details on species / strain selection:
- Wistar rats, Crl:WI(Han)
Rats were selected since this rodent species is recommended in the respective test guidelines. Wistar rats were selected since there is extensive experience available in the laboratory with this strain of rats. - Sex:
- male
- Details on test animals or test system and environmental conditions:
- TEST ANIMALS
- Source: Charles River Deutschland (Sulzfeld/Germany)
- Age at study initiation: approx. 7 weeks
- Weight at study initiation: The weight variation of the animals used did not exceed ± 20 percent of the mean weight of each sex.
- Assigned to test groups randomly: yes: All animals were randomized before the start of the pre-exposure period (according to weight).
- Fasting period before study: No
- Housing: 5 rats per cage, Typ 2000P ca. 2065 cm² (polysulfone cages) supplied by TECNIPLAST, Germany. Dust-free wooden bedding
- Diet: milled/ mouse and rat maintenance diet, GLP, 12 mm pellets, Granovit AG, Kaiseraugst, Switzerland; ad libitum
- Water: tap water; ad libitum
- Acclimation period: ± 2 weeks
ENVIRONMENTAL CONDITIONS
- Temperature: 22-24°C
- Humidity: 45-65%
- Air changes: 15 air changes per hour
- Photoperiod: 12 hrs dark / 12 hrs light - Route of administration:
- inhalation: aerosol
- Vehicle:
- - Vehicle(s)/solvent(s) used: clean air
- Details on exposure:
- TYPE OF INHALATION EXPOSURE: whole-body
GENERATION OF TEST ATMOSPHERE / CHAMBER DESCRIPTION
- Exposure apparatus: Generation of the inhalation atmospheres via a solid particle generators (brush-generator; BASF SE, Ludwigshafen, Germany) & Aerosol mixing tube (stainless steel; BASF SE, Ludwigshafen, Germany). Whole body exposure systems were used. The animals were kept singly in wire cages located in a glass steel inhalation chamber, volume of 1.1 m³ or 1.4 m³(BASF SE).
- Method of holding animals in test chamber: Whole body exposure systems. The animals were kept singly in wire cages located in a glass steel inhalation chamber, volume of 1.1 m³ or 1.4 m³(BASF SE). The chambers were located in exhaust hoods in an air conditioned room.
- Source and rate of air: Conditioned air from the central air conditioning system, compressed and exhaust air. Compressed air was produced by an oil-free compressor (HT 6, Josef Mehrer GmbH & Co KG, Germany). For this purpose, air is filtered by an inlet air strainer and introduced into the compressor. After passing through an second ultra filter (SMF 5/3, 108 mm, Donalson), the compressed air (15 bar) is stored in a storage of 1500 or 5000 L. The compressed air is conducted to the laboratories via pipes, where the pressure is reduced to 5 - 6 bar. In the laboratory, the compressed air can be taken as required.
- Method of conditioning air: Conditioned air from the central air conditioning system provides cold air of about 15°C. This cold air passes through an activated charcoal filter, is adjusted to room temperature of 20 to 24°C and passes through a second particle filter (H13 (HEPA) Camfil Farr, Germany). The so generated conditioned air was used to generate inhalation atmospheres.
- System of generating particulates/aerosols: The particles/aerosol was generated via a solid particle generator (brush-generator; BASF SE, Ludwigshafen, Germany) and an aerosol mixing tube (stainless steel; BASF SE, Ludwigshafen, Germany), according to the following method: For each concentration the dust aerosol was generated with the dust generator and compressed air inside a mixing stage; mixed with conditioned dilution air and passed into the inhalation system.
- Temperature, humidity, pressure in air chamber: Daily mean relative humidities in the inhalation systems ranged between 40.7 and 50.8 %. Daily mean temperatures in the inhalation systems ranged between 20.4 and 23.0°C. They are within the range suggested by the respective testing guidelines.
- Air flow rate: The air flows were constantly maintained in the desired range.
- Air change rate: An air change of about 20 times per hour can be calculated by dividing the supply air flow through the volume of each inhalation system.
- Method of particle size determination: The particle size analysis was carried out with a cascade impactor. Equipment for particle size analysis: Stack sampler Marple 298 (New Star Environmental, Inc., Roswell, Georgia 30075, USA) ; Vacuum compressed air pump (Millipore Corporation, Billerica, MA 01821, USA) ; Limiting orifice 3 L/min (Millipore Corporation, Billerica, MA 01821, USA) ; Sampling probe internal diameter 6.9 mm ; Balance Sartorius MSA 6.6S-000-DF (Sartorius AG, Göttingen, Germany). The calculation of the particle size distribution was carried out in the Laboratory for Inhalation Toxicology of the Experimental Toxicology and Ecology of BASF SE on the basis of mathematical methods for evaluating particle measurements (OECD guidance document No. 39).
To determine the particle size distribution in the submicrometer range, each test atmosphere was measured with the Scanning Mobility Particle Sizer (SMPS; Grimm Aerosol Technik GmbH & Co KG, Ainring, Germany). The SMPS system comprises an Electrostatic Classifier (Model Vienna U-DMA) which separates the particles into known size fractions, and a Condensation Particle Counter (CPC) which measures particle count concentrations. The DMA was equipped with Am-241 neutralizer. The sampling duration was about 7 minutes. As a rule 10 repeats were measured for each exposure concentration.
- Treatment of exhaust air: Exhaust air was filtered and conducted into the exhaust air of the building.
TEST ATMOSPHERE
- Brief description of analytical method used: The concentrations of the inhalation atmospheres were determined by gravimetrical measurements of filter samples in all test groups. Control group was not sampled. This analytical method was judged to be valid because the test substances did not possess an appreciable vapor pressure.
- Samples taken from breathing zone: yes - Duration of treatment / exposure:
- 14 days
- Frequency of treatment:
- 14 days, 6 h per day
- Dose / conc.:
- 0 mg/m³ air
- Remarks:
- Test Group 0 - air control
- Dose / conc.:
- 0.54 mg/m³ air (analytical)
- Remarks:
- SD: ± 0.06 mg/m³; target concentration: 0.5 mg/m³ : Test group 1
- Dose / conc.:
- 2.07 mg/m³ air (analytical)
- Remarks:
- SD: ± 0.15 mg/m³; target concentration: 2 mg/m³ : Test group 2
- Dose / conc.:
- 7.89 mg/m³ air (analytical)
- Remarks:
- SD: ± 0.53 mg/m³ ; target concentration: 8 mg/m³: Test group 3
- No. of animals per sex per dose:
- 5 male rats per dose
- Control animals:
- yes, concurrent vehicle
- Positive control(s):
- ethylmethanesulphonate
- Justification for choice of positive control(s):
- Route of administration: oral gavage which guarantees systemic distribution of the compound and thus exposure to all assessed tissues
- Doses / concentrations: 300 mg/kg body weight - Tissues and cell types examined:
- Comet assay:
1. Bone marrow
2. Liver
3. Lung
4. Nasal mucosa
Bronchoalveolar lavage fluid (BAL): for cytology and total protein and enzyme levels. - Details of tissue and slide preparation:
- COMET ASSAY
Samples were minced or aspirated in cold mincing buffer to produce a cell suspension. Cell suspensions were diluted as necessary and kept cold until they were processed further. At least three comet slides were prepared per sample. An aliquot of cell suspension was mixed with low melting point agarose, layered onto microscope slides precoated with normal melting point agarose, and covered with an additional layer of low melting point agarose. After the agarose had solidified, slides were lysed in cold working high salt lysing solution and maintained cold for at least 1 hour. At least two comet slides were removed from lysis for electrophoresis. Slides were rinsed with 0.4M Tris buffer and submerged in alkaline electrophoresis buffer for 20 minutes at 1 to 10°C to unwind the DNA. After unwinding, slides were electrophoresed in the same buffer at 1 to 10°C for 40 minutes at a constant voltage of 0.7V/cm. The buffer level was adjusted as necessary at the start of electrophoresis to achieve a starting current of 300±10 mA. After electrophoresis, the slides were neutralized with 0.4M Tris buffer, rinsed in ethanol, and air dried. The air-dried comet slides were stored at room temperature at a RH of ≤60% until shipment with desiccant to Helix3 for analysis.
Slides were stained with SYBR Gold™ stain and unless precluded by poor cell density and/or poor sample/slide quality, 150 cells per sample (75 cells per slide, if possible) were scored using the Komet© Image Analysis System (Andor Technology, Northern Ireland). The image analysis version and settings were documented in the raw data. For each sample ghosts defined as comets with heavily diffused tail and a non-discernable head that cannot be accurately measured by image analysis were counted in parallel with the image analysis scoring. Slides were scored without knowledge of the sample treatment group. - Evaluation criteria:
- Criteria for a Valid Test
a. Where no statistically significant (p<0.05) response in DNA damage as measured by %Tail is detected at any test article dose concentration, the concurrent positive control must induce a statistically significant increase in the same genotoxic endpoint when compared to the concurrent vehicle control.
b. The concurrent negative control must be considered acceptable for addition to the Helix3 historical control database by providing a sufficient dynamic range to detect a statistically significant positive effect.
Criteria for a Positive Response
An experienced scientific investigator classifies a test article as positive, equivocal, or negative for inducing genotoxicity based on the results of the statistical analysis and the biological relevance of the results, taking into consideration the appropriateness of the concurrent control data and the reproducibility of the results in any repeat experiments.
The test article may be classified as positive for inducing genotoxicity if the following criteria are met:
a. a statistically significant increase in DNA damage is detected at one or more dose concentrations and
b. a statistically significant dose dependent response is detected in the same tissue
If cytotoxicity is detected in the same tissue and dose concentration(s) at which a significant increase in DNA damage is detected, cytotoxicity may be considered a confounding factor in the determination of genotoxicity. Where cytotoxicity may be a confounding factor and/or when cytotoxicity is present in all doses tested, a repeat study including lower non-cytotoxic doses may be conducted to evaluate for the presence of genotoxicity in the absence of cytotoxicity.
A test article may be classified as equivocal for inducing genotoxicity if either criteria (a) or (b) are met, but not both. If the results are equivocal and/or the biological relevance of the results are unclear, a repeat study at the same doses may be con - Statistics:
- Mean values and standard deviations were calculated. In addition, the median of the values from each slide was determined and for each animal the mean of the median from the slides were calculated. To be consistent with historical control comet data generated at Helix3, the individual animal mean %Tail values was calculated as the mean of the total cells scored. The following statistical analyses were carried out, additionally. For each test, a 95% confidence Interval (P<0.05) was used to determine statistical significance:
Parameter: Statistical test
%Tail and %LMW data distribution: Shapiro-Wilk, test group 0 only
%Tail and %LMW equality of variances: Bartlett test (2-tailed; test groups 0 and 1 to 3; test groups 0 and 4 to 6)
Normally distributed data with equal variances: Dunnett (2-tailed; test groups 1 to 3 compared with test group 0; test groups 4 to 6 compared with test group 0); Line fit trend test (2-tailed; test groups 0 and 1 to 3; test groups 0 and 4 to 6)
Non-normally distributed data or unequal variances: Steel ((2-tailed; test groups 1 to 3 compared with test group 0; test groups 4 to 6 compared with test group 0); Kendall rank trend test (2-tailed; test groups 0 and 1 to 3; test groups 0 and 4 to 6)
%Tail and %LMW References and Positive control only: Fisher’s F-test (2-tailed; test group 7 compared with test group 0; test group 8 compared with test group 0; test group 9 compared with test group 0); student’s t-test for equal variances or Welch’s t-test for unequal variances (1-tailed; test group 7 compared with test group 0; test group 8 compared with test group 0; test group 9 compared with test group 0) - Key result
- Sex:
- male
- Genotoxicity:
- negative
- Toxicity:
- no effects
- Vehicle controls validity:
- valid
- Negative controls validity:
- valid
- Positive controls validity:
- valid
- Additional information on results:
- COMET ASSAY
- in the nasal epithelium tissue:
The assessment of the DNA damage in the nasal epithelium showed that neither Zinc oxide T0420 nor Zinc oxide T0421 showed a potential to induce DNA damage (see table in any other info on results). The mean % tail DNA of test groups treated with Zinc oxide T0420 ranged between 14.6 – 15.9% (mean of mean values) and 8.3 - 9.3% (mean of medians) and for Zinc oxide T0421 the range was between 15.9 to 16.6% (mean of means) and 8.8 to 10.8% (mean of medians). The values were not statistically significant as compared to the corresponding air control value (16.6 and 10.7% mean of mean and medians, respectively). A dose response was not observed as determined via the two-tailed trend test. Micro-scaled Zinc oxide T0242 showed a lower level of % tail DNA in the comet assay as compared to the air control values. The mean % tail DNA values were 13.4% (mean of means) and 7.8% (mean of medians). The difference between the mean of mean values was statistically significant. However, the difference between the mean of medians was not. Similarly, Zinc sulfate monohydrate also showed lower tail intensity values (15.4 and 7.6% mean of means and medians, respectively) as compared to the air control. However, in this case the drop in the mean of medians was statistically significant but not the mean of means. The group treated with the positive control (EMS) showed a distinct, statistically significant and biologically relevant increase in the mean % DNA tail intensity values (34.9 and 32.3% mean of means and medians, respectively).
The % tail intensity values (mean of means) of all groups (except for the positive control group) were below the upper range (23.1%) of the historical control data range (mean of means).
- in the lung tissue:
The assessment of the DNA damage in the lung tissue also did not show a biologically relevant alteration of the level of observed DNA damage after treatment with either Zinc oxide T0420 or Zinc oxide T0421 as compared to the air control values (The % tail intensity values (mean of means) of all groups (except for the positive control group) were below the upper range (15.3%) of the historical control data range (mean of means) (see table in any other info on results). The mean % tail DNA of test groups treated with Zinc oxide T0420 ranged between 9.9 – 11.5% (mean of mean values) and 4.9 - 6.9% (mean of medians) and for Zinc oxide T0421 the range was between 8.2 to 10.8% (mean of means) and 4.2 to 5.3% (mean of medians). The values were not statistically significant as compared to the corresponding air control value (11.3 and 5.6% mean of mean and medians, respectively). A dose response was not observed as determined via the two-tailed trend test with T0420, however, a negative trend (dose related decrease in the % tail intensity values) was observed in the mean of mean values of the test groups treated with T0421. This effect was not, however, observed in the mean of median values. Micro-scaled Zinc oxide T0242 showed a similar level of % tail DNA in the comet assay as compared to the air control values. The mean % tail DNA values were 8.6% (mean of means) and 4.0% (mean of medians) and not statistically significant as compared to the air control values. Zinc sulfate monohydrate showed slightly lower tail intensity values (8.3 and 4.1% mean of means and medians, respectively) as compared to the air control. The drop in the mean of means was statistically significant but not the mean of medians. The group treated with the positive control (EMS) showed a distinct, statistically significant and biologically relevant increase in the mean % DNA tail intensity values (52.8 and 52.4% mean of means and medians, respectively).
The % tail intensity values (mean of means) of all groups (except for the positive control group) were below the upper range (15.3%) of the historical control data range (mean of means).
- in the liver:
The % tail DNA intensity in the liver tissue (see table in any other info on results) ranged between 6.9 – 8.7% (mean of means) and 2.7 to 3.8% (mean of medians) for test groups treated with various concentrations of T0420. The mean of mean value of the test group treated with 8 mg/m³ (8.7 ± 1.08%) was statistically higher than the respective air control value (6.6 ± 1.15%). Furthermore, a concentration related trend was observed in the groups treated with 0.5, 2.0 and 8.0 mg/m3 Zinc oxide T0420. However, the mean of median value at 8.0 mg/m³ (3.8 ± 1.19%) was not statistically higher than its respective air control value (2.8 ± 1.00%) and the trend observed when using the mean of means was not observed when using the mean of medians. The mean % tail DNA of test groups treated with Zinc oxide T0421 ranged between 7.7 – 8.7% (mean of mean values) and 3.1-3.6% (mean of medians). The values were not statistically significant as compared to the corresponding air control value (6.6 and 2.8% mean of mean and medians, respectively). A dose response was not observed as determined via the two-tailed trend test. Micro-scaled Zinc oxide T0242 showed a similar level of % tail DNA in the comet assay as compared to the air control values. The mean % tail DNA values were 7.6% (mean of means) and 3.2% (mean of medians) and not statistically significant as compared to the air control values. Similarly, Zinc sulfate monohydrate also did not show significantly altered tail intensity values (7.5 and 3.1% mean of means and medians, respectively) as compared to the air control. The group treated with the positive control (EMS) showed a distinct, statistically significant and biologically relevant increase in the mean % DNA tail intensity values (32.1 and 30.7% mean of means and medians, respectively).
The % tail intensity values (mean of means) of all groups (except for the positive control group) were below the upper range (16.9%) of the historical control data range (mean of means).
- in the bone marrow:
The assessment of the DNA damage in the bone showed that neither Zinc oxide T0420 nor Zinc oxide T0421 showed a potential to induce DNA damage (see table in any other info on results). The mean % tail DNA of test groups treated with Zinc oxide T0420 ranged between 6.1 - 6.6% (mean of mean values) and 2.7 – 2.9% (mean of medians) and for Zinc oxide T0421 the range was between 5.2 to 6.6% (mean of means) and 2.6 to 2.9% (mean of medians). The values were not statistically significant as compared to the corresponding air control value (6.2 and 2.9% mean of mean and medians, respectively). A dose response was not observed as determined via the two-tailed trend test. Micro-scaled Zinc oxide T0242 showed a similar level of % tail DNA in the comet assay as compared to the air control values. The mean % tail DNA values were 5.4% (mean of means) and 2.2% (mean of medians) and not statistically significant as compared to the air control values. Similarly, Zinc sulfate monohydrate also showed similar and not statistically significant tail intensity values (6.0 and 2.5% mean of means and medians, respectively) as compared to the air control. The group treated with the positive control (EMS) showed a distinct, statistically significant and biologically relevant increase in the mean % DNA tail intensity values (32.4 and 30.6% mean of means and medians, respectively).
The % tail intensity values (mean of means) of all groups (except for the positive control group) were below the upper range (11.0%) of the historical control data range (mean of means).
CYTOTOXICITY:
The assessment of the potential of the test and reference substances to induce cytotoxicity in the LMW DNA diffusion assay did not show any significant increases in the percentage of diffused low molecular weight DNA in any of the tested tissues and doses (see table in any other info on results), except for micro-scaled Zinc oxide T0242, which induced a statistically higher diffusible LMW DNA (12.6%) as compared to the corresponding air control (8.6%). The positive control (EMS), however, induced statistically significant increases in the amount of diffused LMW DNA in all examined organs.
The comparison of the obtained LMW DNA data with the historical control data showed that all values from the nasal epithelium (including the air control values; 26.8 - 36.8%) were above the maximum value obtained in the historical control data for this tissue (8.0%). In the lung all values (8.4 - 12.6%), except for the positive control group, were below the maximum value of the historical control data range for the lung (17.6%). In the liver the values were similarly below the maximum historical control data range (13.6%), except for the low dose group of T0420, which had a value of 15.2% and the positive control group (38.2%). All bone marrow values (12.0 – 16.8% for the test and reference substances and 34.6% for the positive control group) were also above the upper limit of the historical control data (6.8%).
TOXICITY
- General toxicity: No Signs of general toxicity. No impairment of body weight gain.
- Local effects: In lavage, several parameters were increased in a concentration related manner in animals exposed to T0420 and T0421 indicating inflammation process in the lung (see table ' Changes in mean total protein and enzyme levels in BAL' in any other info on results)
- Conclusions:
- In this study, male Wistar rats were whole-body exposed to dust aerosols of T0420 and T0421 at target concentrations of 0.5, 2 and 8 mg/m³, as well as to 8 mg/m³ micronsize zinc oxide or 18 mg/m³ zinc sulfate monohydrate for 6 hours daily on 14 consecutive days. A concurrent control group was exposed to conditioned air.
The tested atmospheric were met and they were maintained throughout the study. Cascade impactor measurement of both substances showed particle sizes within the respirable range. There were no signs of toxicity, nor were there any impairment of body weight gain. In the range finding 14-day study (BASF project no.: 36I0050/20I005) animals of the mid dose groups were nominally exposed to 12 mg/m³. The actual assessment of test substance concentrations, however, revealed an exposure to 10.9 and 10.8 mg/m³ for T0420 and T0421, respectively. At this dose level significant histopathological alterations (grade 2 degeneration/regeneration, olfactory epithelium) as well as alterations indicative of inflammatory responses in the lung were observed, which could have had a confounding impact on the outcome of the comet assays results. Thus, the top exposure concentration was slightly reduced to 8 mg/m³. At this level in the lavage fluid, several parameters were increased in a concentration related manner in animals exposed to T0420 and T0421, indicating inflammation process in the lung. These findings were comparable with those observed previously in the range finding 14-day inhalation study with these two test materials. Similar findings were observed in animals exposed to the reference substances.
