Registration Dossier
Registration Dossier
Data platform availability banner - registered substances factsheets
Please be aware that this old REACH registration data factsheet is no longer maintained; it remains frozen as of 19th May 2023.
The new ECHA CHEM database has been released by ECHA, and it now contains all REACH registration data. There are more details on the transition of ECHA's published data to ECHA CHEM here.
Diss Factsheets
Use of this information is subject to copyright laws and may require the permission of the owner of the information, as described in the ECHA Legal Notice.
EC number: 442-080-3 | CAS number: -
- Life Cycle description
- Uses advised against
- Endpoint summary
- Appearance / physical state / colour
- Melting point / freezing point
- Boiling point
- Density
- Particle size distribution (Granulometry)
- Vapour pressure
- Partition coefficient
- Water solubility
- Solubility in organic solvents / fat solubility
- Surface tension
- Flash point
- Auto flammability
- Flammability
- Explosiveness
- Oxidising properties
- Oxidation reduction potential
- Stability in organic solvents and identity of relevant degradation products
- Storage stability and reactivity towards container material
- Stability: thermal, sunlight, metals
- pH
- Dissociation constant
- Viscosity
- Additional physico-chemical information
- Additional physico-chemical properties of nanomaterials
- Nanomaterial agglomeration / aggregation
- Nanomaterial crystalline phase
- Nanomaterial crystallite and grain size
- Nanomaterial aspect ratio / shape
- Nanomaterial specific surface area
- Nanomaterial Zeta potential
- Nanomaterial surface chemistry
- Nanomaterial dustiness
- Nanomaterial porosity
- Nanomaterial pour density
- Nanomaterial photocatalytic activity
- Nanomaterial radical formation potential
- Nanomaterial catalytic activity
- Endpoint summary
- Stability
- Biodegradation
- Bioaccumulation
- Transport and distribution
- Environmental data
- Additional information on environmental fate and behaviour
- Ecotoxicological Summary
- Aquatic toxicity
- Endpoint summary
- Short-term toxicity to fish
- Long-term toxicity to fish
- Short-term toxicity to aquatic invertebrates
- Long-term toxicity to aquatic invertebrates
- Toxicity to aquatic algae and cyanobacteria
- Toxicity to aquatic plants other than algae
- Toxicity to microorganisms
- Endocrine disrupter testing in aquatic vertebrates – in vivo
- Toxicity to other aquatic organisms
- Sediment toxicity
- Terrestrial toxicity
- Biological effects monitoring
- Biotransformation and kinetics
- Additional ecotoxological information
- Toxicological Summary
- Toxicokinetics, metabolism and distribution
- Acute Toxicity
- Irritation / corrosion
- Sensitisation
- Repeated dose toxicity
- Genetic toxicity
- Carcinogenicity
- Toxicity to reproduction
- Specific investigations
- Exposure related observations in humans
- Toxic effects on livestock and pets
- Additional toxicological data

Hydrolysis
Administrative data
Link to relevant study record(s)
Description of key information
Negligible.
Key value for chemical safety assessment
Additional information
Hydrolysis is a common degradation route in the environment, namely the reaction involving a substance and water in a net exchange of the X with the OH group, in the following reaction: RX + H2O → ROH + HX.
Hydrolysis is often dependent upon pH and the reaction is commonly driven by hydrogen or hydroxide ions. Hydrolysable organic groups can react with water like Esters, Anhydrides, Amides, Carbammates, Nitriles, Cyanates, Epoxides, Halomethanes, Alkylhalides, Urea.
Literature data
In solution, linear polyphosphates undergo slow hydrolysis. This process continues as the shorter chain phosphates break down further to yield still shorter chain polyphosphates, metaphosphates and orthophosphates. Under neutral pH and normal room temperatures, this hydrolysis is relatively slow. At 20 °C and a pH of 7, about 50 % of pyrophosphate (the shortest polyphosphate) will revert to orthophosphate in 12 years. At 50 °C, the half-life is reduced to two months [1].
In the HERA document [2] on tripolyphosphate (STPP) a predicted half-life is reported in the order of years of STPP at pH 7-8 and at 20 °C in sterile water. The STPP and pyrophosphate (PP) compounds hydrolysed 100-1000 times faster in sterile lake water than in distilled water, and about 5-10 times slower in sterile algal media than in sterile lake water. The increase of the hydrolysis rates was attributed to dissolved substances such as calcium. In sterile medium triphosphate hydrolysed slightly quicker than PP. STPP half-lives in sterile lake water and algal medium ranged between 83 h and 608 h at 23 °C. The rates of hydrolysis of STPP and PP in non-sterile media at 25 °C were also determined by Clesceri and Lee [3] in two media of lake water supplemented or not with glucose; the results showed that compounds were hydrolysed in orthophosphate in a period of several days. Addition of glucose increased the rate of hydrolysis, indicating that microbial activity was one of the primary mechanisms of hydrolysis. The mechanism of the hydrolysis of condensed phosphate was investigated [4] and it was concluded that there are two processes for the hydrolytic degradation of the polyphosphates: one is the hydrolysis into shorter chains, the other is the degradation into trimetaphosphate. When polyphosphate are dissolved in water, the folding of the chain into a helix occurs with a random number of PO4 tetrahedra in the repeating units. Both processes are pH dependent.
