Registration Dossier
Registration Dossier
Data platform availability banner - registered substances factsheets
Please be aware that this old REACH registration data factsheet is no longer maintained; it remains frozen as of 19th May 2023.
The new ECHA CHEM database has been released by ECHA, and it now contains all REACH registration data. There are more details on the transition of ECHA's published data to ECHA CHEM here.
Diss Factsheets
Use of this information is subject to copyright laws and may require the permission of the owner of the information, as described in the ECHA Legal Notice.
EC number: 202-851-5 | CAS number: 100-42-5
- Life Cycle description
- Uses advised against
- Endpoint summary
- Appearance / physical state / colour
- Melting point / freezing point
- Boiling point
- Density
- Particle size distribution (Granulometry)
- Vapour pressure
- Partition coefficient
- Water solubility
- Solubility in organic solvents / fat solubility
- Surface tension
- Flash point
- Auto flammability
- Flammability
- Explosiveness
- Oxidising properties
- Oxidation reduction potential
- Stability in organic solvents and identity of relevant degradation products
- Storage stability and reactivity towards container material
- Stability: thermal, sunlight, metals
- pH
- Dissociation constant
- Viscosity
- Additional physico-chemical information
- Additional physico-chemical properties of nanomaterials
- Nanomaterial agglomeration / aggregation
- Nanomaterial crystalline phase
- Nanomaterial crystallite and grain size
- Nanomaterial aspect ratio / shape
- Nanomaterial specific surface area
- Nanomaterial Zeta potential
- Nanomaterial surface chemistry
- Nanomaterial dustiness
- Nanomaterial porosity
- Nanomaterial pour density
- Nanomaterial photocatalytic activity
- Nanomaterial radical formation potential
- Nanomaterial catalytic activity
- Endpoint summary
- Stability
- Biodegradation
- Bioaccumulation
- Transport and distribution
- Environmental data
- Additional information on environmental fate and behaviour
- Ecotoxicological Summary
- Aquatic toxicity
- Endpoint summary
- Short-term toxicity to fish
- Long-term toxicity to fish
- Short-term toxicity to aquatic invertebrates
- Long-term toxicity to aquatic invertebrates
- Toxicity to aquatic algae and cyanobacteria
- Toxicity to aquatic plants other than algae
- Toxicity to microorganisms
- Endocrine disrupter testing in aquatic vertebrates – in vivo
- Toxicity to other aquatic organisms
- Sediment toxicity
- Terrestrial toxicity
- Biological effects monitoring
- Biotransformation and kinetics
- Additional ecotoxological information
- Toxicological Summary
- Toxicokinetics, metabolism and distribution
- Acute Toxicity
- Irritation / corrosion
- Sensitisation
- Repeated dose toxicity
- Genetic toxicity
- Carcinogenicity
- Toxicity to reproduction
- Specific investigations
- Exposure related observations in humans
- Toxic effects on livestock and pets
- Additional toxicological data

Endpoint summary
Administrative data
Description of key information
- human: effects on colour vision after long-term inhalation: NOAEC = 50 ppm (8-hr TWA) (Seeber et al., 2009)
Inhalation:
- human: ototoxicity after long-term inhalation: NOAEC = 20 ppm (Triebig et al., 2009)
- rat: ototoxicity after long-term inhalation: NOAEC = 500 ppm (Lataye et al., 2005)
- rat: developmental toxicity after long-term inhalation: NOAEC = 500 ppm (Cruzan et al., 2005a, b)
Dermal: corrected NOAEL = 615 mg/kg/d (Triebig et al., 2009), resulting from route-to-route extrapolation (inhalation to dermal route)
Key value for chemical safety assessment
Additional information
- Influence of exposure duration on NOAEC
- Sensitivity of histopathological and (electro)physiological effects
- Anatomical/histological differences of target tissues between rats and humans
Repeated Dose Toxicity
In the UK RAR (June 2008) the data on repeated dose toxicity were summarized as follows (the references are given in brackets):
STUDIES IN ANIMALS
Summary of repeated inhalation exposure studies in rats
A variety of repeated inhalation exposure studies in rats have been conducted. Two well-characterised target sites have been identified: the nasal epithelium and the ear. In relation to nasal epithelium damage, a NOAEC has not been identified, with chronic inflammatory changes (mild in degree and confined to the olfactory epithelium with no effects on Bowman’s glands or olfactory nerve fibres), having been seen with long-term daily exposure to 50 ppm styrene, the lowest concentration explored in a 2-year study (Cruzan et al., 1998). Nasal lesions have also been observed after 13 weeks of exposure at 500 ppm (Cruzan et al., 1997) and above and in the high exposure group (500 ppm) of the F0 and F1 generations (treated approximately for 10 weeks) in a recent 2-generation study (Cruzan et al., 2005a). It has been shown that the cytochrome P450-mediated metabolism of styrene to the reactive metabolite styrene oxide (Green, 1999c; Green et al., 2001b; Cruzan et al.,2009) and to other reactive metabolites (e.g. the downstream metabolites of 4-vinylphenol) is a crucial factor in the expression of nasal epithelium toxicity. It is important to consider that cytochrome P450-catalysed metabolic activation of styrene to styrene oxide in human nasal epithelial tissue appears to be negligible (see toxicokinetics section) (Green, 1999c; Green et al., 2001b). In relation to 4-VP and its downstream metabolites, studies with rat, mouse and human lung microsomes have shown that the relative formation rates of these chemical species indicate that the mouse ability to produce these metabolites is greater than in the rat (14-79% of the mouse concentrations) and even greater than in humans (1.5-5% of the mouse concentrations) (Carlson et al., 2001; Bartels et al., 2004).