LMW DNA diffusion assay
In all test groups using the test or reference substances, the quantification of the diffused low molecular weight DNA as a measure for cytotoxicity did not show any indication of excessive cytotoxicity as compared to the respective air control value, which could have hampered the interpretation of the comet assay data. The significantly increased value observed for the lung using micro-scaled Zinc oxide T0242 was not high enough to interfere the comet data evaluation. The positive control group, however, showed significant increases in the LMW DNA value. This is, however, expected from the positive control considering the amount of DNA damage it has induced. The fact that all diffusible LMW DNA values from bone marrow and nasal epithelium samples were above the historical control data, does not have an impact on the data interpretation, since the air control values were also above the historical control data range and furthermore, the high LMW DNA values did not have an impact on the % tail intensities, which were all within the historical control data range for these two organs.
Comet assay
The study is considered as valid, since all concurrent negative control data are acceptable for addition to the historical data base. Furthermore, the positive control group showed that the test is able to detect positive response in all assessed tissues.
The assessment of the comet assay in the various tissues did not show and biologically relevant indication of a genotoxic potential in any of the test or reference substances. The significant concentration dependent increase observed in the mean of mean values obtained from the liver of animals treated with T0420 is not considered as relevant, since the same values were not statistically significant, when the mean of medians was used instead of mean of means. This indicates that the observed effect is more likely due to the greater impact of individual values on the group mean value when using the mean of means. The current OECD guideline, therefore, also recommends the use of mean of medians. The statistically significant decreases in the observed in the lung and nasal epithelium using Zinc oxide T0421, micro-scaled Zinc oxide T0242 and Zinc sulfate are not considered as biologically relevant effects, since once again the statistical significance is only observed in either mean of mean or mean of median calculations.
CONCLUSION
In conclusion it can be stated that under the described circumstances neither Zinc oxide T0420 nor Zinc oxide T0421 showed a genotoxic potential in the nasal epithelium, lung liver and bone marrow of rats exposed for 14 days via inhalation.
- Executive summary:
In this study two nanosized test substances Zinc oxide T0420 and Zinc oxide T0421 were assessed for their genotoxic potential using the alkaline comet assay after a 14-day exposure period via inhalation. This was a multisite study, where the in-life phase, necropsy, as well as the examination of the lung lavage fluid was performed by the test facility. The processing of the isolated tissues and the comet assay was performed by the test site (Helix 3 Inc.). The target tissues addressed in this study were the nasal epithelium, the lung, the liver as well as the bone marrow.
The concentrations used in this study was based on a separately performed dose range finding assay (BASF Project no.: 36I0050/20I005). The three concentrations ,selected based on the dose range finding study, were 0.5, 2.0 and 8.0 mg/m³. Wistar rats were exposed whole-body to the indicated concentrations of each test substance for a 6 h period per day for 14 days. In addition, for comparison, a micro-scaled Zinc oxide as well as well as a soluble Zinc salt (Zinc sulfate monohydrate) were tested in parallel under the same conditions at a single (equimolar) concentration. Ethylmethane sulfonate (EMS) was used as a positive control and applied orally at a single time point.
During the exposure period, the animals were observed for signs of toxicity before, during and after the exposure. Body weight was determined once weekly. The following mean concentrations and particle size distribution were determined.
In animals exposed to test item 1, concentration-related increases of lavage parameters were observed, as well as in animals exposed to test item 2. The exposure of single concentration of reference substance 1 and 2 caused significantly increases in most of the lavage parameters. The changes in lavage fluid demonstrate the toxicity in the lungs, which were comparable with the range finding study.
The assessment of the target tissues in the comet assay did not show any biologically relevant increases in the % tail intensity of the analyzed tissues under the indicated conditions. The positive control group showed a distinct and statistically significant increase in all analyzed tissues.
Thus, under the indicated circumstances, the two test substances as well as the reference substances (micro-scaled Zinc oxide and Zinc sulfate monohydrate) are considered as non-genotoxic in this assay.
Referenceopen allclose all
Table 1. Red blood cell formation and micronucleus induction in bone marrow of rats, after 14 days of exposure to clean air, cyclophosphamide, and uncoated Z-COTE.
Treatment group |
Concentration, sampling time |
PCE/500 RBC |
PCE:NCE |
MN/2000 PCE |
% MN PCE |
Negative control, |
24 h |
♂ 86 |
♂ 0.22 |
♂ 5.8 |
♂ 0.29 |
Positive control, |
20 mg/kg b.w., |
♂ 67 |
♂ 0.16 |
♂ 55.4** |
♂ 2.77** |
Z-COTE |
7.93 mg/m3, 24 h |
♂ 86 |
♂ 0.21 |
♂ 4.2 |
♂ 0.21 |
PCE: Polychromatic erythrocytes; NCE: Normochromatic erythrocytes; RBC: Red blood cells; MN: Micronuclei; % MN PCE: Percent micronucleated PCE; Significantly different from negative controls:*:P≤0.05, **:P≤0.01, Mann-Whitney Rank Sum Test; (*): P ≤0.05, Student’st-Test.
Table 1. hOGG1-modified Comet assay, DNA-damage in BAL cells after 1 and 14 days of recovery.
|
Tail intensity [%] Mean ± SD1 |
Tail intensity [%] Mean ± SD1 |
||
Post exposure day |
1 |
14 |
||
Treatment |
hOGG1 |
hOGG1 |
||
|
without |
with |
without |
with |
Vehicle control: Clean air |
0.6 ± 0.98 |
1.1 ± 1.67 |
0.5 ± 0.28 |
0.5 ± 0.31 |
Z-COTE: 8.24 mg/m³ |
0.2 ± 0.08 |
0.2 ± 0.09 |
1.1 ± 1.40 |
1.4 ± 1.58 |
Positive control: KBrO3 |
1.3 ± 1.10 |
7.8 ± 3.83# |
0.5 ± 0.22 |
7.9 ± 2.51## |
#/## Significantly different from samples incubated without hOGG1: p ≤ 0.05, Student’s t-test for paired values.
1Mean derived from medians of approx. 100 nuclei per animal and 5 animals per group.
Table 2. 8-OH-dG positive nuclei in terminal bronchioles and lung parenchymal cells
|
8_OH-dG |
|
|
Positive nuclei per 10,000 μm² Mean ± SD |
|
Post exposure day |
1 |
14 |
Vehicle control: Clean air |
6.92 ± 1.34 |
7.63 ± 0.78 |
Z-COTE: 8.24 mg/m³ |
7.37 ± 1.22 |
8.08 ± 0.97 |
Table 3. In vivo control values rfom independent experiment performed by the same toxicological laboratory in 2020.
Group and treatment |
Animal number |
Sacrifice day |
Median Tail Intensity [%] |
|
Arithmetic Mean Animals |
Group Mean |
Slide A |
Slide B |
|||||
Vehicle control |
1201 |
1 |
0.08 |
0.06 |
0.07 |
0.32 |
1202 |
1 |
0.22 |
0.19 |
0.20 |
||
1203 |
1 |
0.08 |
0.28 |
0.18 |
||
52 |
2 |
0.69 |
0.47 |
0.58 |
||
107 |
3 |
0.62 |
0.71 |
0.66 |
||
110 |
3 |
0.20 |
0.29 |
0.24 |
||
Positive control: EMS |
2 |
1 |
11.14 |
12.21 |
11.67 |
11.72 |
3 |
1 |
13.56 |
14.43 |
14.00 |
||
4 |
2 |
12.01 |
9.27 |
10.64 |
||
5 |
2 |
10.30 |
9.34 |
9.82 |
||
6 |
3 |
12.82 |
11.21 |
12.01 |
||
7 |
3 |
13.41 |
11.54 |
12.47 |
Table. Results of the comet assay in the nasal epithelium
Test group | Test Substance | Concentration | % Tail intensity ± SD | |
Mean of means | Mean of medians | |||
0 | Air control | 0 | 16.6 ± 2.64 | 10.7 ± 2.89 |
1 | Zinc Oxide T0420 | 0.5 | 14.6 ± 3.62 | 9.3 ± 2.64 |
2 | 2.0 | 15.9 ± 3.60 | 9.0 ± 2.86 | |
3 | 8.0 | 14.8 ± 3.00 | 8.3 ± 1.69 | |
4 | Zinc Oxide T0421 | 0.5 | 16.6 ± 5.28 | 10.8 ± 5.05 |
5 | 2.0 | 15.9 ± 4.73 | 9.9 ± 4.03 | |
6 | 8.0 | 16.0 ± 3.34 | 8.8 ± 3.91 | |
7 | Zinc Oxide T0242 | 8.0 | 13.4 ± 2.67# | 7.8 ± 2.13 |
8 | Zinc sulfate monohydrate | 18.0 | 15.4 ± 1.89 | 7.6 ± 1.28$ |
9 | EMS | 300 mg/kg b.w. | 34.9 ± 3.63* | 32.3 ± 4.12* |
#: statistically significant P=0.048 (decrease)
$: statistically significant P=0.031 (decrease)
*: statistically significant P<0.001 (increase)
Table. Results of the comet assay in the lung
Test group | Test Substance | Concentration | % Tail intensity ± SD | |
Mean of means | Mean of medians | |||
0 | Air control | 0 | 11.3 ± 1.93 | 5.6 ± 1.50 |
1 | Zinc Oxide T0420 | 0.5 | 11.5 ± 3.25 | 6.9 ± 4.15 |
2 | 2.0 | 9.9 ± 1.62 | 4.9 ± 1.19 | |
3 | 8.0 | 10.1 ± 2.70 | 5.4 ± 2.89 | |
4 | Zinc Oxide T0421 | 0.5 | 10.8 ± 3.681 | 5.3 ± 2.67 |
5 | 2.0 | 9.0 ± 1.541 | 4.5 ± 1.34 | |
6 | 8.0 | 8.2 ± 1.581 | 4.2 ± 1.60 | |
7 | Zinc Oxide T0242 | 8.0 | 8.6 ± 2.85 | 4.0 ± 1.60 |
8 | Zinc sulfate monohydrate | 18.0 | 8.3 ± 1.69# | 4.1 ± 1.51 |
9 | EMS | 300 mg/kg b.w. | 52.8 ± 6.84* | 52.4 ± 7.84* |
#: statistically significant P=0.015 (decrease)
*: statistically significant P<0.001 (increase)
1: negative trend statistically significant P=0.047
Table. Results of the comet assay in the liver
Test group | Test Substance | Concentration | % Tail intensity ± SD | |
Mean of means | Mean of medians | |||
0 | Air control | 0 | 6.6 ± 1.15 | 2.8 ± 1.00 |
1 | Zinc Oxide T0420 | 0.5 | 7.9 ± 0.491 | 3.2 ± 0.53 |
2 | 2.0 | 6.9 ± 1.301 | 2.7 ± 0.38 | |
3 | 8.0 | 8.7 ± 1.08#1 | 3.8 ± 1.19 | |
4 | Zinc Oxide T0421 | 0.5 | 8.7 ± 0.91 | 3.1 ± 0.80 |
5 | 2.0 | 7.8 ± 1.05 | 3.6 ± 0.73 | |
6 | 8.0 | 7.7 ± 2.25 | 3.1 ± 0.83 | |
7 | Zinc Oxide T0242 | 8.0 | 7.6 ± 2.03 | 3.2 ± 1.11 |
8 | Zinc sulfate monohydrate | 18.0 | 7.5 ± 2.03 | 3.1 ± 1.26 |
9 | EMS | 300 mg/kg b.w. | 32.1 ± 2.55* | 30.7 ± 3.07* |
#: statistically significant P=0.016
*: statistically significant P<0.001
1: positive trend statistically significant P=0.032
Table. Results of the comet assay in the bone marrow
Test group | Test Substance | Concentration | % Tail intensity ± SD | |
Mean of means | Mean of medians | |||
0 | Air control | 0 | 6.2 ± 1.00 | 2.9 ± 0.66 |
1 | Zinc Oxide T0420 | 0.5 | 6.6 ± 1.02 | 2.9 ± 0.52 |
2 | 2.0 | 6.5 ± 0.78 | 2.8 ± 0.76 | |
3 | 8.0 | 6.1 ± 0.30 | 2.7 ± 0.42 | |
4 | Zinc Oxide T0421 | 0.5 | 6.0 ± 0.60 | 2.9 ± 0.68 |
5 | 2.0 | 6.6 ± 1.63 | 2.9 ± 0.67 | |
6 | 8.0 | 5.2 ± 0.80 | 2.6 ± 0.75 | |
7 | Zinc Oxide T0242 | 8.0 | 5.4 ± 0.55 | 2.2 ± 0.54 |
8 | Zinc sulfate monohydrate | 18.0 | 6.0 ± 0.83 | 2.5 ± 0.37 |
9 | EMS | 300 mg/kg b.w. | 32.4 ± 4.84* | 30.6 ± 5.53* |
*: statistically significant P<0.001
Table. Results of the LMW DNA diffusion assay
Test group | Test Substance | Concentration | Group Mean ± SD (%) | |||
Nasal epithelium | Lung | Liver | Bone marrow | |||
0 | Air control | 0 | 30.0 ± 9.19 | 8.6 ± 1.52 | 12.6 ± 5.37 | 14.8 ± 2.17 |
1 | Zinc Oxide T0420 | 0.5 | 26.8 ± 6.76 | 11.6 ± 3.29 | 15.2 ± 6.02 | 13.6 ± 2.07 |
2 | 2.0 | 33.8 ± 8.98 | 11.4 ± 2.51 | 13.2 ± 3.35 | 14.0 ± 3.54 | |
3 | 8.0 | 35.8 ± 16.40 | 10.6 ± 1.52 | 13.4 ± 6.95 | 13.8 ± 5.02 | |
4 | Zinc Oxide T0421 | 0.5 | 31.6 ± 8.62 | 11.8 ± 3.56 | 12.6 ± 6.80 | 12.0 ± 3.54 |
5 | 2.0 | 36.8 ± 15.67 | 8.4 ± 3.36 | 10.0 ± 4.30 | 16.8 ± 5.89 | |
6 | 8.0 | 34.6 ± 14.24 | 10.4 ± 2.61 | 11.0 ± 3.74 | 15.2 ± 5.40 | |
7 | Zinc Oxide T0242 | 8.0 | 29.0 ± 11.05 | 12.6 ± 4.22€ | 11.8 ± 4.09 | 15.6 ± 5.98 |
8 | Zinc sulfate monohydrate | 18.0 | 33.0 ± 8.80 | 12.0 ± 5.92 | 10.6 ± 7.64 | 14.6 ± 6.66 |
9 | EMS | 300 mg/kg b.w. | 39.8 ± 4.49* | 26.8 ± 13.37# | 38.2 ± 7.05$ | 34.6 ± 6.73$ |
*: statistically significant P=0.032
€: statistically significant P=0.041
#: statistically significant P=0.019
$: statistically significant P=0.032
Table. Changes in mean total protein and enzyme levels in BAL (x-fold of concurrent control) in male rats after inhalation exposure to Zinc oxide T0420 on 14 consecutive days
Analyte | Gr. 1 0.5 mg/m3 | Gr.2 2 mg/m3 | Gr.3 8 mg/m3 |
Total Protein | 1.0 | 1.3 | 5.1** |
GGT | 1.1 | 2.4** | 3.6** |
LDH | 1.0 | 1.6* | 7.7** |
ALP | 1.6* | 2.2** | 15.3** |
NAG | 1.0 | 1.2 | 2.0** |
GGT = γ-Glutamyl-transferase; LDH = Lactate dehydrogenase; ALP = Alkaline phosphatase; NAG = β-N-Acetyl glucosaminidase, One-sided Wilcoxon-test: * : p £ 0.05; ** : p £ 0.01
Endpoint conclusion
- Endpoint conclusion:
- no adverse effect observed (negative)
Additional information
This section addresses the genetic toxicity of nano-size zinc oxide, summarising all available in vitro and in vivo data. The genetic toxicity data for non-nano zinc oxide as well as other zinc substances of the zinc category are summarised in a separate endpoint summary.
This assessment is restricted to those studies which are relevant from a regulatory point of view and fulfil the relevance reliability and adequacy criteria as e.g. laid down in the ECHA Guidance on information requirements and chemical safety assessment, Chapter R.4: Evaluation of available information. In contrast, studies reporting DNA damage in bacteria, induction of SCE in mammalian cells or tests in inappropriate test systems such as yeasts are considered to contribute far less to the overall assessment and are therefore not further discussed.
1 In vitro
1.1 bacterial reverse mutation assays
Landsiedel, R. et al. (2010) performed in a bacterial reverse mutation assay in Salmonella typhimurium strains TA 98, TA 100, TA 102, TA 1535 and TA 1537 with Z-COTE® MAX both in presence and absence of a metabolic activation system. According to the authors, Z-COTE® MAX did not show mutagenic activity in the standard plate incorporation nor in the preincubation S. typhimurium reverse mutation assay with and without exogenous metabolic activation tested up to 5000 µg per plate. The positive controls used are not adequate to demonstrate adequateness of the test system (non-particulate test material). Historical control data is missing. Five doses of test substance (i.e., 20, 100, 500, 1000, 2500 and 5000 µg/plate) together with concurrent vehicle controls and appropriate positive controls were tested in triplicate. Since the choice of the vehicle is one of the most critical steps and has a great impact on the particle size distribution and the bioavailability of a test substance, different vehicles were used in the standard plate incorporation test (dimethylsulfoxide) and in the preincubation test (fetal bovine serum). The test substance preparations were dispersions in the respective vehicles. No mutagenic activity was observed in the standard plate incorporation and in the preincubation S. typhimurium reverse mutation assay with and without exogenous metabolic activation tested up to 5,000 mg per plate. This guideline study is considered reliable without restriction (RL1).
Kwon, J.Y. et al. (2014) investigated on the mutagenic activity of two different ZnO NP types, each prepared in two different suspensions, utilising a bacterial reverse mutation assay with S. typhimurium strains TA 98, TA 100, TA 1535, and TA 1537 as well as E. coli WP2 uvrA. The four different test material suspensions were tested both in absence and presence of a metabolic activation system. The tester strains were exposed to the test material preparations for 48-72 hours. Each experiment was performed in triplicate. According to the authors, the ZnO NP preparations did not increase the number of revertant colonies in any strain tested both in absence and presence of a metabolic activation system. In contrast, the positive control substances induced increases in the revertant colony frequency. No bacteriotoxic effects were observed in a preliminary dose range finder. No conclusion can be drawn due to reporting and methodological deficiencies. The test material is insufficiently characterised, since information on purities, impurities, and surface modifications is missing. Uptake of the test material was not investigated. The identities of the positive control substances are not specified. The description of the bacterial reverse mutation assay methodology lacks details. Information on confounding factors, i.e. precipitation and cytotoxicity, in the main experiments is not provided. No confirmatory experiment was performed. Evaluation criteria are not specified. Historical control data is missing. Based on the above-mentioned shortcomings the reference is considered not reliable and therefore disregarded for the hazard assessment.
Li, C.-H. et al. (2011) performed a Salmonella reverse mutation assay in order to evaluate the mutagenic potential of ZnO NPs and ZnO MPs. The assay was performed according to the plate incorporation method both in presence and absence of a metabolic activation system. Three independent experiments were performed. According to the authors, neither ZnO NPs nor ZnO MPs induced a significant increase in the revertant colony number in any of the strains tested, when compared to the solvent control. The positive controls elicit a significant response as compared to the solvent controls. No conclusion can be drawn due to reporting and methodological deficiencies. The test material is insufficiently characterised, since information on purities and impurities is missing. Description of the test material preparation lacks details. Uptake of the test material was not investigated. Information on confounding factors, i.e. precipitation and cytotoxicity, in the main experiments is not provided. No confirmatory experiment was performed. Historical control data is missing. Based on the above-mentioned shortcomings the reference is considered not reliable (RL3) and therefore disregarded for the hazard assessment.