The water hydrolysis is a first order reaction with a rate constant in the order to the 10 -4/10-5 min-1 and generally, lower pH and higher temperatures will increase the rate of hydrolysis in water.
Further studies about hydrolysis in soil are available on polyphosphates. A study conducted on the solid and liquid ammonium polyphosphate (APP) in four soils at three temperature regimes reported that rates were lower at 10 °C than at 20 °C or 30 °C. The amount of soil phosphorus hydrolyzed in the three acid soils decreased with increasing chain length although there were no significant differences between PP and tripolyphosphate [5]. Chang and Racz [6] confirmed that rates increased linearly and increased about two- to three-fold from 20 °C to 35 °C. Tripolyphosphate hydrolysis was found to be greater than PP and both rates were higher in the non-calcareous soil. About 40-70 % of the phosphate hydrolyzed in 120 hours at 20 °C whereas about 80-95 % hydrolyzed in 120 hours at 35 °C. Minerals may also affect hydrolysis rate.
Melamine can be hydrolysed in strong alkaline and acidic solutions to Ammeline (CAS No. 645-92-1). The rate constants at 100 °C are: k (s-1) = 3.80 x 10-6 [OH-] respectively k(s-1) = 1.25 x 10-4 [H+] [7 -8]. Considering that in the environment the pH's of most rivers and lakes are between 5 and 9 and that conditions of extreme pHs are unlikely to occur, it can be considered the hydrolysis reaction of the melamine as negligible in the environmental and fate context; on the contrary this information may be relevant from a toxicological viewpoint.
The EU Risk Assessment Report on Piperazine [9] reportes that in a study on the biotic degradation of piperazine [9 -10] a sterile control (kept in darkness) showed no degradation during the test period, indicating that the compound is persistent to hydrolysis. There is also information on the stability of piperazine under highly acidic and alkaline conditions, respectively, which implies that no hydrolytic degradation takes place [9 -11]. As suggested into the RAR document, in the absence of standard data on hydrolytically degradation the conservative assumption that piperazine is hydrolytically stable is assumed.
Experimental supporting data
In order to confirm the expected behaviour of the substance to be registered, a titration was performed with HCl 0.1 N and NaOH 0.1 N. In fact, the titration curve may highlight the possibility of the substance to generate free acids or free basis. In both the cases of the acid and the base curves, no inflection point were recorded demonstrating the absence of hydrolysed groups.
Conclusion
In conclusion, the hydrolysis results to be a negligible abiotic degradation process in environmental conditions.
Reference
[1] “The Use of Phosphates For Potable Water Treatment” publication of The Phosphate Forum of the Americas.
[2] HERA Targeted Risk Assessment of Sodium Tripolyphosphate (STPP) – June 2003
[3] Clesceri N.L. and Lee G.F. (1965b) Hydrolysis of Condensed Phosphates – I : Non-Sterile Environment, Int. J. Air Wat. Poll. 9, 723-742.
[4] The mechanism of the Hydrolysis of Condensed Phosphates. II. The Mechanism of the degradation of long-chain polyphosphate. Watanabe M., Sato S. And Saito H. Bullettin of the chemical society of Japan, vol. 48 (3), 896-898 (1975).
[5] Dick R.P. and Tabatabai M.A. (1986) Hydrolysis of polyphosphates in soils. Soil Sci 142:132–140
[6] Chang, C. and Racz, G. J. 1977. Effects of temperature and phosphate concentration on rate of sodium pyrophosphate and sodium tripolyphosphate hydrolysis in soil. Can. J. Soil Sci. 57: 211-278.
[7] OECD SIDS Melanine CAS N. 108 -78 -1. UNEP Publications.
[8] Gmelins Handbuch der Anorganischen Chemie, 14 Kohlenstoff, Teil D1, page 361, Verlag Chemie, 8th edition, 1971.
[9] European Union Risk Assessment Report. PIPERAZINE. 3rd Priority List Volume: 56. Consumer Protection European Chemicals Bureau. 2005.
[10] Emtiazi G and Knapp JS (1994). The biodegradation of piperazine and structurally-related linear and cyclic amines. Biodegradation 5, 83-92.
[11] Lightbody S and Thomson AK (1998). Validation of Analytical Methodology for Piperazine Salts. Invernesk Research, Scotland.
Information on Registered Substances comes from registration dossiers which have been assigned a registration number. The assignment of a registration number does however not guarantee that the information in the dossier is correct or that the dossier is compliant with Regulation (EC) No 1907/2006 (the REACH Regulation). This information has not been reviewed or verified by the Agency or any other authority. The content is subject to change without prior notice.
Reproduction or further distribution of this information may be subject to copyright protection. Use of the information without obtaining the permission from the owner(s) of the respective information might violate the rights of the owner.
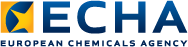