Pan et al. (2010) investigated on the mutagenicity of zinc oxide nanoparticles using a bacterial reverse mutation assay. The tester strains Salmonella typhimurium TA 97a, TA 100, and Escherichia coli WP2 uvr A were exposed to zinc oxide nanoparticles at concentration levels of 10, 100, and 1000 µg/mL both in absence and presence of a S9 metabolic activation system. Vehicle and positive control cultures were run concurrently. The experiment was run in triplicates. The zinc oxide nanoparticles did not induce growth inhibition of tested bacterial strains at any concentrations used. No mutagenicity was observed in all three tested strains without S9 metabolic activation. Using a preincubation procedure and high S9 (9%) activation, the number of revertant Escherichia coli WP2 uvr A colonies was statistically significantly increased after treatment with the highest zinc oxide nanoparticle concentration, when compared to the concurrent vehicle control value. The increase was, however, only marginal, i.e., 1.4-fold above the concurrent vehicle control level. This increase in considered to be not biologically relevant, since usually the increase in considered to be relevant for the E. coli WP2 uvr A tester strain only at ≥2-fold increases above the concurrent vehicle control value. Furthermore, it appears to be implausible that a metal oxide without surface modifications shows a relevant increase in the revertant colony number only in presence of a metabolic activation system. No conclusion can be drawn due to major deficiencies with regards to reporting and the methodology applied. The test material is insufficiently characterised, since information on the purity and the manufacturer are not provided. Only three tester strains were included, whereas the testing of five different strain is foreseen by the OECD TG 471 (1997). Moreover, according the test guideline, at least five adequately spaced concentration levels should be tested; however, this study included only three concentration levels with logarithmic spacing. Furthermore, the authors did not perform a repeat experiment to confirm the results obtained. The number of viable cells treated is not specified. Furthermore, information on test material precipitation is not provided. Historical control data ranges are not provided. Evaluation and scoring criteria are not specified. The test material was not tested up to cytotoxic or precipitating concentrations. The positive control used for testing in absence of S9 are not adequate. In general, the positive control showed only a 2-3-fold increase in the number of revertant colonies. Individual plate counts are not reported. Based on the above-mentioned shortcomings the reference is considered not reliable (RL3) and therefore disregarded for the hazard assessment.
Yoshida et al. (2009) conducted a mutagenicity test on water soluble ZnO nanoparticles capped with tetramethylammonium hydroxide (TMAOH) in a bacterial reverse mutation assay using Salmonella typhimurium strains TA98, TA100, TA1537, and Escherichia coli strain WP2urvA- with and without metabolic activation by S9 mix in the preincubation method. The amount of TMAOH-capped ZnO nanoparticles with excess TMAOH molecules used were 0, 39.1, 78.1, 156, 313, 625, 1250, 2500 and 5000 µg/plate. Because the molecular weight of ZnO nanoparticles is quite large, the dose of ZnO nanoparticles would be high compared with that of the positive control. Growth inhibition was observed in all tester strains with or without S9 mix due to addition of TMAOH-capped ZnO nanoparticles in cases of high-dose. Large increases in the number of revertant colonies were seen for the positive control in all cases, indicating that the test system responded appropriately. However, mutagenicity was negative in all strains with and without metabolic activation. Under the test conditions, ZnO nanoparticles did not induce mutations in bacterial reverse mutation assay. This study is comparable to a guideline study and considered reliable with restriction (RL2).
BASF 2012
*** discussion will be added in due course ***
Li, C.-H. et al. (2012) performed a Salmonella reverse mutation assay in order to evaluate the mutagenic potential ZnO NP. The assay was performed according to the plate incorporation method both in presence and absence of a metabolic activation system. Three independent experiments were performed. According to the authors, ZnO NP did not induce a significant increase in the revertant colony number in any of the strains tested, when compared to the solvent control. The positive controls elicit a significant response as compared to the solvent controls. The test material is insufficiently characterised, since information on purities and impurities is missing. Information on confounding factors, i.e. precipitation and cytotoxicity, in the main experiments is not provided. No confirmatory experiment was performed. Historical control data is missing. Based on the above-mentioned shortcomings the reference is considered reliable with restriction (RL2) and only suitable for the weight of evidence analysis of the genetic toxicity of ZnO.
1.2 in vitro mammalian mutagenicity tests (TK/HPRT)
Ziemann (2011) conducted an in vitro gene mutation assay with nanoscaled ZnO. Mouse lymphoma L5178Y/ TK± cells in suspension culture were treated for 4 hours with different concentrations of the test item with and without S9 -mix. Medium, medium with soy lecithine, methyl methanesulfonate and cyclophosphamide were used as negative control, vehicle control, positive control without S9 -mix and positive control with S9 -mix, respectively. The gene mutation potential of the test item Z-COTE HP1 (coated nanoscaled ZnO) was determined. Z-COTE HP1 induced increases of MF with and without S9 -mix with a concentration-dependency in the presence of S9 -mix, which was significantly elevated almost exclusively at the highest concentration. The increase mutant colonies were primarily characterised as small colonies, indicating a clastogenic event. The significantly increased MF was always linked to pronounced cytotoxicity. For this reason and the limited significance of the test system for particles, the test results should more likely be judged as questionable in L5178Y/TK cells under the conditions and restrictions of this assay, implying a possible false positive result. Consequently, the results are interpreted as equivocal, as the clastogenic findings were confounded with cytotoxicity. This guideline study is considered reliable without restriction (RL1).
Ziemann (2011) conducted an in vitro gene mutation assay with coated nanoscaled ZnO. Mouse lymphoma L5178Y/ TK± cells in suspension culture were treated for 4 hours with different concentrations of the test item with and without S9 -mix. Medium, medium with soy lecithine, methyl methanesulfonate and cyclophosphamide were used as negative control, vehicle control, positive control without S9 -mix and positive control with S9 -mix, respectively. The gene mutation potential of the test item Z-COTE HP1 (coated nanoscaled ZnO) was determined. Z-COTE HP1 induced increases of MF with and without S9 -mix with a concentration-dependency in the presence of S9 -mix, which was significantly elevated almost exclusively at the highest concentration. The increase mutant colonies were primarily characterised as small colonies, indicating a clastogenic event. The significantly increased MF was always linked to pronounced cytotoxicity. For this reason and the limited significance of the test system for particles, the test results should more likely be judged as questionable in L5178Y/TK cells under the conditions and restrictions of this assay, implying a possible false positive result. Consequently, the results are interpreted as equivocal, as the clastogenic findings were confounded with cytotoxicity. This guideline study is considered reliable without restriction (RL1).
Jain, A.K. et al. (2018) examined the capacity of ZnO NP to induce gene mutations at the Hprt locus in V79 (Chinese hamster fibroblasts). The assay was performed according to OECD TG 476 (1997). Moreover, cytotoxicity was analysed utilising the MTT assay as well as by analysis of apoptosis and cell cycle. In addition, cellular uptake and ROS generation were analysed. According to the authors, ZnO NPs induced a significant and concentration-dependent increase in the mutant frequency in V79 cells treated for 6 h starting at a concentration of 10 µg/mL. Moreover, cytotoxic effects were observed at concentrations of 15-20 and 10-20 µg/mL in cells treated for 6 and 24 h, respectively, as evidenced by the MTT assay. Moreover, induction of apoptosis and increased proportions of cells in the G2-M cell cycle phase by a 24-h ZnO NP treatment were observed in further analyses. Further, a significant concentration-dependent cellular uptake of test material was observed in cells treated for 24 h. The particles were predominantly found in vesicles but also sporadically in the nucleus. Intracellular ROS levels were significantly increased in cells exposed for 6 and 24 h to concentrations of 10 µg/mL and above. The increase showed a concentration-related response. No conclusion can be drawn due to reporting and methodological deficiencies. Description of the Hprt assay methodology lacks details, including details on exposure temperature, replicates, and staining. The number of cells used was lower (1x10^5) than recommend by OECD TG 476 followed (>1x10^6 should be utilised at each step). Cytotoxicity was not evaluated according to the parameters recommended in OECD TG 476 (1997) and was only evaluated in a separate assay utilising the colorimetric MTT assay. Details on the cell line, including cell doubling time, number of passages, and potential mycoplasma infection are missing. Furthermore, the author did not state on precipitation and distribution of ZnO NPs during the treatment. Potential effects on pH or osmolality of the culture medium induced by ZnO NPs were not discussed. Information on scoring and evaluation criteria are unclear or missing. Exposure concentrations are stated in µM and µg/mL; however, the cell line is composed of adherent cells, and thus, it would be appropriate to specify exposure concentrations in µg/cm². Historical control data is not provided. ZnO NP dissolution and Zn ion effects on the cells are neither examined nor discussed. The increase in the mutant frequency was significant but only slightly increased above untreated control (<2.8x). Based on the above-mentioned shortcomings the reference is considered not reliable and therefore disregarded for the hazard assessment.
1.3 in vitro mammalian clastogenicity assays (MN)
Demir et al. (2014) examined the capacity of two different ZnO NP materials to induce chromosomal damage in HEK293 and NIH/3T3 cells utilising the cytokinesis-blocked micronucleus test. Additionally, the authors run a ZnO bulk reference material to compare the difference in response between NP and bulk particles. The cytostasis was evaluated based on the cytokinesis-block proliferation index (CBPI). According to the authors, both ZnO NP types induced significant increases in the frequency of binucleated cells with micronuclei at the two highest concentration tested (100 and 1000 µg/mL). Moreover, the response appeared to be concentration dependent. In contrast, the bulky ZnO form induced no positive response under the conditions tested. The effects observed were induced at concentrations, which concurrently induced significantly decreased CBPIs. No conclusion can be drawn due to reporting and methodological deficiencies. The test item is insufficiently characterised, since information on the purity, impurity elements and surface modifications are missing. The micronucleus test was performed in cell lines, which are not validated for the assay. Moreover, details on the cell lines, including cell doubling time, are missing. The description of the micronucleus test lacks some details. Furthermore, the author did not state on precipitation and distribution of ZnO NPs during the treatment. Especially treatment at excessively high concentrations >500 µg/mL may have resulted in particle overload. Potential effects on pH or osmolality of the culture medium induced by ZnO NPs were not discussed. Information on evaluation criteria are unclear or missing. Exposure concentrations are stated in µg/mL; however, both cell lines are composed of adherent cells, and thus, it would be appropriate to specify exposure concentrations in µg/cm². Cellular uptake of the test material was not investigated. Further, the cytokinesis-blocked micronucleus test is considered to be inadequate to test NP, when cytochalasin B treatment is performed concurrently with NP treatment, since cytochalsin B could potentially interfere with NP uptake during the exposure time. Historical control data is not provided. The positive responses in the micronucleus frequency were only observed in presence significant cytostasis as evidence by the CBPI. Especially, the ZnO NP (<35 nm) type resulted in >55% and >80% cytostasis in HEK293 and NIH/3T3 cells, when treated at two highest concentration. Similarly, the ZnO NP (50-80 nm) type resulted in marked cytostasis (>83%) in the NIH/3T3 cells. In HEK293 cells, the cytostasis was about 17 and 19% at 100 and 1000 µg/mL, respectively. Based on the above-mentioned shortcomings the reference is considered not reliable and therefore disregarded for the hazard assessment.
Kononenko, V. et al. (2017) examined potential chromosomal damage induced by ZnO NPs, ZnO macroparticles (MPs), and ZnCl2 treatment in MDCK cells. The chromosomal damage was evaluated in a cytokinesis-block micronucleus test. Cell viability and cytostatic effects were examined concurrently by analysis of the nuclear division index and scoring of apoptotic or necrotic cells. Moreover, cytotoxicity was investigated utilising the MTT, NRU, and trypan blue exclusion assays. In further experiments, the authors examined ROS generation, ZnO NP dissolution, and the activity of catalase and glutathione-S-transferase (GST) after test material treatment. According to the authors, cell viability and proliferation were not affected under the conditions tested in the micronucleus test. Chromosomal damage was only increased after treatment with ZnO NPs at concentrations of 61 and 123 µM, when compared to untreated controls. Moreover, the test materials induced statistically significant cytotoxicity at concentration of 184 µM in the MTT and NRU assay, whereas the trypan blue exclusion assay indicated considerable cytotoxicity at concentrations of 360 µM and above. GST activity was statistically significantly decreased only after ZnO NP treatment, whereas catalase activity was reduced in treatment with both ZnO NPs and ZnCl2. However, catalase activity was only slightly impaired after ZnCl2 treatment. Acellular measurement of ROS indicated no significant in ROS levels in cell culture media after the 24 h incubation with either ZnO NPs, ZnO MPs, or ZnCl2. No conclusion can be drawn due to reporting and methodological deficiencies. The test item is insufficiently characterised, since information on the purity, impurity elements and surface modifications are missing. Cytotoxicity was not evaluated using parameters recommend by the current test guideline (OECD TG 487, 2016) and the results of the NDI calculation and apoptosis/necrosis evaluation are not shown. The micronucleus test was performed in a cell line, which is not recommended by the current test guideline. Moreover, details on the cell lines, including cell doubling time, are missing. The description of the micronucleus test lacks some details. Furthermore, the authors did not state on precipitation and distribution of ZnO NPs during the treatment. Potential effects on pH or osmolality of the culture medium induced by ZnO NPs were not discussed. Information on evaluation criteria are unclear or missing. Exposure concentrations are stated in µg/mL; however, the cell line is composed of adherent cells, and thus, it would be appropriate to specify exposure concentrations in µg/cm². Cellular uptake of the test material was not investigated. Historical control data is not provided. The micronucleus frequency was only slightly increased and micronucleus frequencies show considerable variability within groups. Based on the above-mentioned shortcomings the reference is considered not reliable and therefore disregarded for the hazard assessment.
Roszak, J. et al. (2016) investigated on cytogenetic damage in BEAS-2B (human bronchial epithelial cells) cells after 48-exposue to ZANO® (ZnO nanosized) and Pharma B (fine ZnO) using the CBMN assay. After 6-h of exposure, cytokinesis was blocked by a cytochalasin B treatment. Each replicate was scored for the number of micronuclei present in 1000 binucleated cells. The experiment was repeated independently with adjusted concentrations. Concurrently, cytostasis was calculated from 500 cells per replicate. In addition, cytotoxicity was evaluated in a separate experiment by counting manually propidium iodide stained cells. According to the authors, both ZnO particle types showed a very steep decreasing dose-response curve in the concentration range of 1.5-3.0 µg/cm². Moreover, nanosized ZnO particles were found to be more cytotoxic in presence of BSA, whereas fine ZnO showed weaker cytotoxic effects in the presence of BSA. Based on the cytotoxicity experiment, a concentration range of 0.75 to 2.5 µg/cm² was used in the micronucleus tests. In the micronucleus test, the first experiment gave a negative outcome under any conditions tested. In contrast, in the second experiment, exposure to nanosized ZnO particles resulted in significantly increased micronucleus frequency at concentrations of 1.5 µg/cm² under all conditions tested. The increase showed, however, no concentration related response. The fine ZnO NP resulted in significant and concentration related increases of the micronucleus frequency, when treated at concentrations of >1.25 and > 1.5 µg/cm² suspended in water or water and BSA, respectively. No conclusion can be drawn due to reporting and methodological deficiencies. The micronucleus test was performed in a cell line, which is not recommended by the current test guideline or the test guideline followed. Moreover, details on the cell lines, including cell doubling time, passages, and mycoplasma infection, are missing. Description of exposure conditions lacks some details, since information on cell density and temperature are not provided. The authors did not state on precipitation and distribution of ZnO NPs during the treatment. Potential effects on pH or osmolality of the culture medium induced by ZnO NPs were not discussed. Information on evaluation criteria are unclear or missing. Exposure to cytochalasin B during the particle exposure could have interfered with cellular NP uptake. Historical control data is missing. Cellular uptake and dissolution of the test material were not investigated. Notably, the results of the two independent experiments show great variability and equivocal results. In experiment 1, all treatments resulted in negative findings, whereas the second experiment showed positive results for both ZnO particle types and combinations with different vehicles used. Confirmatory experiment and experiments with other treatment schedules (according to the test guideline) were not performed. Based on the above-mentioned shortcomings the reference is considered not reliable and therefore disregarded for the hazard assessment.
The study by Gümüş et al. (2014) was conducted to investigate the genotoxic effects of zinc oxide nanoparticles in cultured human peripheral blood lymphocytes using the micronucleus assay. The lymphocytes were exposed to zinc oxide nanoparticles at concentration levels of 1, 2, 5, 10, 15, and 20 µg/mL for 48 hours. Cytokinesis was blocked via cytochalasin B treatment 44 hours after initiation of the culture. Negative and positive (mitomycin C) cultures were run concurrently. A total of 2000 lymphocytes per concentration were scored for the occurrence of micronuclei. As measure of cytotoxicity, the cytokinesis-block proliferation index was determined in parallel. The exposure to zinc oxide nanoparticles resulted in a statistically significantly increased micronucleus number in lymphocytes only at concentration levels of 10 and 15 µg/mL (1.05% and 0.80% micronucleated lymphocytes, respectively). According to the authors, the increase was concentration dependent (r=0.51). Notably, cultures treated at the highest concentration level did not show a statistically significantly increased micronucleus number. Furthermore, the correlation analysis revealed only a medium correlation between the concentration tested and the micronucleus rate. The proportion of micronucleated cells was not calculated; but based on the presented numbers recalculated to be 0.8% and 0.9% at zinc oxide nanoparticle concentration levels of 10 and 15 µg/mL, respectively. Moreover, the highest micronucleus rate observed is within the historical control data range of other reputable laboratories (Harlan 2009-2013: 0.05-1.45 (solvent control); Covance: 0-1.0 (95% vehicle control range). The cytokinesis-block proliferation index was not altered, when compared to the concurrent negative control. The positive control cultures showed a markedly increased micronucleus frequency. The test substance was not tested up to cytotoxic or precipitating (at least not reported) concentrations. No conclusion can be drawn on the results presented due to major deficiencies with regards to reporting and the methodology applied. The test material was not sufficiently characterised, and the test material preparation is only poorly described. The only information given is that the test substance was prepared and characterised by Ada et al. (2008). The description of the cell slide preparation and evaluation is insufficient, since the only information given is a reference to another publication. The authors provide no information on confounding factors, such as test material precipitation or pH and osmolality effects of the test material on the culture medium. Statistical analysis was performed based in the total micronuclei number but not proportion of cells with micronuclei. The proportion of micronuclei was not correctly calculated. Historical control data are not included. Evaluation and scoring criteria are missing. The sex of the blood donors and the normal cell proliferation time of the lymphocytes were not specified. The type of negative control run is not specified. The authors did not examine on cellular uptake of the test material. Based on the above-mentioned shortcomings the reference is considered not reliable and therefore disregarded for the hazard assessment.
A study was conducted by Corradi et al. 2012 to investigate the in situ proliferation of A549 human lung epithelial carcinoma cells exposed to nanoparticles in the presence or absence of 10% serum according to the procedure similar to OECD guideline 487. Cells were treated with ZnO nanoparticle (in the dispersed medium) at 0, 5, 10, 25, 50 and 75 μg/mL. 4 h later, cytochalasin-B was added and at 36 h, cell morphology was analyzed under a light microscope. Nuclearity was scored to determine the micronuclei (MN) detection and cytokinesis-block proliferation index (CBPI). CBPI, based on percentage of mono-, bi- and multi-nucleated cells, reflects cell toxicity and cell cycle delay. A potent aneugen, nocodazole, at a concentration of 0.02 µg/mL, was used as positive control for scoring MN. Negative control culture samples were exposed to Dulbecco’s Modified Eagle’s (DMEM) with 10% or 0% fetal bovine serum (FBS). A statistically significant decrease of CBPI was found for ZnO nanoparticle suspended in medium in the absence or presence of 10% serum at 25 µg/mL and 50 µg/mL, respectively. No significant increase in micronucleus was shown for ZnO nanoparticle, except at the highest dose tested (50 µg/mL) in the presence of 10% serum, where high toxicity was observed. Genotoxicity induced by exposure to ZnO nanoparticle therefore appears to be an indirect consequence of high levels of cell killing. Under the test conditions, ZnO nanoparticles did not cause significant increase in micronucleus except at the highest dose tested, where high toxicity was observed. Therefore, genotoxicity induced by exposure to ZnO appears to be an indirect consequence of high levels of cell killing.
1.4 in vitro mammalian clastogenicity assays (CA)
Ziemann (2010) conducted an in vitro mammalian chromosome aberration test with V79 cells to assess the potential of uncoated nanoscaled ZnO (Z-COTE) to induce structural (and numerical) chromosome aberrations in somatic mammalian cells according to the OECD guideline 473 in compliance with GLP. V79 Chinese hamster lung fibroblast cells were exposed to the test substance for 4 h both in the absence and presence of metabolic activation system. An initial experiment on cytotoxicity was conducted to determine the concentrations of the test substance to be used in main experiment. Uncoated ZnO nanoparticlesone concentration per main experiment was used, with 10 µg/mL for the short term experiment without S9-mix, 12.5 µg/mL for the short term experiment with S9-mix, and 3.0 µg/mL for the long term experiment without S9-mix, respectively. There was a decrease in M.I. for uncoated nanoscaled ZnO, primarily without S9-mix. Test performance and activity of the metabolizing system were satisfactory. Irrespective of its cytotoxic potential in the absence of S9-mix,uncoated nanoscaled ZnO, under all treatment modalities, did not increase significantly the aberration frequency, as compared to the respective vehicle controls. Besides some gaps, which were also evident in the negative and vehicle controls, a sum of only 4 chromosome breaks was observed in all 3 main experiments. The aberration frequencies (without gaps) in no case exceeded 5 % and they all fell within the range of the historical negative controls. Under the test conditions, nanoscaled uncoated ZnO is considered not to induce structural chromosome aberrations in cultured mammalian somatic cells.
Ziemann (2010) conducted an in vitro mammalian chromosome aberration test with V79 cells to assess the potential of coated nanoscaled ZnO (Z-COTE HP) to induce structural (and numerical) chromosome aberrations in somatic mammalian cells according to the OECD guideline 473 in compliance with GLP. V79 Chinese hamster lung fibroblast cells were exposed to the test substance for 4 h both in the absence and presence of metabolic activation system. An initial experiment on cytotoxicity was conducted to determine the concentrations of the test substance to be used in main experiment. Based on its result, three concentrations (short term experiments both without and with S9-mix) or four concentrations (long term experiment, without S9-mix) of nanoscaled were finally analysed for structural chromosome aberrations. In the main experiments without S9-mix,coated nanoscaled ZnO mediated concentration-dependent cytotoxicity, as determined by the mitotic index (M.I.). With S9-mix a significant reduction of the mean M.I. to 44.5 % of the negative control was only seen at the highest coated nanoscaled ZnO concentration used (50 µg/mL). Test performance and activity of the metabolizing system were satisfactory. Irrespective of its cytotoxic potential in the absence of S9-mix,coated nanoscaled ZnO, under all treatment modalities, did not increase significantly the aberration frequency, as compared to the respective vehicle controls. Besides some gaps, which were also evident in the negative and vehicle controls, a sum of only 4 chromosome breaks was observed in all 3 main experiments. The aberration frequencies (without gaps) in no case exceeded 5 % and they all fell within the range of the historical negative controls. Under the test conditions, nanoscaled ZnO is considered not to induce structural chromosome aberrations in cultured mammalian somatic cells.
Kwon, J.Y. et al. (2014) examined the chromosome damaging capacity of two different ZnO NP types, each prepared in two different suspensions, in Chinese hamster lung fibroblasts using the in vitro mammalian chromosomal aberration test. The experiments were performed with the four different test material suspensions at three different test concentrations (3.75, 7.5, and 15 µg/mL) both in absence and presence of a metabolic activation system. The top dose was chosen based on cytotoxicity (<50%) in a range finding test. According to the authors, the four different ZnO NP suspension did not induce structural aberration in CHL cells both in absence and presence of S9, when compared with the negative and solvent control values. Moreover, the structural aberration frequency was always below 5%. The positive control induced a marked increase in the chromosomal aberration frequency, when compared with concurrent negative controls. No conclusion can be drawn due to reporting and methodological deficiencies. The test material is insufficiently characterised, since information on purities, impurities, and surface modifications is missing. Details on the cell line, including doubling time and passage number, are missing. The concentration of the positive control substance is not specified. Mitomycin C was used as positive control substance for experiments both with and without metabolic activation; no indirect positive control substance was used. The description of the chromosome aberration assay methodology lacks details. Information on confounding factors, i.e. precipitation and cytotoxicity, in the main experiments is not provided. No additional experiment without metabolic activation was performed. The concentration is specified in µg/mL, which, however, is pointless for adherent cells. Evaluation and scoring criteria are not specified. Historical control data is missing. Uptake of the test material was not investigated. Based on the above-mentioned shortcomings the reference is considered not reliable and therefore disregarded for the hazard assessment.
The study (Gümüş et al., 2014) was conducted to investigate on potential clastogenic effects of zinc oxide nanoparticles on cultured human peripheral blood lymphocytes using the in vitro chromosomal aberrations assay. The cells were obtained from two different donors and exposed to zinc oxide nanoparticles at concentration levels of 1, 2, 5, 10, 15, and 20 µg/mL for 24 and 48 hours. Colchicine was added to each culture 2 hours before harvesting. Negative and positive (mitomycin C) control cultures were concurrently. A total of 100 metaphases per donor and concentration were scored for chromosomal aberration. In addition, the mitotic index was determined on the basis of 3000 cells. According to the authors, the 24-h exposure to zinc oxide nanoparticles resulted in a statistically significant increase of cells with chromosomal aberrations at 1, 5, 10, and 20 µg/mL, when compared to the concurrent negative control cultures. Moreover, the authors stated that the increase was concentration dependent (r=0.43). The mitotic index was statistically significantly decreased at all concentration levels. Notably, the effect on the chromosomal aberration frequency appears to be correlating with the cytotoxic response, and the highest concentration level resulted in a markedly reduced mitotic index (-53%). Thus, in absence of a strong concentration-response relationship, an absence of effects at the second highest concentration level, and increased cytotoxicity, there is no clear indication for clastogenic effects induced by zinc oxide nanoparticles under the conditions tested. After the 48-hour treatment, the chromosomal aberration frequency of zinc oxide nanoparticle-exposed lymphocytes was statistically significantly reduced only at a concentration level of 10 µg/mL. Furthermore, the response was not concentration dependent. The mitotic indices were not statistically significantly different from the concurrently control value. These results further substantiate the lack of clear indication for clastogenic activity of zinc oxide nanoparticles under the conditions of the test. In contrary, comparing the results obtained after 24 and 48 hours point into the direction of being a secondary effect caused by cytotoxicity. No conclusion can be drawn on the results presented due to major deficiencies with regards to reporting and the methodology applied. The test material was not sufficiently characterised, and the test material preparation is only poorly described. The only information given is that the test substance was prepared and characterised by Ada et al. (2008). The description of the cell slide preparation and evaluation is insufficient, since the only information given is a reference to another publication. The authors provide no information on confounding factors, such as test material precipitation or pH and osmolality effects of the test material on the culture medium. Historical control data are not included. Evaluation and scoring criteria are missing. The sex of the blood donors and the normal cell proliferation time of the lymphocytes were not specified. The type of negative control run is not specified. The authors did not examine on cellular uptake of the test material. The test material induced marked cytotoxicity after 24-hour exposure, whereas a 48-hour exposure period did not result in cytotoxicity. This observation appears to be implausible. Based on the above-mentioned shortcomings the reference is considered not reliable and therefore disregarded for the hazard assessment.
1.5 in vitro DNA damage assays (Comet assay)
Annangi et al. (2015) examined potential DNA damage Ogg1+/+ and Ogg1-/- in mouse embryonic fibroblasts (MEF) after acute and long-term ZnO NP exposure. The DNA damage was evaluated in the modified alkaline comet assay using the FPG enzyme to discriminate between general and oxidative DNA damage. The cytotoxic effect of ZnO NPs in the two MEF cell lines was evaluated in separate experiment. Moreover, ROS generation, cellular NP uptake, and ZnO NP dissolution were examined. According to the authors, ZnO NPs induced significant but transient genotoxic and oxidative DNA damage as well as significantly increased ROS levels in both cell lines treated for 24 h. In contrast, cells treated for 6 and 12 weeks showed neither significant DNA damage nor significant ROS generation at the concentration tested. Analysis of long-term exposed cells revealed no presence of ZnO NPs within cells. Further analyses revealed that ZnO NPs showed rapid dissolution and that presumably ≥50% of the ZnO NPs uptake was in form of zinc ions. No conclusion can be drawn due to reporting and methodological deficiencies. The test item is insufficiently characterised, since information on the purity, impurity elements and surface modifications are missing. Information on test material preparation lacks details. Details on the cell lines, including cell doubling time, are missing. The description of the comet assay lacks some details. Only untreated cells were utilised as negative control. No parallel cytotoxicity test was performed, and cytotoxicity evaluation was only based on a separate cell count assay which was performed under different exposure conditions. In the comet assay, the cell number analysed per sample (100) was lower than recommended in the equivalent in vivo guideline OECD TG 489 (150). The long-term treatments were performed at only one concentration (1 µg/mL). Importantly, the authors do not state removal and disposition of particles after exposure and light conditions during the comet assay, especially for the modified comet assay necessitating the additional FPG incubation step. However, the photocatalytic capacity of the ZnO NPs could have led in association with ‘naked’ DNA and light to false positives. Moreover, remaining ZnO NPs could have interfered with electrophoresis and comet scoring. Furthermore, the author did not state on precipitation and distribution of ZnO NPs during the treatment. Potential effects on pH or osmolality of the culture medium induced by ZnO NPs were not discussed. Information on comet assay evaluation criteria are unclear or missing. The authors do not state on scorable vs. non-scorable cells and hedgehog occurrence is not stated. Exposure concentrations are stated in µg/mL; however, the cells are adherent, and thus, it would be appropriate to specify exposure concentrations in µg/cm². The use of FPG in the modified comet assay is reported to increase inter-sample variability of the DNA damage response. Moreover, laboratory’s proficiency in preparing and analysing comets’ characteristics in comet assays is a prerequisite for reliable data. It was shown that there is huge variability in tail DNA intensities even within negative or positive controls (Tavares, A.M. et al. (2014); Nanogenotox Programme). The increase observed in MEF Ogg1-/- cells exposed to ZnO NPs for 24 h was apparently not concentration dependent. Similarly, the net oxidative DNA damage was not increased in a concentration dependent manner in MEF Ogg1+/+ cells. Based on the above-mentioned shortcomings the reference is considered not reliable and therefore disregarded for the hazard assessment.
The authors (Branica et al., 2016) evaluated DNA damage in ZnO NP-treated human lymphocytes after 14 days exposure using the conventional alkaline comet assay and by combining the comet assay with FISH staining (comet-FISH). Moreover, the cell viability was evaluated using acridine orange/ethidium bromide stain. The authors concluded that primary DNA damage (conventional alkaline comet assay) increased with NP concentration in unseparated lymphocytes and high-density lymphocytes, resulting from density gradient separation. Similarly, the comet-FISH assay showed increased fragmentation of the TP53 gene. NP accumulation was significant only with the two highest concentrations, as indicated by measurements of intracellular Zn2+ concentrations. Moreover, the authors stated that the toxic effects correlate with the Zn2+ concentrations and intracellular Zn2+ concentrations, in turn, correlate with the ZnO NP concentration used in the assay. Furthermore, the authors speculated that there are differences in the accumulation capacity between lymphocytes at ZnO NP concentrations above 2.5 µg/mL resulting in the two different lymphocyte populations after density gradient separation. Notably, the authors stated that it remains questionable whether and how complexed Zn2+ ions have interfered with ability of damaged DNA to migrate, resulting in artificial responses. No conclusion can be drawn due to reporting and methodological deficiencies. The test item is only insufficiently characterized, since information on purity, surface modifications, and particle size distribution are missing. Moreover, reporting on the test material solution lacks details. The number of cells scored for DNA damage was too low, since only 60 cells were evaluated per treatment (>150 are recommended by the equivalent in vivo guideline OECD T489). The performance of the comet assay is insufficiently described. Importantly, the authors do not state removal and disposition of particles after exposure and light conditions during the comet assay. However, the photocatalytic capacity of the ZnO NPs could have led in association with ‘naked’ DNA and light to false positives. Moreover, remaining ZnO NPs could have interfered with electrophoresis and comet scoring. The description of the treatment includes no information on the number of cells treated and the medium used for cell culture. Precipitation of the test item as well as potential effects on osmolality and pH on the culture medium are not discussed. Direct information on cellular uptake and intracellular localisation of ZnO NPs are missing. Evaluation and acceptability criteria were not specified. Moreover, laboratory’s proficiency in preparing and analysing comets’ characteristics in comet assays is a prerequisite for reliable data. It was shown that there is huge variability in tail DNA intensities even within negative or positive controls (Tavares, A.M. et al. (2014); Nanogenotox Programme). In the conventional alkaline comet assay, no increased DNA damage was observed in unseparated and low-density lymphocytes. However, the DNA damage observed in high-density lymphocytes was only observed at concentrations which also compromised significantly the cell viability.
El Yamani et al. (2017) tested the DNA damaging capacity of ZnO NM110 by using a high throughput comet assay system. A549 and TK6 cells were treated with ZnO NM110 at a range of concentrations for 3 or 24 h. An alkaline comet assay was performed with cells treated with or without formamidopyrimidne-DNA glycosylase (Fpg) enzyme, which detects oxidised bases. Afterwards, the amount of DNA in the tail was determined. Cytotoxicity was determined using colony forming efficiency (CFE) assay and the colorimetric alamarBlue® assay. According to the authors, ZnO NM110 induced high levels of DNA damage in TK6 cell at cytotoxic concentrations after 3 h of exposure, whereas no significant damage was observed in the prolonged treatment. The authors stated that the DNA damage was presumably secondary to toxicity. In A549 cells, there was general and oxidative DNA damage observed at both cytotoxic and non-cytotoxic concentrations after 3 h and 24 h of exposure. The oxidative damage was decreased in after prolonged exposure. The cytotoxicity assays revealed that ZnO NM110 showed cytotoxic and cytostatic effects already at low and moderate concentrations in A549 cells. No conclusion can be drawn due to reporting and methodological deficiencies. The test material is not characterised sufficiently. Purity, impurity elements, and information on surface modifications are not specified. Details on the performance of the comet assay are missing- Importantly, the authors do not state removal and disposition of particles after exposure and light conditions during the comet assay. However, the photocatalytic capacity of the ZnO NPs could have led in association with ‘naked’ DNA and light to false positives, especially for the modified comet assay necessitating the additional Fpg incubation step. Moreover, remaining ZnO NPs could have interfered with electrophoresis and comet scoring. Furthermore, the author did not state on precipitation and distribution of ZnO NPs during the treatment, which could be an important especially in the case of adherent cells, covered by particles. Potential effects on pH or osmolality of the culture medium induced by ZnO NM110 were not discussed. Information on comet assay evaluation criteria are unclear or missing. The authors do not state on scorable vs. non-scorable cells and hedgehog occurrence is not stated. Details on statistical analysis are missing. Performance of a trend test was not specified. There are no statements on the normality of homogeneity of variances of the analysed data. A vehicle control was not used in the assay; the only negative controls were untreated cells. Cytotoxicity assays were only performed for the A549 cells but not for the TK6 cells. Measurement of cytotoxicity is essential to interpret the findings, since cytotoxicity is reported to be a confounder of comet assay potentially leading to false positives. Moreover, the alamarBlue® assay is known to be prone to interference of NPs. Interference with the assay was not discussed and could have potentially led to errors in cytotoxicity determinations. The authors used lesion-specific endonucleases to reveal oxidative DNA damages. However, the use of FPG in the modified comet assay is reported to increase inter-sample variability of the DNA damage response. The number of analysed cells is too low, the OECD test guideline for in vivo testing recommends scoring of at least 150 randomly selected cells. Information on cellular uptake and intracellular localisation of ZnO NPs are missing. Evaluation and acceptability criteria were not specified. Moreover, laboratory’s proficiency in preparing and analysing comets’ characteristics in comet assays is a prerequisite for reliable data. It was shown that there is huge variability in tail DNA intensities even within negative or positive controls (Tavares, A.M. et al. (2014); Nanogenotox Programme). In TK6 cells, ZnO NM110 induced no statistically significant increase in SBs after 24 h exposure, according to the authors, 3 h exposure led only in presence of cytotoxicity to DNA damage. Similarly, in A549 cells, the conventional comet assay revealed ZnO NM110-induced DNA damage only in the presence of cytotoxicity (at a concentration of 10 µg/cm² and above), as indicated by the alamarBlue® and CFE assays. Moreover, the CFE assay showed ZnO NM110 already at a concentration of 0.3 µg/cm² to be cytotoxic. Thus, there is only one ZnO NM110 concentration tested (0.1 µg/cm²) which is not cytotoxic with regard to the CFE assay. Based on the above-mentioned shortcomings the reference is considered not reliable and therefore disregarded for the hazard assessment.
Hackenberg et al. (2011) examined DNA damage and repair after repeated ZnO NP exposure of human nasal mucosal mini cultures (MOCs). The DNA damage and repair were evaluated in a conventional single-cell comet assay. Additionally, cell viability, apoptosis, ROS generation, and cellular localisation of NP were analysed. According to the authors, ZnO NPs particles induced DNA damage only in MOCs treated at 5 µg/mL after treatment for 1, 2, and 3 h. Moreover, significantly increased DNA damage was observed in the 24 h regeneration groups treated at both concentrations. The authors hypothesised that the increased DNA damage in these groups is due to persisting ZnO NPs, which induce further DNA damage. Cell viability and apoptosis were not affected under the conditions tested. Further, the ZnO NP induced ROS generation in human nasal mucosal cells at both concentrations, as determined via a qualitative assay. TEM analysis revealed particles which were localised in the cytoplasm and sporadically in the nucleus of the cells. No conclusion can be drawn due to reporting and methodological deficiencies. The test item is insufficiently characterised, since information on the impurity elements and potential surface modifications are missing. The comet assay was only performed with untreated control, a vehicle control is missing. The test system were mini organ cultures obtained from ten different patient during surgery in the nasal airways. The authors, however, do not state which sample was used for which test group and whether this could have impacted on the outcome of the comet. Since the patients are a heterogenous group (male and females, age range 20-87 years), a potential effect on the grade of response cannot be excluded. It is not specified whether particles were still present after lysis. Remnant NPs could have potentially interfered with electrophoresis and scoring. The authors reported on a sporadically nuclear localisation of the ZnO NP. It remains, however, unclear how NP aggregates of a size up to 1106 nm (mean: 354 nm) could have entered the nucleus. Information on cell proliferation is not provided. The comet assay was performed only at two concentrations, and thus, a potential concentration-response relationship cannot be derived. Details on the cytotoxicity results are missing; no raw data is included. Negative controls in the ROS experiments are missing. The authors do not state on existence of test item precipitates and potential changes in pH and osmolality and test item treated cell medium. Three parameters were analysed to assess DNA damage (% DNA in tail, tail length, OTM), a justification for the selected parameter is, however, missing. The number of analysed cells is too low, the OECD test guideline for in vivo testing recommends scoring of at least 150 randomly selected cells. Evaluation and acceptability criteria were not specified. Moreover, laboratory’s proficiency in preparing and analysing comets’ characteristics in comet assays is a prerequisite for reliable data. It was shown that there is huge variability in tail DNA intensities even within negative or positive controls (Tavares, A.M. et al. (2014); Nanogenotox Programme). Based on the above-mentioned shortcomings the reference is considered not reliable and therefore disregarded for the hazard assessment.
Hackenberg et al. (2017) investigated on potential DNA damage and repair after ZnO NP exposure in human nasal mucosal cell monolayers (at the air-liquid interface) and mini organ cultures (MOCs). DNA damage and repair were investigated via conventional alkaline comet assays. Moreover, cell viability was assessed via the colorimetric MTT assay. Transmission electron microscopy was used to examine the cellular distribution of the ZnO NPs. According to the authors, ZnO NPs induced statistically significant increases in DNA damage in human nasal mucosal cell monolayers at both concentrations tested (0.1 and 1.0 µg/mL). In contrast, in human nasal mucosal mini organ cultures, statistically significant increases in DNA damage were only observed at 1.0 µg/mL. However, MOCs exposed to both concentrations for one hour showed increased DNA damage after the 24-h particle-free regeneration time. The concentration used in the comet assay were not cytotoxic as indicated by the MTT assay. Moreover, the author found no interference of the ZnO NPs with the MTT in a control experiment. The TEM analysis revealed uptake of ZnO NPs via endocytosis. Notably, the cell nuclei were devoid of any ZnO NPs. No conclusion can be drawn due to reporting and methodological deficiencies. The test item is insufficiently characterised, since information on the impurity elements and potential surface modifications are missing. The methodology of the comet assay is insufficiently described- Importantly, the authors do not state removal and disposition of particles after exposure and light conditions during the comet assay. However, the photocatalytic capacity of the ZnO NPs could have led in association with ‘naked’ DNA and light to false positives. The comet assay was only performed with untreated control, a vehicle control is missing. Remnant NPs could have potentially interfered with electrophoresis and scoring. The comet assay was performed only at two concentrations, and thus, a potential concentration-response relationship cannot be derived. Details on the cytotoxicity results are missing; no raw data is included. The authors do not state on existence of test item precipitates and potential changes in pH and osmolality of the test item treated cell medium. The number of analysed cells is too low, the OECD test guideline for in vivo testing recommends scoring of at least 150 randomly selected cells. Three parameters were analysed to assess DNA damage (% DNA in tail, tail length, OTM), a justification for the selected parameter is, however, missing. Moreover, it is not clear which parameters was actually evaluated in which experiments, since information given in the comet assay description, statistical analysis, main text and figures are contradictory. Further, in the results section is stated that 10 µg/mL ZnO NPs induced significant DNA damage in MOC, whereas, in the figure and material and methods section, it stated that the test material was only tested at 0.1 and 1 µg/mL. Evaluation and acceptability criteria were not specified. Moreover, laboratory’s proficiency in preparing and analysing comets’ characteristics in comet assays is a prerequisite for reliable data. It was shown that there is huge variability in tail DNA intensities even within negative or positive controls (Tavares, A.M. et al. (2014); Nanogenotox Programme). Based on the above-mentioned shortcomings the reference is considered not reliable and therefore disregarded for the hazard assessment.
Kermanizadeh et al. (2013) analysed the capacity to induce DNA damage of two different ZnO NP types in adult human renal proximal tubule epithelial cells (HK-2) using the modified comet assay. The authors examined the DNA damage in both absence and presence of FPG to analyse DNA strand breaks and specific oxidative DNA damage. Furthermore, cytotoxicity, ROS generation, and cytokine expression were evaluated after ZnO NP treatment. According to the authors, significant DNA damage was observed only after the treatment with ZnO NM100 in absence of FPG. Moreover, ZnO NM110 and ZnO NM111 showed increased cytotoxic effects on HK-2 cells in both media. Furthermore, both particles induced a significantly increased ROS generation at the two highest concentrations tested. The expression of the cytokines, IL6 and IL8, was significantly increased at higher ZnO NP concentrations. No conclusion can be drawn due to reporting and methodological deficiencies. The test item is insufficiently characterised, since information on the purity and impurity elements are missing. The comet assay was only performed with untreated control, a vehicle control is missing. The description of the methodology lacks details. Importantly, the authors do not state removal and disposition of particles after exposure and light conditions during the comet assay. However, the photocatalytic capacity of the ZnO NPs could have led in association with ‘naked’ DNA and light to false positives, especially for the modified comet assay necessitating the additional Fpg incubation step. Moreover, remaining ZnO NPs could have interfered with electrophoresis and comet scoring. The use of FPG in the modified comet assay is reported to increase inter-sample variability of the DNA damage response. Furthermore, the author did not state on precipitation and distribution of ZnO NPs during the treatment, which could be an important especially in the case of adherent cells, covered by particles. Potential effects on pH or osmolality of the culture medium induced by ZnO NPs were not discussed. Information on comet assay evaluation criteria are unclear or missing. The authors do not state on scorable vs. non-scorable cells and hedgehog occurrence is not stated. The authors do not state on potential changes in pH and osmolality of the test item treated cell medium. Moreover, laboratory’s proficiency in preparing and analysing comets’ characteristics in comet assays is a prerequisite for reliable data. It was shown that there is huge variability in tail DNA intensities even within negative or positive controls (Tavares, A.M. et al. (2014); Nanogenotox Programme). The information on the parameter analysed to evaluate DNA damage is contradictory (tail moment vs. DNA in tail). Analyses of test material uptake were not performed. Cytotoxicity was measured using the colorimetric WST-1 assay. However, the cytotoxicity was evaluated in separate experiment under different exposure conditions (24 and 48 h vs 4h in the comet assay). Moreover, a potential interference of the test item with the colorimetric assay is not discussed. ZnO NM110 resulted in a statistically significant increase in DNA damage only in the absence of FPG enzyme. Notably, the response was only marginal and not concentrations-related over three different concentrations. Moreover, it appears that this significance is more due to a relatively low control value (compared to the experiments with other NPs) than due to a meaningful test item-related increase. Based on the above-mentioned shortcomings the reference is considered not reliable and therefore disregarded for the hazard assessment.
Kermanizadeh et al. (2012) analysed the capacity to induce DNA damage of two different ZnO NP types in a human hepatocyte cell line (C3A) using the modified comet assay. The authors examined the DNA damage in both absence and presence of FPG to analyse DNA strand breaks and specific oxidative DNA damage. Moreover, ROS generation and GSH depletion were evaluated after ZnO NP treatment. According to the authors, both ZnO NPs induce a small but significant increase in DNA damage in C3A cells after a 4-h exposure period. In the WST-1 cytotoxicity, both NP types were highly cytotoxic in C3 cells. Moreover, both NP types induced a significant GSH depletion, whereas the intracellular ROS levels were not or only slightly increased. No conclusion can be drawn due to reporting and methodological deficiencies. The test item is insufficiently characterised, since information on the purity and impurity elements are missing. The comet assay was only performed with untreated control, a vehicle control is missing. The description of the methodology lacks details. Importantly, the authors do not state removal and disposition of particles after exposure and light conditions during the comet assay. However, the photocatalytic capacity of the ZnO NPs could have led in association with ‘naked’ DNA and light to false positives, especially for the modified comet assay necessitating the additional Fpg incubation step. Moreover, remaining ZnO NPs could have interfered with electrophoresis and comet scoring. The use of FPG in the modified comet assay is reported to increase inter-sample variability of the DNA damage response. Furthermore, the author did not state on precipitation and distribution of ZnO NPs during the treatment, which could be an important especially in the case of adherent cells, covered by particles. Potential effects on pH or osmolality of the culture medium induced by ZnO NPs were not discussed. Information on comet assay evaluation criteria are unclear or missing. The authors do not state on scorable vs. non-scorable cells and hedgehog occurrence is not stated. Moreover, laboratory’s proficiency in preparing and analysing comets’ characteristics in comet assays is a prerequisite for reliable data. It was shown that there is huge variability in tail DNA intensities even within negative or positive controls (Tavares, A.M. et al. (2014); Nanogenotox Programme). The information on the parameter analysed to evaluate DNA damage is contradictory (tail moment vs. DNA in tail). Analyses of test material uptake were not performed. Cytotoxicity was determined only in a separate experiment in a different publication and was measured using the colorimetric WST-1 assay. However, the cytotoxicity was evaluated under different exposure conditions (24 h vs 4h in the comet assay). Moreover, a potential interference of the test item with the colorimetric assay is not discussed. The proportion of DNA in tail was statistically significantly increased after exposure to 1.25 and 2.5 µg/cm² ZnO NM100 in presence of FPG. However, the increase was only small and showed not concentration-response relationship. In the case of ZnO NM111, a statistically significant increase was only observed at the highest concentration (10 µg/cm²), which is also associated with increased cytotoxicity (LC50 value). Thus, the biological significance of these findings is questionable. Based on the above-mentioned shortcomings the reference is considered not reliable and therefore disregarded for the hazard assessment.
Ickrath et al. (2017) investigated on the DNA damaging capacity of ZnO NPs in primary human adipose tissue-derived mesenchymal stem cells after prolonged and repetitive exposure. The DNA damage was evaluated using the conventional comet assay under alkaline conditions. The colorimetric MTT assay was utilised to evaluate the cytotoxicity of the ZnO NP under the same exposure conditions. Moreover, cellular uptake of ZnO NPs was qualitatively analysed using TEM. According to the authors, ZnO NPs induced significant DNA damage in cells exposed to 1 and 10 µg/mL for 24 h compared with controls. In contrast, repetitive exposure resulted not in significantly increased DNA damage under the conditions tested. Notably, the concentrations tested in both experiments were not causing cytotoxicity in human adipose tissue-derived mesenchymal stem under the same exposure conditions, as determined via the MTT assay. The TEM analysis revealed cellular uptake of the ZnO NPs already after short incubation intervals. Long-term evaluation revealed the persistence of the ZnO NPs in the cells which were in rare cases also localised to the nucleus. No conclusion can be drawn due to reporting and methodological deficiencies. The test item is insufficiently characterised, since information on the impurity elements and surface modifications are missing. The number of cells scored for DNA damage was too low, since only 50 cells were evaluated per replicate (>150 are recommended by the equivalent in vivo guideline OECD T489). The comet assay was only performed with untreated control, a vehicle control is missing. The methodology of the comet assay is only poorly described. Importantly, the authors do not state removal and disposition of particles after exposure and light conditions during the comet assay. However, the photocatalytic capacity of the ZnO NPs could have led in association with ‘naked’ DNA and light to false positives. Moreover, remaining ZnO NPs could have interfered with electrophoresis and comet scoring. Furthermore, the author did not state on precipitation and distribution of ZnO NPs during the treatment, which could be an important especially in the case of adherent cells, covered by particles. Potential effects on pH or osmolality of the culture medium induced by ZnO NPs were not discussed. Exposure concentrations are stated in µg/mL; however, the cells are adherent, and thus, it would be appropriate to specify exposure concentrations in µg/cm². Details on the donors are missing. Information on cell proliferation are not provided. Information on comet assay evaluation criteria are unclear or missing. The authors do not state on scorable vs. non-scorable cells and hedgehog occurrence is not stated. Moreover, laboratory’s proficiency in preparing and analysing comets’ characteristics in comet assays is a prerequisite for reliable data. It was shown that there is huge variability in tail DNA intensities even within negative or positive controls (Tavares, A.M. et al. (2014); Nanogenotox Programme). The results of the negative and positive control are not shown for the cytotoxicity and comet assay, respectively. Intracellular Zn2+ concentration was not tested. Based on the above-mentioned shortcomings the reference is considered not reliable and therefore disregarded for the hazard assessment.
Sharma et al. (2011) examined potential DNA damage after the exposure of primary human epidermal keratinocytes to ZnO NPs. The DNA damage was evaluated in a conventional alkaline comet assay and was specified in olive tail moment, DNA in tail, and tail length. Concurrently, cytotoxicity was determined using the trypan blue exclusion test. Moreover, the MTT assay was utilised, in a separate experiment, to evaluate cytotoxicity. Cellular uptake of the test material was analysed qualitatively using SEM and TEM.
According to the authors, exposure to ZnO NPs resulted in a significant induction in DNA damage at concentrations of 8 and 14 µg/mL compared to the negative control. These concentrations elicited no cytotoxic effects as indicated via the MTT assay and trypan exclusion test. However, treatment with ZnO NPs resulted in a concentration- and time-dependent increase in cytotoxicity as evidenced by the MTT assay. Microscopical analyses (SEM and TEM) revealed cellular uptake of the ZnO NPs. No conclusion can be drawn due to reporting and methodological deficiencies. The test item is insufficiently characterised, since information on the impurity elements and surface modifications are missing. The number of cells scored for DNA damage was too low, since only 50 cells were evaluated per experiment (>150 are recommended by the equivalent in vivo guideline OECD T489). The methodology of the comet assay lacks details. Importantly, the authors do not state removal and disposition of particles after exposure and light conditions during the comet assay. However, the photocatalytic capacity of the ZnO NPs could have led in association with ‘naked’ DNA and light to false positives. Moreover, remaining ZnO NPs could have interfered with electrophoresis and comet scoring. Furthermore, the author did not state on precipitation and distribution of ZnO NPs during the treatment, which could be an important especially in the case of adherent cells, covered by particles. Potential effects on pH or osmolality of the culture medium induced by ZnO NPs were not discussed. Exposure concentrations are stated in µg/mL; however, the cells are adherent, and thus, it would be appropriate to specify exposure concentrations in µg/cm². Information on comet assay evaluation criteria are unclear or missing. The authors do not state on scorable vs. non-scorable cells and hedgehog occurrence is not stated. Potential interference of NPs with colorimetric MTT assay was not discussed. Moreover, laboratory’s proficiency in preparing and analysing comets’ characteristics in comet assays is a prerequisite for reliable data. It was shown that there is huge variability in tail DNA intensities even within negative or positive controls (Tavares, A.M. et al. (2014); Nanogenotox Programme). Intracellular Zn2+ concentration was not tested. Based on the above-mentioned shortcomings the reference is considered not reliable and therefore disregarded for the hazard assessment.
Sharma, M. et al. (2009) investigated on the potential of ZnO NPs to induce DNA damage in A431 human epidermal cells. A conventional alkaline comet assay was performed in order to examine the DNA damage, which specified in proportion of DNA in tail and OTM (arbitrary categories). The cytotoxicity was concurrently determined evaluated in a trypan blue exclusion test. Additionally, MTT reduction, LDH release, and neutral red uptake (NRU) were analysed as measure for cytotoxicity. The oxidative activity of the ZnO NPs in the test system was evaluated using different parameter, which were glutathione levels, lipid peroxidation, catalase activity, and superoxide dismutase activity. According to the authors, ZnO NP treatment induced significant DNA damage starting at a concentration of 0.8 µg/mL, when compared with untreated controls. Notably, the authors stated that the concentration tested induced no cytotoxicity as determined via the trypan blue exclusion test and as indicated by the other cytotoxicity test utilised. Moreover, oxidative stress markers were significantly affected after 24 h exposure to ZnO NPs. No conclusion can be drawn due to reporting and methodological deficiencies. The test item is insufficiently characterised, since information on the impurity elements and surface modifications are missing. The number of cells scored for DNA damage was too low, since only 50 cells were evaluated per replicate (>150 are recommended by the equivalent in vivo guideline OECD T489). The comet assay was only performed with untreated control, a vehicle control is missing. The description of the methodology lacks details. Importantly, the authors do not state removal and disposition of particles after exposure and light conditions during the comet assay. However, the photocatalytic capacity of the ZnO NPs could have led in association with ‘naked’ DNA and light to false positives. Moreover, remaining ZnO NPs could have interfered with electrophoresis and comet scoring. Furthermore, the author did not state on precipitation and distribution of ZnO NPs during the treatment, which could be an important especially in the case of adherent cells, covered by particles. Potential effects on pH or osmolality of the culture medium induced by ZnO NPs were not discussed. The others did not investigate on cellular uptake and distribution of the ZnO NPs. Exposure concentrations are stated in µg/mL; however, the cells are adherent, and thus, it would be appropriate to specify exposure concentrations in µg/cm². The authors classified the OTMs in arbitrary units, but do not state on criteria for this classification. Information on comet assay evaluation criteria are unclear or missing. The authors do not state on scorable vs. non-scorable cells and hedgehog occurrence is not stated. Moreover, laboratory’s proficiency in preparing and analysing comets’ characteristics in comet assays is a prerequisite for reliable data. It was shown that there is huge variability in tail DNA intensities even within negative or positive controls (Tavares, A.M. et al. (2014); Nanogenotox Programme). The information on the parameter analysed to evaluate DNA damage is contradictory (tail moment vs. DNA in tail). Analyses of test material uptake were not performed. Interference of ZnO NPs with the colorimetric cytotoxicity assays is not discussed. The increase in the proportion of DNA in tail is only slight at the highest concentrations tested (8±0.71 vs 10.6±0.76 and 13±1.50), when compared to untreated controls. Based on the above-mentioned shortcomings the reference is considered not reliable and therefore disregarded for the hazard assessment.
Sliwinska et al. (2015) examined DNA damage in human peripheral lymphocytes after ZnO NP treatment. The DNA damage was evaluated in a set of different comet assay types. Cytotoxicity was evaluated in the colorimetric MTT assay. Furthermore, apoptosis was evaluated via FACS. According to the authors, ZnO NP treatment resulted in significant and concentration dependent increases of DNA damage in human peripheral lymphocytes, in comet assays performed at pH 12.1 and >13. In the neutral comet assay, the increase in DNA damage was statistically significant at the two highest concentrations tested, when compared to untreated controls. Moreover, additional treatment with DNA glycosylase Endo III and FPG showed significantly higher DNA damage, when compared to samples without enzyme treatment, indicating oxidative DNA damage induced by the ZnO NP treatment. Further, comet assay performed after a particle-free regeneration period, indicated significantly decreased DNA damage after 2 h. However, the DNA damage was still higher in high-dose cultures after the 2-h regeneration interval, when compared to untreated controls. No conclusion can be drawn due to reporting and methodological deficiencies. The test item is insufficiently characterised, since information on the purity, impurity elements and surface modifications are missing. Information on test material preparation lacks information, since no information on the particle size distribution in the cell medium is provided. Details on the cell sample, including age of each donor and whether the cells were pooled, are missing. In the comet assays, the cell number analysed per sample (100) was lower than recommended in the equivalent in vivo guideline OECD TG 489 (150). Importantly, the authors do not state removal and disposition of particles after exposure Remaining ZnO NPs could have interfered with electrophoresis and comet scoring. Furthermore, the author did not state on precipitation and distribution of ZnO NPs during the treatment. Potential effects on pH or osmolality of the culture medium induced by ZnO NPs were not discussed. Information on comet assay evaluation criteria are unclear or missing. The authors do not state on scorable vs. non-scorable cells and hedgehog occurrence is not stated. Potential interference of NPs with colorimetric MTT assay was not discussed. The use of FPG in the modified comet assay is reported to increase inter-sample variability of the DNA damage response. Moreover, laboratory’s proficiency in preparing and analysing comets’ characteristics in comet assays is a prerequisite for reliable data. It was shown that there is huge variability in tail DNA intensities even within negative or positive controls (Tavares, A.M. et al. (2014); Nanogenotox Programme). Cellular uptake was not investigated. In the neutral comet assay, statistically significant increases were observed only in presence of significant cytotoxicity. Based on the above-mentioned shortcomings the reference is considered not reliable and therefore disregarded for the hazard assessment.
Demir et al. (2014) performed comet assays to evaluate DNA damage in HEK293 and NIH/3T3 cells after treatment with two different ZnO NP types. Concurrently, cell viability was evaluated using a stain discriminating between live and dead cells. According to the authors, treatment with the ZnO NPs did not result in a significant decrease in cell viability. In the conventional comet assay, the proportion of DNA in tail and the tail length were significantly increased in both cell lines treated with either ZnO NP type at concentrations of 100 and 1000 µg/mL. In contrast, the ZnO bulk reference showed no significant increase in the DNA damage parameters in either cell line. In the modified comet assay, using either FPG or EndoIII enzyme, both ZnO NP types induced significantly DNA damage at 100 and 1000 µg/mL in both cell lines in experiments with either FPG or EndoIII as well as in buffer-only control experiments. However, the net oxidative damage was not significantly different under any condition in cells treated with either ZnO NP type. Again, the treatment with the ZnO bulk reference induced no significant DNA damage under any condition tested. No conclusion can be drawn due to reporting and methodological deficiencies. The test item is insufficiently characterised, since information on the purity, impurity elements and surface modifications are missing. Details on the cell lines, including cell doubling time, are missing. The description of the comet assay lacks some details. Importantly, the authors do not state removal and disposition of particles after exposure. Remaining ZnO NPs could have interfered with electrophoresis and comet scoring. Furthermore, the author did not state on precipitation and distribution of ZnO NPs during the treatment. Especially treatment at excessively high concentrations >500 µg/mL may have resulted in particle overload. Potential effects on pH or osmolality of the culture medium induced by ZnO NPs were not discussed. Information on comet assay evaluation criteria are unclear or missing. The authors do not state on scorable vs. non-scorable cells and hedgehog occurrence is not stated. Exposure concentrations are stated in µg/mL; however, both cell lines are composed of adherent cells, and thus, it would be appropriate to specify exposure concentrations in µg/cm². The use of DNA glycosylases in the modified comet assay is reported to increase inter-sample variability of the DNA damage response. Moreover, laboratory’s proficiency in preparing and analysing comets’ characteristics in comet assays is a prerequisite for reliable data. It was shown that there is huge variability in tail DNA intensities even within negative or positive controls (Tavares, A.M. et al. (2014); Nanogenotox Programme). Cellular uptake of the test material was not investigated. Based on the above-mentioned shortcomings the reference is considered not reliable and therefore disregarded for the hazard assessment.
Jain, A.K. et al. (2018) investigated on potential DNA damage after ZnO NP treatment of V79 (Chinese hamster fibroblasts) cells using the conventional alkaline comet assay. Moreover, cytotoxicity was analysed utilising the MTT assay as well as by analysis of apoptosis and cell cycle. In addition, cellular uptake and ROS generation were analysed. According to the authors, ZnO NPs induced significant and concentration-dependent DNA damage in V79 cells treated for 6 h starting at concentrations of 5 and 10 µg/mL as represented by increased in the proportion of DNA in tail and OTM, respectively. Moreover, cytotoxic effects were observed at concentrations of 15-20 and 10-20 µg/mL in cells treated for 6 and 24 h, respectively, as evidenced by the MTT assay. Moreover, induction of apoptosis and increased proportions of cells in the G2-M cell cycle phase by a 24-h ZnO NP treatment were observed in further analyses. Further, a significant concentration-dependent cellular uptake of test material was observed in cells treated for 24 h. The particles were predominantly found in vesicles but also sporadically in the nucleus. Intracellular ROS levels were significantly increased in cells exposed for 6 and 24 h to concentrations of 10 µg/mL and above. The increase showed a concentration-related response. No conclusion can be drawn due to reporting and methodological deficiencies. Description of the comet methodology lacks some details, since information on temperature and V/cm during electrophoresis and staining time is missing. Importantly, the authors do not state removal and disposition of particles after exposure and light conditions during the comet assay. However, the photocatalytic capacity of the ZnO NPs could have led in association with ‘naked’ DNA and light to false positives. Moreover, remaining ZnO NPs could have interfered with electrophoresis and comet scoring. Only 100 cells were scored for DNA damage per treatment, which is less than required by the equivalent in vivo test guideline (OECD TG 489, 2016). No positive control was included. Cytotoxicity was not tested in parallel, but only in a separate experiment utilising the colorimetric MTT assay. Details on the cell line, including cell doubling time, number of passages, and potential mycoplasma infection are missing. Furthermore, the author did not state on precipitation and distribution of ZnO NPs during the treatment. Potential effects on pH or osmolality of the culture medium induced by ZnO NPs were not discussed. Information on comet assay evaluation criteria are unclear or missing. The authors do not state on scorable vs. non-scorable cells and hedgehog occurrence is not stated. Exposure concentrations are stated in µM and µg/mL; however, the cell line is composed of adherent cells, and thus, it would be appropriate to specify exposure concentrations in µg/cm². Moreover, laboratory’s proficiency in preparing and analysing comets’ characteristics in comet assays is a prerequisite for reliable data. It was shown that there is huge variability in tail DNA intensities even within negative or positive controls (Tavares, A.M. et al. (2014); Nanogenotox Programme). ZnO NP dissolution and Zn ion effects on the cells are neither examined nor discussed. Based on the above-mentioned shortcomings the reference is considered not reliable and therefore disregarded for the hazard assessment.
Kononenko, V., et al. (2017) investigated on the DNA damaging capacity of three different test materials in in the MDCK (Madin-Darby Canine Kidney) cell line. The DNA damage was evaluated in a conventional alkaline comet assay. Moreover, cytotoxicity was investigated utilising the MTT, NRU, and trypan blue exclusion assays. In further experiments, the authors examined ROS generation, ZnO NP dissolution, and the activity of catalase and glutathione-S-transferase (GST) after test material treatment. According to the authors, the comet assay results indicated a statistically significant increase in double- and single-strand DNA breaks in cells exposed to ZnO NP at concentrations of 61 and 123 µM, whereas ZnO macroparticles (MPs) and ZnCl2 did not. Moreover, the test materials induced statistically significant cytotoxicity at concentration of 184 µM in the MTT and NRU assay, whereas the trypan blue exclusion assay indicated considerable cytotoxicity at concentrations of 360 µM and above. GST activity was statistically significantly decreased only after ZnO NP treatment, whereas catalase activity was reduced in treatment with both ZnO NPs and ZnCl2. However, catalase activity was only slightly impaired after ZnCl2 treatment. Acellular measurement of ROS indicated no significant in ROS levels in cell culture media after the 24 h incubation with either ZnO NPs, ZnO MPs, or ZnCl2. No conclusion can be drawn due to reporting and methodological deficiencies. The test item is insufficiently characterised, since information on the purity, impurity elements and surface modifications are missing. Description of test material preparation and comet methodology lacks some details. Only 60 cells were scored for DNA damage per experiment, the number of experiments was, however, comparatively high (n=6). Cytotoxicity was not tested in parallel. The comet assay was performed in a cell line which is non-standard for genotoxicity testing. Details on the cell lines, including cell doubling time, are missing. The authors do not state removal and disposition of particles after exposure. Remaining ZnO NPs could have interfered with electrophoresis and comet scoring. Furthermore, the author did not state on precipitation and distribution of ZnO NPs during the treatment. Potential effects on pH or osmolality of the culture medium induced by ZnO NPs were not discussed. Information on comet assay evaluation criteria are unclear or missing. The authors do not state on scorable vs. non-scorable cells and hedgehog occurrence is not stated. Exposure concentrations are stated in µM and µg/mL; however, the cell line is composed of adherent cells, and thus, it would be appropriate to specify exposure concentrations in µg/cm². Moreover, laboratory’s proficiency in preparing and analysing comets’ characteristics in comet assays is a prerequisite for reliable data. It was shown that there is huge variability in tail DNA intensities even within negative or positive controls (Tavares, A.M. et al. (2014); Nanogenotox Programme). Cellular uptake was not examined. Based on the above-mentioned shortcomings the reference is considered not reliable and therefore disregarded for the hazard assessment.
1.6 Summary entry - In vitro Clastogenicity
No conclusion can be drawn from the above publications due to lack of quality, reliability and adequacy of the experimental data for the fulfilment of data requirements under REACH.
The references contained in this summary entry represent in vitro clastogenicity experiments (Micronucleus test) in mammalian cells with very limited value for risk assessment purposes. All references do not fulfil the criteria for quality, reliability and adequacy of experimental data for the fulfilment of data requirements under REACH and hazard assessment purposes (ECHA guidance R4 in conjunction with regulation (EC) 1907/2006, Annexes VII-X). The information contained therein were included for information purposes only.
Osman, I.F. et al. (2010):
The test material is insufficiently characterised. The number of cells analysed was too low (1000), as compared to OECD TG 487 (≥2000). The cell line used is not recommended by the current OECD TG 487 (2016) and essential details on the cell line are missing. Cytotoxicity was not evaluated using the recommended parameters. Information on cellular uptake or intracellular zinc ion concentration were provided. Concentration stated in µg/mL instead of µg/cm².
Valdiglesias, V. et al. (2013):
The test material was insufficiently characterised. The cell line used is not recommended by the current OECD TG 487 (2016). Moreover, essential information on the cell line is missing. The methodology of the micronucleus test is only poorly described. The number of cells analysed is not stated. The results of the negative control are not specified. Cytotoxicity is not evaluated using parameters recommend by the current OECD TG 487. The results obtained on DNA damage are implausible and show no concentration related response. Moreover, the majority of tests investigating cytotoxicity, indicated significant decreases in cell viability under all conditions tested.
Soni, D. et al. (2017):
The test material was poorly characterised. Information on the primary particle size and the particle size distribution in the medium are missing. The number of binucleated cells scored for micronucleus occurrence (1000) was lower than recommended by the current OECD TG 487 (≥2000). Information provided on the donor(s) is insufficient. No positive control was included. The description of the methodology lacks essential details (exposure period not clearly stated). Cellular uptake of the test material and intracellular zinc ion concentration were not examined. Cytotoxicity was not evaluated using the recommended parameters. The concentrations tested showed cell viabilities which were below 70%. Cytotoxicity was only evaluated in a separate experiment using the colorimetric MTT assay. Further, a potential interference with the MTT assay was neither examined nor discussed. PHA treatment and exposure deviates from the current test guideline.
Zijno, A. et al (2015):
The test material was insufficiently characterised. The negative control showed unusual high micronucleus frequencies (>7.5%). Moreover, ZnO NP treatments showing positive responses in the micronucleus test were associated with significant reductions in the replicative index, indicating marked cytotoxicity (>50%). Further, ZnO NP exposure for 24 hours resulted in significant cytotoxicity in three of the four tested concentrations. The description of the methodology lacks details. Cellular uptake and intracellular Zn ion concentration were not examined.
Bhattacharya, D. et al. (2013):
The self-synthesised test material was not fully characterised. Only one concentration was tested. The negative control showed unusual high micronucleus frequencies (>4.5%). Cytotoxicity was not evaluated using the parameters recommended by OECD TG 487 (2016) and was evaluated only in a separate experiment. The positive response observed for the rod-shaped ZnO NPs was only observed at a concentration which induced significant cytotoxicity (LD50 = 45 µg/mL). Details on the methodology and cell exposure are partly missing. It is unclear which sample of which donor(s) was used for the micronucleus test. No positive control was included. Intracellular zinc ion concentration was not examined.
Yin, H. et al. (2015):
The characterisation of the self-synthesis material lacks details. Only one concentration was tested. The number of binucleated cells scored for micronucleus occurrence (1000) was lower than recommended by the current OECD TG 487 (≥2000). Cytotoxicity was not evaluated using the parameters recommended by OECD TG 487 (2016) and was based only on 400 cells. The cell line used is not recommended by the current test guideline, and essential details on the cell line are missing. The description of the methodology and exposure misses some information. A positive control was not included. Information on the negative control is missing. Information on replicates and confirmatory experiments is not provided.
Jain, A.K. et al. (2018):
The assay was not performed according to validated test guideline. The number of cells analysed is not specified. Details on the cell line are missing. Cytotoxicity was not evaluated according to parameters recommended by OECD TG 487 (2016) and was only performed in a separate experiment utilising the MTT assay. Cytotoxicity was not markedly but significantly increased (ca. -22%) at the highest concentration tested. A positive control is not included. The frequency of micronuclei observed in control cells is not specified. Results obtained for the ZnO NP treatments are specified only relative to the negative control. Concentrations are specified in µg/mL instead of µg/cm², as appropriate for adherent cells. Historical control data is missing. ZnO NP dissolution was not investigated.
Pati, R. et al. (2016):
The self-synthesised test material was insufficiently characterised. Description of the test material preparation is incomprehensive. The assay was performed in cells not recommended by the OECD TG 487 (2016). The description of the cell line lacks details. The number of cells scored for micronucleus occurrence (1000 cells per concentration) were lower than recommended by the current OECD TG 487 (≥2000 cells). The number of independent experiments and replicates is unknown. The quantitative results are not shown. A positive control was not included. Cytotoxicity was neither concurrently examined nor using the recommended parameters. Historical control data is missing. Positive findings were observed in presence of increased cytotoxicity (50-80% cell death).
Pati, R. et al. (2016):
The self-synthesised test material was insufficiently characterised. Description of the test material preparation is incomprehensive. The description of the methodology is poor. The description of the cell line lacks details. The number of cells scored for chromosomal damage was not specified. The number of independent experiments and replicates is unknown. Quantitative results are not shown. A positive control was not included. Cytotoxicity was examined only in separate experiments. Positive findings were observed in presence of increased cytotoxicity (80% cell death).
No conclusion can be drawn from the above publications due to lack of quality, reliability and adequacy of the experimental data for the fulfilment of data requirements under REACH.
1.7 Summary entry - In vitro DNA damage
No conclusion can be drawn from the above publications due to lack of quality, reliability and adequacy of the experimental data for the fulfilment of data requirements under REACH.
The references contained in this summary entry represent in vitro DNA damage experiments in mammalian cells (Comet assay) with very limited value for risk assessment purposes. All references do not fulfil the criteria for quality, reliability and adequacy of experimental data for the fulfilment of data requirements under REACH and hazard assessment purposes (ECHA guidance R4 in conjunction with regulation (EC) 1907/2006, Annexes VII-X). The information contained therein were included for information purposes only.
Mu, Q. et al. (2014): The positive findings were found in presence of cytotoxicity and were not confirmed in a secondary experiment. Increase observed for the polymer-coated ZnO NP not dose-related. Experimental conditions were poorly described. No information on number of cells tested. No information on number of replicates Only 2-3 doses were tested. No positive control. Data is only represented by diagrams.
Anchordoquy, J.M. et al. (2011):
Methodology was insufficiently described. Test item was not characterised. No information on particle size distribution in the medium. No dose justification. No replicates or confirmatory experiments. Data is only represented by diagrams.
Guan, R. et al. (2012):
The test item was only poorly characterised. Information on test item preparation was not specified. Particle size distribution in the medium was not determined. Methodology lacks details. The number of cells analysed was too low for several experiments, since only 50 cells per experiment were scored for DNA damage (analysis of >150 cells is recommended by OECD TG 489 for the analysis of the in vivo comet). Cytotoxicity was only determined after 24 h of exposure; data on 4- and 12-h exposure are missing. The type of the negative control used was not specified. Potential interference of ZnO NPs with the MTT assay was not discussed. Contradictory information on the DNA damage parameter analysed (tail moment vs. DNA in tail). No information on precipitates or effects on pH and osmolality in culture medium. Information on particle remnants after the exposure period and during the comet assay was not provided. Concentration stated in µg/mL instead of µg/cm².
Kermanizadeh, A. et al. (2014):
Information on the methodology of the comet is missing (e.g. no info on light conditions). Information on the response of the positive control are partly missing. The results indicate a high variability in the response between treatments. The author cannot include an uneven exposure of the test system. Thus, the results could be dependent on the cells analysed (‘outer’ vs ‘inner’). The proportion of DNA in tail of FPG treated cells is in some cases apparently lower as compared to cells not treated with FPG, which is implausible. Information on potential pH or osmolality effects on the test item-treated cell medium are not discussed. Potential interference of remnant ZnO NPs with the comet assay is not discussed. Cellular uptake of the test item was not analysed.
Khan, M. et al. (2015):
The exposure time is not specified. The self-synthesised test item is insufficiently characterised (purity only specified as pure: the aspect ratio of the rod-shaped particles was not analysed; particles were not analysed after sonication). The number of cells analysed was too low, since only 50 cells were scored for DNA damage per experiment (analysis of >150 cells is recommended by OECD TG 489 for the analysis of the in vivo comet). The particle size distribution in the cell medium was not analysed. Distribution and cellular uptake of the NPs was not analysed. Information on the light conditions during treatment and comet assay is not given. Cytotoxicity was measured only in a separate experiment using different cells (erythrocytes vs. lymphocytes). However, based on the information provided, significant DNA damage was only observed in the presence of cytotoxicity. No information on negative control is provided. A positive control was missing. Potential interference of remnant ZnO NPs with the comet assay is not discussed.
Schneider, T. et al. (2017):
The test item was insufficiently characterised. Preparation of the test material lacks details. The number of cells treated was not stated. Concentration was stated in µg/mL, which is pointless for adherent cell cultures. The results on DNA damage and apoptosis are somehow implausible, since the ZnO NP treatment resulted in a significantly increased proportion of late apoptotic cells up to 80%, whereas the DNA damage was only insignificantly higher compared to the negative controls. Potential interference of remnant ZnO NPs with the comet assay is not discussed.
Ng, C.T. et al. (2017):
Exposure conditions were only poorly described, since the exposure time and cell number treated were not specified. The methodology of the comet is only poorly described and lacks all relevant information. Only one concentration was tested. The number of comets analysed is missing. A positive control was not included. Test material concentration is stated in µg/mL, which is pointless for adherent cells. Cytotoxicity tests cannot be related to the comet assay. However, treatment at 25 µg/mL (positive in the comet) resulted in a markedly increased proportion of apoptotic cells.
Musarrat, J. et al. (2009):
Results of the cytotoxicity test were neither shown nor discussed. The test material was insufficiently characterised. Details on test material preparation are missing. No characterisation of the test material preparation. Excessively high concentrations were tested.
Yang, H. et al. (2008):
The test material was insufficiently characterised. Description of test material preparation lacks details. Test material preparation was not characterised. The cell number analysed in the comet assay was to low (50 cells per experiment vs. >150 cells, as required by the OECD TG 489). Only two concentration were tested. Both concentrations tested showed cytotoxic effects in some of the cytotoxicity utilised. Test concentration were specified in µg/mL which is pointless in case adherent cells are used. Positive control is missing. No information in cellular uptake.
Watson, C. et al. (2014):
The self-synthesised test material was insufficiently characterised, since information on purity and impurity elements are missing. The number of cells analysed in the comet assay is not specified. The description of the methodology of the comet assay lacks details. The concentration of the treatments is specified in µg/mL for the adherent H9T3 cell line. Information on cellular uptake is not provided. All concentration tested with H9T3 cells showed significant cytotoxicity as evidenced by the MTT assay.
Uzar, N.K. et al. (2015):
The characterisation of the self-synthesised material is implausible or at least fully comprehensible. Impurities are not discussed but are present according to EDS. The number of cells analysed per concentration was lower than recommended in equivalent test guidelines (100 vs 150 cells). The comet methodology was not sufficiently described, and light conditions were not specified. The significant increase in DNA damage was observed in presence of cytotoxicity, as indicated by the neural red uptake and trypan blue exclusion assays. Concentration specified in µg/mL instead of µg/cm². No information on cellular uptake.
Osman, I.F. et al. (2010):
The test material is insufficiently characterised. The number of cells analysed was too low for several experiments, since only 50 cells per experiment were scored for DNA damage (analysis of >150 cells is recommended by OECD TG 489 for the analysis of the in vivo comet). A non-standard cell line was used for the comet assay and essential details on the cell line are missing. The comet methodology was insufficiently described. No information on light conditions during comet assay were provided. Information on cellular uptake or intracellular zinc ion concentration were provided. Concentration stated in µg/mL instead of µg/cm².
Valdiglesias, V. et al. (2013):
The test material was insufficiently characterised. A cell line, which is not standard for genotoxicity testing, was used in the assay. The methodology of the comet assay was only poorly described. The number of cells analysed was too low for all experiments, since only 100 cells per experiment were scored for DNA damage (analysis of >150 cells is recommended by OECD TG 489 for the analysis of the in vivo comet). Information on light conditions during the comet assay are not provided. The results of the negative control are not specified. The results obtained on DNA damage are implausible and show no concentration related response. Moreover, the majority of tests investigating cytotoxicity, indicated significant decreases in cell viability under all conditions tested.
Soni, D. et al. (2017):
The test material was poorly characterised. Information on the primary particle size and the particle size distribution in the medium are missing. The comet assay was performed in a cell line which is non-standard for genotoxicity testing. The description of the comet assay methodology lacks details. The number of cells analysed was too low for all experiments, since only 100 cells per experiment were scored for DNA damage (analysis of >150 cells is recommended by OECD TG 489 for the analysis of the in vivo comet). The cell line is insufficiently characterised. No positive control was included. Cellular uptake of the test material and intracellular zinc ion concentration were not examined. The concentrations tested showed cell viabilities which were below 70%. Cytotoxicity was only evaluated in a separate experiment using the colorimetric MTT assay. Further, a potential interference with the MTT assay was neither examined nor discussed.
Zijno, A. et al (2015):
The test material was insufficiently characterised. The number of cells scored for DNA damage is not specified. No information on replicate slides. Only two concentrations were tested. The description of the methodology lacks details. No information on light exposure in post-lysis steps. Cytotoxicity was not determined. The DNA damage was only in depicted in diagrams and as measure of total DNA damage, net oxidative DNA damage after DNA glycosylase treatment, however, was neither depicted nor discussed, which hampers adequate evaluation of the amount of oxidative DNA damage after NP treatment. Cellular uptake and intracellular Zn ion concentration were not examined.
Bhattacharya, D. et al. (2013):
The self-synthesised test material was not fully characterised. Only one concentration was tested. The results are contradictory, since the spherical ZnO NP resulted in both a positive and negative response under the same conditions tested. Moreover, the positive response observed for the rod-shaped ZnO NPs was only observed at a concentration which induced significant cytotoxicity (LD50 = 45 µg/mL). Details on the comet methodology and cell exposure are missing. Light condition during the comet are not specified. It is unclear which sample of which donor(s) was used for the comet assay. Cytotoxicity was evaluated only in a separate experiment. No positive control was included. Intracellular zinc ion concentration was not examined.
Pal, A. et al. (2016):
The test material was insufficiently characterised. The comet methodology was not described. No information on light conditions during comet assay. Only one concentration was tested. Only 50 cells per experiment were scored for DNA damage, whereas the equivalent in vivo test guideline (OECD TG 489, 2016) requires to score at least 150 cells. The positive response was observed in presence of significant cytotoxicity as the proportion of apoptotic cells and cell cycle arrested cells were significantly increased. The results of the negative control are not specified, and results are only specified in fold changes over control. A positive control was not included. Concentration specified in µg/mL, which is pointless for adherent cells.
Ghosh, M. et al. (2016):
The test material is insufficiently characterised. The comet assay methodology is not described. The light conditions during the comet assay are not described. The test showed the test material to be negative, but the test material was not tested up to cytotoxic concentrations. A positive control was not included. The type of the negative control is not specified.
Pati, R. et al. (2016):
The self-synthesised test material was insufficiently described. The description of the test material preparation is incomprehensive. The description of the comet assay methodology lacks details. Information on potential exposure during electrophoresis is not provided. The number of cells scored for DNA damage was not specified. The DNA damage was specified only in tail length, which, however, is not the recommended parameter to assess DNA damage in the comet assay. Only two concentration were evaluated for DNA damage. The positive finding in DNA damage was observed only at a markedly cytotoxic (80% cell death) concentration. A positive control was not included. Number of replicates and independent experiments unknown.
Valdiglesias, V. et al. (2013):
The test material was insufficiently characterised. A cell line, which is not standard for genotoxicity testing, was used in the assay. The results of the negative control are not specified. The positive results obtained on H2AX phosphorylation show no concentration related response and were only observed in the mid dose group. Moreover, the majority of tests investigating cytotoxicity, indicated significant decreases in cell viability under all conditions tested.
Heim, J. et al. (2015):
The test material was insufficiently characterised. A cell line, which is not standard for genotoxicity testing, was used in the assay. Description of the cell line lacks details. Only 60 cells were analysed per experiment. The variability of results was high. No positive was included. Only two concentrations were analysable for the 24-h treatment- Particle size distribution in the medium was not examined. Cytotoxicity was evaluated only in a separate experiment and not for all time points tested in the γH2AX assay.
Nam, S.-H., et al. (2012):
The assay was not performed according to any validated test guideline. The test materials are insufficiently characterised. Bacterial assays are considered inadequate for genotoxicity testing of NPs, since NP uptake is not convincingly demonstrated for bacteria*. The positive control used is not adequate to demonstrate adequateness of the test system (non-particulate test material). Zn concentration in cell is not determined. Cellular uptake of test material was not examined. Type of negative control is not further specified. Viability of cells before treatment no checked and information on cell viability after treatment are missing. Cytotoxicity only specified in alkaline phosphates enzyme units.
Wang, M.M. et al. (2015):
The assay was not performed according to any validated guideline. The CD59 assay methodology and analysis is only poorly described. The number of cells treated and exposure conditions are not sufficiently described. The cell line is not sufficiently described. The response is not correlated with the cytotoxicity. A positive control is not included. Type of negative control is not specified. Evaluation are not stated and unclear. Historical control is missing. The increases in mutation frequency were only slight (≤2.1x-fold) and the strongest increased were found in presence of cytotoxicity.
*References
Elespuru, R. et al. (2018). Genotoxicity Assessment of Nanomaterials: Recommendations on Best Practices, Assays, and Methods. TOXICOLOGICAL SCIENCES, 164(2), 2018, 391–416
No conclusion can be drawn from the above publications due to lack of quality, reliability and adequacy of the experimental data for the fulfilment of data requirements under REACH.
The references contained in this summary entry represent in vitro DNA damage experiments in mammalian cells (Comet assay) with very limited value for risk assessment purposes. All references do not fulfil the criteria for quality, reliability and adequacy of experimental data for the fulfilment of data requirements under REACH and hazard assessment purposes (ECHA guidance R4 in conjunction with regulation (EC) 1907/2006, Annexes VII-X). The information contained therein were included for information purposes only.
Picco, S.J. et al. (2009):
No information on comet assay methodology. Controls were not included. Information on the test material is restricted to its name.
Sharif, R. et al. (2012):
The test materials are, except for the substance name, not characterised. The comet assay methodology is only very poorly described. The cells used are not standard for genotoxicity testing. The description of the cell line lacks details. A negative control was not included in the multiple comparison analysis. No positive control was included. Cytotoxicity was examined only in a separate experiment utilising the MTT assay and the response was not related to negative control values.
Padula, G. et al. (2014):
The test material characterisation is restricted to the substance name. Description of the comet assay methodology lacks details. The Zn ion levels of the different groups were not specified, which reduces the comparability of the different groups. Negative control cells were treated with unchelated culture medium in combination with ZnSO4. Not specified whether the lymphocyte samples were pooled, or which samples was used for which treatment. Cytotoxicity was only evaluated in a separate experiment using the trypan blue exclusion test. The two highest concentration tested in the comet assay showed markedly and significantly decreased cell viability (ca. -79 and -91%). Positive control was not included.
Sharif, R. et al. (2011):
The test materials are only poorly characterised. The cells used are not standard for genotoxicity testing. The description of the cell line lacks details. The comet assay methodology is only poorly described. A negative control was not included in the multiple comparison analysis. No positive control was included. Cytotoxicity was examined only in a separate experiment utilising the MTT assay and the response was not related to negative control values.
2 In vivo
2.1 in vivo clastogenicity
2.1.1 Micronucleus assays
A 14-day repeated dose inhalation toxicity study (Creutzenberg, 2013) was conducted to establish exposure dose-response relationships of the Z-COTE HP1 in rats after subacute nose-only exposure according to the OECD Guideline 412 in compliance with GLP. In the framework of this study also systemic clastogenic and aneugenic effects were investigated in male and female rats using the bone marrow micronucleus assay according to OECD Guideline 474. Five rats per sex and group were exposed at concentration levels of 0, 0.5, 2, or 8 mg/m³ with Z-COTE HP1. Fresh air treated animals served as concurrent control. Positive control animals were orally exposed to 20 mg/kg cyclophosphamide monohydrate. There was no treatment-related reduction of body weights in any test group; no clinical signs were detected. However, high dose rats showed adverse local effects in the lungs. In male rats, Z-COTE HP1 and the reference substances did not mediate significant repression of red blood cell formation. In females, the PCE/NCE was significantly reduced in the positive control as well as in females exposed to 8 mg/m³ Z-COTE HP1. This effect might indicate that these test items reached the bone marrow as target organ. However, toxicokinetic data obtained in parallel showed the test material to be not available in the blood stream. There was no evidence of a significantly enhanced mean frequency of micronucleated erythrocytes due to Z‑COTE HP1 exposure in males or females, as compared to the vehicle control groups (clean air) at any dose level. The positive and vehicle controls gave valid results. Inhaled Z-COTE HP1 is considered non-mutagenic in immature bone marrow erythrocytes (PCE) of Wistar rats under the conditions tested.
A 14-day repeated dose inhalation toxicity study (Creutzenberg, 2013) was conducted with uncoated Z-COTE in rats exposed subacutely via nose-only exposure according to the OECD Guideline 412 in compliance with GLP. In the framework of this study also systemic clastogenic and aneugenic effects were investigated in male and female rats using the bone marrow micronucleus assay according to OECD Guideline 474. Five rats per sex and group were exposed at a concentration level of 8 mg/m³ with Z-COTE HP1. Fresh air treated animals served as concurrent control. Positive control animals were orally exposed to 20 mg/kg cyclophosphamide monohydrate. There was no treatment-related reduction of body weights in any test group; no clinical signs were detected. However, high dose rats showed adverse local effects in the lungs. In male rats, the test item and the reference substances did not mediate significant repression of red blood cell formation. In females, the PCE/NCE was significantly reduced in the positive control as well as in females exposed to 8 mg/m³ uncoated Z-COTE. This effect might indicate that these test items reached the bone marrow as target organ. However, toxicokinetic data obtained in parallel showed the test material to be not available in the blood stream. There was no evidence of a significantly enhanced mean frequency of micronucleated erythrocytes due to Z‑COTE HP1 exposure in males or females, as compared to the vehicle control groups (clean air) at any dose level. The positive and vehicle controls gave valid results. Inhaled Z-COTE is considered non-mutagenic in immature bone marrow erythrocytes (PCE) of Wistar rats under the conditions tested.
In a BASF study (2009; published by Landsiedel, R. et al., 2010), Z-COTE HP1 was assessed in NMRI mice for a potential clastogenic or aneugenic activity. Groups of five male mice received a single intraperitoneal injection of Z-COTE HP1 at dose levels of 15, 30, and 60 mg/kg bw. Concurrent vehicle control animals received a single injection of pure FCS. Positive control animals received either cyclophosphamide or vincristine. Test animals from each group were euthanised after 24 hours. In addition, test animals from the Z-COTE HP1 high dose and vehicle control animals were sacrificed after 48 hours. Subsequently, femoral bone marrow cells were obtained from each animal and stained with modified May-Grünwald/Giemsa solution. A total of 2000 polychromatic erythrocytes per animal were scored for the occurrence of micronuclei. In addition, the proportion of large and small micronuclei was determined for each group. Moreover, the micronucleus frequency in normochromatic erythrocytes and the proportion of polychromatic among total erythrocytes were determined. Male NMIR mice, exposed to Z-COTE HP1 for 24 and 48 hours via a single intraperitoneal injection, did not show a statistically significant alteration in the micronucleus frequency, when compared to vehicle control animals. Moreover, the micronucleus frequencies observed were well within the historical negative control data range. At the same time, the concurrent vehicle control groups showed micronucleus frequencies within the historical negative control data range and positive control groups showed a clear and statistically significant increase in the micronucleus formation rate. Thus, the validity and sensitivity of the test system was demonstrated. According to the authors, the proportion of polychromatic erythrocytes was slightly depressed, indicating that the test material reached the target organ. This effect was, however, not statistically significant and possibly due to a low concurrent negative control group value. The study presented herein shows major shortcomings in the design of the study. The test material was administered via intraperitoneal injection, which is considered to be a non-physiological route of exposure with only limited value for hazard assessment purposes. Moreover, exposure of the target organ was not demonstrated. Based on these limitations, the study is considered to be not reliable.
Gosh, M. et al. (2016) investigated on the chromosome damaging capacity of ZnO NP in mice bone marrow erythrocytes after intraperitoneal injections. In order to evaluate the chromosomal damage, an erythrocyte micronucleus test was performed. Furthermore, the generation of ROS, mitochondrial membrane potential, and cell cycle phases in bone marrow cells were analysed using FACS in combination with DCFDA, Rhodamine 123, and propidium iodide, respectively. In order to investigate on oxidative stress, levels of malondialdehyde, glutathione, glutathione S-transferase activity, and catalase activity were determined. Moreover, serum albumin levels were spectrophotometrically determined. According to the authors, intraperitoneal treatment with ZnO-NP resulted in significant increase in the micronucleus frequency in polychromatic erythrocytes at all doses tested, when compared to the vehicle control group. Moreover, the highest dose induced a fivefold micronucleus formation frequency above the control. Significant cytotoxicity, as indicated by the proportion of polychromatic erythrocytes among total erythrocytes, was not evident at the doses tested. Further, the authors observed significantly increased ROS levels and a significant reduction in the mitochondrial membrane potential. The distribution of cell cycle phase was altered showing decrease in G2/M and S-phase cells in the two highest dose groups. Moreover, the catalase and glutathione S-transferase activity were significantly decreased in the lowest-dose group, whereas the reduced glutathione level showed a significant decrease in the high-dose group. No conclusion can be drawn due to reporting and methodological deficiencies. The test material is insufficiently described, since information on purity, impurity elements, and surface modifications are missing. The test material was administered via intraperitoneal injection, which, however, is considered as an unphysiological exposure route. The description of the animal treatment lacks details. Information on animal weight and age do not fit according to usual growth charts (8-12 weeks vs 25-30 g bw). Information on caging and test group randomisation are missing. Information on clinical signs or body weight loss in ZnO NP-treated animals is not provided. Moreover, the description of the micronucleus test methodology lacks details. The number of cells scored for chromosomal damage (2000) was lower than recommended by the OECD TG 474 (≥4000). Historical control data is not provided. Based on the above-mentioned shortcomings the reference is considered not reliable and therefore disregarded for the hazard assessment.
Kwon, J.Y. et al. (2014) investigated on the chromosome damaging capacity of two different ZnO NP types, each prepared in two different suspension, in bone marrow erythrocytes of gavaged ICR mice. The mice received test material doses of 500, 1000, and 2000 mg/kg bw via two gavages administered at a 24-h interval. Afterwards, an erythrocyte micronucleus test was performed according to OECD TG 474 (under GLP conditions) in order to evaluate potential chromosomal damage induced by the treatment with the four different ZnO NP suspensions. According to the authors, none of the four ZnO NP suspensions tested induced an increase in the frequency of micronucleated PCEs when compared to the vehicle control groups. Bone marrow toxicity, as indicated by an altered PCE/NCE was evident in mice treated with ZnO NP 20 nm (+) and (-) charge at all doses tested. Moreover, mice exposed to the highest dose of ZnO NP 20 nm (+) charge showed significantly reduced body weights. No conclusion can be drawn due to reporting and methodological deficiencies. The test material is insufficiently described, since information on purity, impurity elements, and surface modifications are missing. The description of the test animals lacks details, since information on body weights at study initiation, sex, and housing conditions are missing. The preparation of the dosing solutions is insufficiently described. The post-exposure period until the sacrifice is not specified. The methodology of the micronucleus test is only poorly described. The number of animals treated, and cells used for micronucleus is not specified. The identity of the positive control substance is not specified. Evaluation and scoring criteria are not specified. Information on statistical tests is not provided. Historical control data is missing. Bone marrow exposure was not demonstrated for the ZnO NP 70 nm suspension. However, the test was performed up the limit dose (2000 mg/kg bw). Based on the above-mentioned shortcomings the reference is considered not reliable and therefore disregarded for the hazard assessment.
Li, C.-H. et al. (2011) examined on the capacity of ZnO NP and ZnO MP to induce chromosomal damage in peripheral blood erythrocytes of orally treated mice. The mice received a single gavage of the test materials and blood samples were taken after 24, 48, and 72 h. The chromosomal damage in erythrocytes was examined via a micronucleus test. Moreover, the authors investigated on the adsorption and biodistribution of the test material after a single oral gavage. According to the authors, mice orally exposed to ZnO NPs and ZnO MPs showed no significant differences in the frequency of micronucleated PCEs, when compared to the solvent control group. Moreover, the ration of PCEs among total erythrocytes was not statistically significantly different between groups. In contrast, the positive control group showed both significantly increased micronucleated PCE frequencies and depressed PCE to total erythrocytes ratios. The analysis of the adsorption and biodistribution after oral treatment revealed rapid absorption in the serum and biodistribution to liver, spleen, and kidneys. In contrast, the lung, heart, brain, and testes showed no increased zinc levels after ZnO NP and ZnO MP treatment. No conclusion can be drawn due to reporting and methodological deficiencies. The test material is insufficiently characterised, since information on purity, and impurity elements is missing. Further, the description of the test material preparation lacks details. The description of the test animals lacks details, including body weights at study initiation, test group randomisation, and housing. Moreover, the description of the micronucleus test methodology lacks details. The number of cells scored for chromosomal damage (1000) was lower than recommended by the OECD TG 474 (≥4000). Furthermore, the number of cells scored to determine the ratio of PCEs among total erythrocytes was lower than requested by OECD TG 474. Information on clinical signs is missing. Evaluation and scoring criteria are not specified. Data from individuals is not specified. Exposure of the target organ was not discussed. However, the analysis of the serum showed rapidly increased zinc levels after a single oral treatment with ZnO NPs and ZnO MPs at 2.5 g/kg bw. Historical control data is missing. Based on the above-mentioned shortcomings the reference is considered not reliable and therefore disregarded for the hazard assessment.
2.2 in vivo DNA damage (Comet assay)
Hornarvar (2022) investigated on the genotoxic potential of uncoated nano Zinc oxide (T0420) and coated nano Zinc oxide (T0421) in male Wistar rats after a whole-body inhalation exposure. Five rats per group were exposed to dust aerosol of T0420 and T0421 at target concentration levels of 0.5, 2, and 8 mg/m3 for 6 hours/day on 14 consecutive days. Vehicle control (clean air) rats were run concurrently. Approximately 0.5-1 hour after the last exposure, the animals were sacrificed, and tissues were prepared for the Comet assay and BALF analysis. Bone marrow, liver, lung and nasal mucosa are tested for DNA damage using the Comet assay. A total of 150 cells per tissue sample were scored for the tail intensity (%DNA in tail). As positive controls, sample tissues of animals administered orally at a single time point Ethyl methanesulfonate (EMS), were scored.
There were no signs of toxicity, nor were there any impairment of body weight gain. Excessive cytotoxicity was not detected. In animals exposed to test item T0420, concentration-related and statistically significant increases of the lavage parameters total protein, gamma-Glutamyl-transferase; lactate dehydrogenase; alkaline phosphatase and beta-N-acetyl glucosaminidase were observed, as well as in animals exposed to test item T0421 (except for beta-N-Acetyl glucosaminidase). The changes in lavage fluid demonstrate the toxicity in the lungs, which were comparable with the 14-days dose range finding study (Ma-Hock, 2022). The assessment of the target tissues in the comet assay did not show any biologically relevant increases in the % tail intensity of the analyzed tissues. The positive control group showed a distinct and statistically significant increase in all analyzed tissues. Thus, under the conditions tested, the two test substances (uncoated nano Zinc oxide (T0420) and coated nano Zinc oxide (T0421) are considered as non-genotoxic in this assay.
Creutzenberg (2013) investigated on the potential site-of-contact genotoxicity of Z-COTE HP1 in male Wistar rats after repeated nose-only inhalation. Five rats per group were exposed to Z-COTE HP1 aerosol at concentration levels of 0.5, 2, and 8 mg/m³ for 5 days/week and 6 hours/day over a total period of two weeks. Vehicle control (clean air) rats were run concurrently. At post exposure days 1 and 14, BAL cells were obtained and tested for DNA damage using the modified alkaline comet assay. In this type of comet assay, lysed cells were either treated with buffer or with hOGG1 enzyme to detect general and oxidative DNA damage. A total of 100 cells per animal were scored for the tail intensity (% DNA in tail). As technical positive control served potassium bromate-treated L5178Y/TK+/- mouse lymphoma cells. Moreover, in an independent experiment, rats exposed to potassium bromate (250 mg/kg bw) via intraperitoneal injection served as in vivo positive controls. Apart from the comet assay, the authors examined the formation of 8-OH-dG in formalin-fixed tissue of the terminal bronchioles and lung parenchymal cells via immunohistochemistry. Wistar rats exposed to Z-COTE HP1 did not show signs of general toxicity. However, the local adverse effects in the lung were evident in the highest dose group. Notably, the LDH activity in BAL fluid was statistically significantly increased in the high dose group indicating cytotoxic effects induced at a concentration level of 8 mg/m³. The BAL cells of rats exposed to Z-COTE HP1 did not show a statistically significant increase in the tail intensity after a 24-hour recovery period, when compared to concurrent vehicle control rats. After 14 days, the intermediate dose group showed a statistically significant increase in the tail intensity only in absence of a hOGG1 treatment. In contrast, BAL cells obtained from the high dose group animals showed a statistically significantly increased tail intensity only after hOGG1 treatment. Notably, the increases observed were only marginally higher than the responses observed for the concurrent vehicle control. Consequently, the observed responses are considered to be not biologically relevant. In contrast, the independent in vivo positive controls showed marked and statistically significantly both with and without hOGG1 treatment, when compared negative controls. Thus, the general sensitivity of the test system was demonstrated. Moreover, the technical positive control demonstrated the activity of the used hOGG1 enzyme and the appropriate performance of the comet assay methodology. In addition, terminal bronchioles and lung parenchymal cells from Z-COTE HP1 exposed rats did not show statistically significantly increased numbers of 8-OH-dG positive nuclei, when compared to the vehicle control values. Thus, the lack of Z-COTE HP1-induced oxidative DNA damage observed in the comet assay was further substantiated by the data obtained in the immunohistochemical assay using lung sections. The study presented herein is considered to be acceptable with restrictions. The experiment included in the study were not performed under GLP conditions, which was basically due to the fact that an OECD validated test guideline was not available at that time. The tail intensity was determined only using a single slide per animal; replicates were not included. Furthermore, the responses observed showed in several cases a huge variability. Thus, the robustness of the data may be limited. Moreover, the description of the methodology lacks detailed information on cell lysis, neutralisation, and electrophoresis. The positive controls were run only in an independent experiment, concurrent positive control animals were not included. Cytotoxicity was not sufficiently investigated and evaluated. The only parameter useful for an evaluation of cytotoxicity is the LDH activity level in BAL fluid. Evaluation criteria are not specified. Historical control data are not included. The test material, Z-COTE HP1, is considered to have no DNA damaging potential based on the absence of biological relevant increases in the median derived mean tail intensity under the conditions tested.
Creutzenberg (2013) investigated on the potential site-of-contact genotoxicity of Z-COTE in male Wistar rats after repeated nose-only inhalation. Five rats were exposed to Z-COTE aerosol at a concentration level of 8 mg/m³ for 5 days/week and 6 hours/day over a total period of two weeks. Vehicle control (clean air) rats were run concurrently. At post exposure days 1 and 14, BAL cells were obtained and tested for DNA damage using the modified alkaline comet assay. In this type of comet assay, lysed cells were either treated with buffer or with hOGG1 enzyme to detect general and oxidative DNA damage. A total of 100 cells per animal were scored for the tail intensity (% DNA in tail). As technical positive control served potassium bromate-treated L5178Y/TK+/- mouse lymphoma cells. Moreover, in an independent experiment, rats exposed to potassium bromate (250 mg/kg bw) via intraperitoneal injection served as in vivo positive controls. Apart from the comet assay, the authors examined the formation of 8-OH-dG in formalin-fixed tissue of the terminal bronchioles and lung parenchymal cells via immunohistochemistry. Wistar rats exposed to Z-COTE did not show signs of general toxicity. However, the local adverse effects in the lung were evident in the highest dose group. Notably, the LDH activity in BAL fluid was statistically significantly increased in animals exposed to 8 mg/m³ indicating cytotoxic effects. The BAL cells of rats exposed to Z-COTE HP1 did not show a statistically significant increase in the tail intensity after 1 and 14 days of the final treatment, when compared to the concurrent vehicle control. Moreover, the hOGG1 treatment did not reveal an increase of oxidative DNA damage. In contrast, the independent in vivo positive controls showed marked and statistically significantly both with and without hOGG1 treatment, when compared negative controls. Thus, the general sensitivity of the test system was demonstrated. Moreover, the technical positive control demonstrated the activity of the used hOGG1 enzyme and the appropriate performance of the comet assay methodology. In addition, terminal bronchioles and lung parenchymal cells from Z-COTE exposed rats did not show statistically significantly increased numbers of 8-OH-dG positive nuclei, when compared to the vehicle control values. Thus, the lack of Z-COTE-induced oxidative DNA damage observed in the comet assay was further substantiated by the data obtained in the immunohistochemical assay using lung sections. The study presented herein is considered to be acceptable with restrictions. The experiment included in the study were not performed under GLP conditions, which was basically due to the fact that an OECD validated test guideline was not available at that time. The tail intensity was determined only using a single slide per animal; replicates were not included. Furthermore, the responses observed showed in several cases a huge variability. Thus, the robustness of the data may be limited. Moreover, the description of the methodology lacks detailed information on cell lysis, neutralisation, and electrophoresis. The positive controls were run only in an independent experiment, concurrent positive control animals were not included. Cytotoxicity was not sufficiently investigated and evaluated. The only parameter useful for an evaluation of cytotoxicity is the LDH activity level in BAL fluid. Evaluation criteria are not specified. Historical control data are not included. Only one dose level was tested, which precludes an evaluation of dose-response relationships. The test material, Z-COTE, is considered to have no DNA damaging potential based on the absence of biological relevant increases in the median derived mean tail intensity under the conditions tested.
Kwon, J.Y. et al. (2014) examined two different particle types, each prepared in two different suspensions, for their potential to induced DNA damage in the liver and stomach of rats treated per gavage. The four different test material suspensions were tested at three different doses, up to the limit dose (2000 mg/kg bw), as selected in a preceding dose ranger finder. The DNA damage was scored for a total of 100 cell per test animal. According to the authors, tail intensity of liver and stomach single cells of rat treated orally with four different ZnO NP suspensions was not significantly different from vehicle control values. In contrast, the positive control group showed a strong response. No conclusion can be drawn due to reporting and methodological deficiencies. The test material is insufficiently characterised, i.e. information on purity, impurity elements and surface modifications is missing. Details on the test animals are partly missing, including source, body weights at study initiation, and housing conditions. Description of the preparation of the dosing solution and test animal treatment lacks details. The description of the comet methodology lacks details. Information on buffers and temperature during electrophoresis are missing. Moreover, it is unclear how many animals were examined for DNA damage. Importantly, the authors do not state removal and disposition of particles after exposure, remaining ZnO NPs could have interfered with electrophoresis and comet scoring. The identity of the positive control substance is not specified. Historical control data is missing. The authors do not state on scorable vs. non-scorable cells and hedgehog occurrence is not stated. Moreover, laboratory’s proficiency in preparing and analysing comets’ characteristics in comet assays is a prerequisite for reliable data. It was shown that there is huge variability in tail DNA intensities even within negative or positive controls (Tavares, A.M. et al. (2014); Nanogenotox Programme). The diagrams presented in the reference show huge intra- and interexperimental variability in the tail intensities. The exposure of the target organs is not demonstrated, the test material was, however, tested up to the limit dose (2000 mg/kg bw). Based on the above-mentioned shortcomings the reference is considered not reliable and therefore disregarded for the hazard assessment.
Larsen, S.T. et al. (2016) examined potential DNA damage, via the alkaline comet assay, in BALF and lung cells of mice exposed to two different ZnO NP types for a single 1-h inhalation. The comet assay and BALF analyses were performed after a 24-h post-exposure period. According to the authors, none of the two ZnO NP types tested induced significant DNA damage in lungs of mice, when compared to air-exposed mice. However, the protein concentration in BALF was significantly increased at the two highest concentrations tested. Moreover, the numbers of both neutrophils and lymphocytes were significantly increased after ZnO NP exposure. No conclusion can be drawn due to reporting and methodological deficiencies. The description of the comet methodology lacks details. Information on buffers and temperature during electrophoresis are missing. Further, electrophoresis times varied between cell types potentially leading to artefactual difference in response. Moreover, it is unclear how many animals were examined for DNA damage and how many comets were scored per animal. Importantly, the authors do not state removal and disposition of particles after exposure and light conditions during the comet assay. However, the photocatalytic capacity of the ZnO NPs could have led in association with ‘naked’ DNA and light to false positives. Moreover, remaining ZnO NPs could have interfered with electrophoresis and comet scoring. The authors tested both BALF and lung cells; however, it is not indicated whether these samples show different responses and how many cells of which type was used for the DNA damage evaluation. Only one dose group was scored for the ZnO_1 NP type treatment. A positive control is not included. However, TiO2 was tested and in parallel and demonstrated the sensitivity of the test system. Historical control data is missing. The authors do not state on scorable vs. non-scorable cells and hedgehog occurrence is not stated. Evaluation criteria are not specified. Moreover, laboratory’s proficiency in preparing and analysing comets’ characteristics in comet assays is a prerequisite for reliable data. It was shown that there is huge variability in tail DNA intensities even within negative or positive controls (Tavares, A.M. et al. (2014); Nanogenotox Programme). Lung histopathology was performed in lavaged lungs. Based on the above-mentioned shortcomings the reference is considered not reliable and therefore disregarded for the hazard assessment.
2.3 Summary entry - In vivo clastogenicity
No conclusion can be drawn from the above publications due to lack of quality, reliability and adequacy of the experimental data for the fulfilment of data requirements under REACH.
The references contained in this summary entry represent in vivo experiments on genetic toxicity with very limited value for risk assessment purposes. All references do not fulfil the criteria for quality, reliability and adequacy of experimental data for the fulfilment of data requirements under REACH and hazard assessment purposes (ECHA guidance R4 in conjunction with regulation (EC) 1907/2006, Annexes VIIX). The information contained therein were included for information purposes only.
Bollu, V.S. et al. (2016):
The test material is insufficiently characterised. Detail on test animals missing, including body weight and clinical signs during treatment. Description of the micronucleus test methodology lacks details. The number of polychromatic erythrocytes scored for micronuclei occurrence (2000 per animal) is lower than recommended by the current OECD TG 474 (≥4000 per animal). Moreover, the number of cells scored for cytotoxicity determination was lower (1000 per animal) than recommended (≥2000 per animal). The test material was not tested up to toxic concentrations. The test material was not tested up to toxic concentrations. Exposure of bone marrow was not shown. A positive control group was not included. The results were not related to a negative control group. Historical control data is missing. Interestingly, the authors evaluated the test material to be negative in the micronucleus test, based on the lack of significant differences between pre-treatment and treated animals. However, the values of micronuclei in polychromatic erythrocytes are apparently increased in a dose-related manner.
Pati, R. et al. (2016):
The test material is insufficiently characterised. The description of the test material preparation lacks details. Exposure and sampling schedule are unclear. The micronucleus assay methodology is only poorly described. The body weight, sex, and number of test animals treated is unknown. The number of cells (100) analysed per mice was lower than recommended in OECD TG 474 (≥4000). Positive control was not included. Historical control data is missing. Both doses tested resulted in animal death and clinical signs as well as significant inflammation.
Bollu, V.S. et al. (2016):
The test material is insufficiently characterised. Detail on test animals missing, including body weight and clinical signs during treatment. Description of the chromosomal aberration assay methodology lacks details. The number of metaphases scored for chromosomal aberration (100 per animal) is lower than recommended by the current OECD TG 475 (≥200 per animal). The test material was not tested up to toxic concentrations. A positive control group was not included. Historical control data is missing. Interestingly, the authors evaluated the test material to be negative in the chromosomal aberration test, based on the lack of significant differences between vehicle control and treatment animals. However, the values of total chromosomal aberrations and chromosomal aberrations per cells are apparently increased in a dose-related manner.
Bollu, V.S. et al. (2016):
The test material is insufficiently characterised. Detail on test animals missing, including body weight and clinical signs during treatment. Description of the chromosomal aberration assay methodology lacks details. The animals were, 2 h before cell harvest, treated with colchicine, according to OECD TG 475, however, the treatment should be 3-5 h before cell harvest. Moreover, the sampling time was later (48 h post-exposure) than recommended (13-18 h). The number of metaphases scored for chromosomal aberration (100 per animal) is lower than recommended by the current OECD TG 475 (≥200 per animal). The test material was not tested up to toxic concentrations. Exposure of bone marrow was not shown. A positive control group was not included. Historical control data is missing. Interestingly, the authors evaluated the test material to be negative in the chromosomal aberration test, based on the lack of significant differences between vehicle control and treatment animals. However, the values of total chromosomal aberrations and chromosomal aberrations per cells are apparently increased in a dose-related manner.
2.4 Summary entry - In vivo DNA damage
No conclusion can be drawn from the above publications due to lack of quality, reliability and adequacy of the experimental data for the fulfilment of data requirements under REACH.
The references contained in this summary entry represent in vivo experiments on genetic toxicity with very limited value for risk assessment purposes. All references do not fulfil the criteria for quality, reliability and adequacy of experimental data for the fulfilment of data requirements under REACH and hazard assessment purposes (ECHA guidance R4 in conjunction with regulation (EC) 1907/2006, Annexes VIIX). The information contained therein were included for information purposes only.
Jacobsen, N.R. et al. (2015):
The test material was administered via unphysiological route of exposure. The comet methodology was not described. The number of cells scored for DNA damage is unknown. Light exposure during cell harvest and preparation as well as during the comet assay is not specified. The number of mice analysed per treatment group varied considerably (4 mice in 72-h post-exposure treatment groups vs. 12 mice in the 24-h post-exposure vehicle control group. The positive control is inadequate, and the positive control group showed no significant increase DNA damage. Dose group number and number of animals per group are too low for the 72-h postexposure period. The response was only slight, since the maximum fold increase in the proportion of DNA in tail observed was 2. The positive responses were observed in presence of inflammation (4 to 6.6-fold increase in neutrophil number) and cell damage (ca. 2-fold increase in protein concentration).
Sharma, V. et al. (2012):
The test material was not sufficiently characterised. Information on animal exposure is incomplete. The description of the comet methodology lacks details. No information on light exposure during cell preparation and comet assay. The number of cells scored (100) for DNA damage was lower than recommend in the current OECD TG 489 (≥150). Only two doses were tested, whereas a minimum of three is recommended by the current test guideline. Number of animals used for DNA damage evaluation is unclear. No positive control was included. Historical control data is missing. The increase in DNA damage is only slight (1.2-1.5x). Moreover, the increase in DNA damage is observed in presence of toxicity, including increased levels of ALT, ALP, and apoptosis as well as histopathological alterations. Results of trypan blue exclusion test are not shown. Body and organ (liver, brain, and kidney) weighs were not significantly different between groups after the 14-day exposure period.
Pal, A. et al. (2016):
The test material was insufficiently characterised. The comet methodology was not described. No information on light conditions during comet assay. Only one concentration was tested. Only 50 cells per experiment were scored for DNA damage, whereas the equivalent in vivo test guideline (OECD TG 489, 2016) requires scoring of at least 150 cells. The positive response was observed in presence of significant cytotoxicity as the proportion of apoptotic cells and cell cycle arrested cells were significantly increased. The results of the negative control are not specified, and results are only specified in fold changes over control. A positive control was not included. Concentration specified in µg/mL, which is pointless for adherent cells.
Attia, H. et al. (2018):
The test material is insufficiently characterised. Description of test material preparation lacks details. Data on the test animals are missing, including source, caging, and test group randomisation. Moreover, the information on age and weight do not match with each other according to typical growth chart data. Information on test material administration are missing. Information on body weights and clinical signs are not provided. Test groups differ in size, results from negative control animals from single and repeated dose groups were pooled. Description of comet methodology lacks details. Electrophoresis was performed at room temperature. The number of cells scored for DNA damage (100 per animal) is lower than recommended by the current OECD TG 489 (≥150 per animal). Only 2 doses were tested, whereas a minimum of three analysable doses is requested by the current test guideline. A positive control group was not included. Historical control data is missing. The positive responses observed were found only in presence of significant apoptosis, as indicated by caspase-3 levels. The response was only slight, since the proportion of DNA tail, which is the recommended parameter according to OECD TG 489, was not significantly increased in low-dose group and only 1.6 fold increased in the high-dose group, when compared to vehicle control animals.
Bollu, V.S. et al. (2016):
The test material is insufficiently characterised. Detail on test animals missing, including body weight and clinical signs during treatment. Description of the comet methodology lacks details. Blood sampling was performed after 36 h after the last exposure, whereas sampling 2-6 h after the last exposure is recommended by the current test guideline. The number of cells scored for DNA damage (100 per animal) is lower than recommended by the current OECD TG 489 (≥150 per animal). DNA damage was not evaluated using the recommended parameter (proportion of DNA in tail) but only using tail length. The test material was not tested up to toxic concentrations. Cytotoxicity was not evaluated for the comet assay, the micronucleus test performed concurrently, however, showed no cytotoxic effects. A positive control group was not included. The results were not related to a negative control group. Historical control data is missing. Interestingly, the authors evaluated the test material to be negative in the comet assay, based on the lack of significant differences between pre-treatment and treated animals. However, the values of tail length are apparently increased in a dose-related manner.
Ghosh, M. et al. (2016):
The test material was insufficiently characterised. The test material was administered via intraperitoneal injection, which, however, is considered as an unphysiological exposure route. The description of the animal treatment lacks details. Information on animal weight and age do not fit according to usual growth charts. Information on clinical signs or body weight loss in ZnO NP-treated animals is not provided. The comet assay methodology was not described. The number of cells scored for DNA damage is not specified. The positive control group results were not specified. Historical control data is missing. The significant increase in liver cells of low-dose groups is considered to be a chance finding and indicates that the methodology is not under control.
Yousef, J.M. et al. (2015):
The test material is insufficiently characterised. Description of the comet methodology is very poor. Only one dose group was tested, whereas OECD TG 489 requests testing of at least three dose groups. The number of comets scored for DNA damage is not specified. Positive control animals are not included. Information on animals and animal housing missing. Historical control data is missing. Positive responses in DNA damage were found only in presence of toxicity indicated by significantly and markedly increased caspase-3, LDH, ALT, and AST levels.
Pati, R. et al. (2016):
The test material is insufficiently characterised. The description of the test material preparation lacks details. Exposure and sampling schedule are unclear. The γH2AX assay methodology is insufficiently described. The body weight, sex, and number of test animals treated is unknown. Number of cells scored for γH2AX-positive stain was low (100 cells per animals). Positive control was not included. Results of γH2AX assay in blood cells not shown. Both doses tested resulted in animal death and clinical signs as well as significant inflammation.
3 Conclusion
No positive Ames result have been observed for coated and uncoated nano zinc oxide in a range of guideline compliant bacterial reverse mutation tests. This is supported by a larger number of studies of lower quality. The negative findings in the bacterial mutation assays have also been confirmed in in vitro mutagenicity tests in mammalian cells (tk assays), in which an ambiguous result was concluded only due to positive effects seen at concentrations with pronounced cytotoxicity. The weak positive responses were primarily seen at high test concentrations in which the findings were confounded by elevated levels of cytotoxicity. Positive responses manifested predominantly in formation of small colonies, which is indicative for a clastogenic event.
Clastogenicity (chromosome breakage) can often be caused by oxidative damage, or by indirect mechanisms such as excessive cytotoxicity, disruption of non-DNA targets etc. The lack of clastogenic potential of coated and uncoated nano-size zinc oxide in vitro has been shown in a number of highly relevant chromosomal aberration and micronucleus assays. An overall equivocal outcome is concluded only for studies which are considered not reliable. Any positive in vitro findings have also been satisfactorly addressed by two in vivo micronucleus tests after 14-day inhalation in rats with coated and uncoated nano-size zinc oxide. No increase in MN frequency was observed in the peripheral blood.
Potential site of contact genotoxicity has been addressed by a 14-day inhalation (nose-only) comet assay in rats, showing a pronounced inflammatory response after inhalation of 8 mg/m³ coated and uncoated nano zinc oxide (expressed in increased LDH and TP levels) but no elevated levels of %DNA in tail. It is therefore concluded that zinc oxide does not elicit site of contact genotoxicity.
Site-of-contact genotoxicity evaluation in lung epithelial cells and nasal mucosa cells, as well as systemic genotoxicity evaluation in liver and bone marrow has been addressed by a 14-day inhalation (whole-body) comet assay in rats. Pronounced concentration-related inflammatory response after inhalation of 0.5, 2 and 8 mg/m³ uncoated nano Zinc oxide (T0420) and coated nano Zinc oxide (T0421) (total protein, gamma-glutamyl-transferase; lactate dehydrogenase; alkaline phosphatase and beta-N-acetyl glucosaminidase (not for T0421) but no elevated levels of %DNA in tail were shown. It is therefore concluded that uncoated nano Zinc oxide (T0420) and coated nano Zinc oxide (T0421) do not elicit site of contact or systemic genotoxicity.
Based on the entire database of genetic toxicity studies it is concluded that in summary, coated and uncoated nano zinc oxide does not elicit any mutagenic activity either in bacterial or mammalian test systems. However, they induce some genotoxic effects in vitro, mainly manifest as DNA strand or chromosome breaks. A weight-of-evidence approach was applied, considering guideline compliant negative in vivo clastogenicity and site of contact comet assay studies. It is concluded that no genetic toxicity is observed with relevance for humans from coated and uncoated nano-size zinc oxide.
Justification for classification or non-classification
Based on the weight of the evidence from the existing in vitro and in vivo genotoxicity assays as presented and discussed above, it is concluded that coated and uncoated nano-size zinc oxide does not have biologically relevant genotoxic activity. Consequently, no classification for germ cell mutagenicity is applicable.
This conclusion is in line with those achieved by other regulatory reviews of the genotoxicity of zinc compounds (WHO, 2001; SCF, 2003; EU RAR, 2004, MAK, 2009). Hence, no classification and labelling for mutagenicity is required.
Information on Registered Substances comes from registration dossiers which have been assigned a registration number. The assignment of a registration number does however not guarantee that the information in the dossier is correct or that the dossier is compliant with Regulation (EC) No 1907/2006 (the REACH Regulation). This information has not been reviewed or verified by the Agency or any other authority. The content is subject to change without prior notice.
Reproduction or further distribution of this information may be subject to copyright protection. Use of the information without obtaining the permission from the owner(s) of the respective information might violate the rights of the owner.
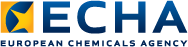