A similar toxification of styrene via 4-VP and its downstream metabolites may also be presumed to be mediated by CYP2F2 in the nasal tissue especially of mice. Hence, it can be concluded that the nasal epithelium damage induced by styrene in rats is not of relevance in relation to the potential repeated dose effects of styrene in humans at relevant levels of exposure.
Detoxification of styrene oxide is more efficient in human and rat nasal tissues as compared with mice. For example while human nasal tissue does not appear to possess any or very little P450 (activation) capacity, it does possess epoxide hydrolase activity, which is about 3-fold higher than that measured in mouse nasal tissues. The activity of epoxide hydrolase for both of the isomers in rat nasal tissue is higher (by a factor of approximately 10) than in the mouse, especially in the olfactory epithelium. Thus, while both mouse and rat nasal tissues appear to be equally efficient at “activating” styrene to styrene oxide, the rat nasal tissue is more efficient at deactivating/detoxifying styrene oxide. Glutathione conjugation appears to be an important detoxification pathway in both rat and mouse nasal tissue with glutathione-S-transferase activity in rat nasal tissue being about 3-fold higher than the mouse (Green, 2000c; Green et al., 2001b).
In relation to effects on the ear, clear evidence of ototoxicity (both functional and histological) has been seen in sedentary/ordinary rats repeatedly exposed to styrene by inhalation at concentrations of 600 ppm and above (Albee et al.,1992; Rebert et al., 1993; Loquet et al., 1999; Lataye et al., 2005; Mäkitie et al., 2002; 2003). In three different studies, no such effects were seen at 200 ppm (13 weeks) (Albee et al.,1992), 300 ppm (Mäkitie et al., 2003) or 500 ppm (Rebert et al., 1993; Loquet et al., 1999; Lataye et al., 2005), although at the concentrations of 300 and 500 ppm the studies were only of four weeks’ duration. Lataye et al., 2005 observed no hearing deficits at 500 ppm but by histopathology some loss of hair cells in the cochlea was observed. Therefore, the NOAEC (based on histopathology) is in the range of 500 ppm or slightly below in this experiment. One study in active rats exposed to styrene for 4 weeks showed that styrene-induced ototoxicity tend to occur at lower exposure concentrations than those at which ototoxicity is observed if sedentary/ordinary rats are employed. This is considered to be due to the increased styrene uptake, which is the consequence of the increased ventilation rate and, in turn, of the increased physical activity. In this study ototoxic effects were seen at 400 ppm and above, but not at 300 ppm, while a small hair cell loss in row 3 of the cochlea was still observed (Lataye et al., 2005). Ototoxicity has been seen at similar exposure levels with other aromatic organic solvents, such as toluene (Loquet et al., 1999; Johnson and Nylen, 1995) and xylene (Nylen et al., 1987; Pryor et al, 1987; Johnson and Nylen, 1995). The underlying toxicological mechanism has not been clearly elucidated. This effect should be therefore regarded as of potential relevance to human health. The histological damage consists of the destruction of the outer hair cells (OHC; especially of row 3) of the cochlea (e.g. Loquet et al., 1999; Lataye et al., 2005). These changes are accompanied by an elevation of the hearing thresholds in the mid-frequency range (10-20 kHz) e.g. (Loquet et al., 1999; Lataye et al., 2005). The destruction of the hair cells is irreversible and occurs at slightly lower exposure concentrations than those producing the audiometric hearing threshold shifts (Loquet et al., 1999; Lataye et al., 2005). Mechanistic investigations indicate that styrene reaches the sensory hair cells of the cochlea via the blood stream (Campo et al., 1999) and that styrene itself and/or its metabolites cause a serious disturbance of the membranous organisation of these target cells (Campo et al., 2001). A number of studies suggest that styrene-induced ototoxicity can be potentiated by exposure to noise (Mäkitie et al., 2003; Lataye et al., 2000; 2005). The available evidence also shows that ototoxicity appears after relatively short exposures (1 week) and that continued treatment (4 weeks up to 19 months for toluene according to Nylen et al., 1987) does not enhance the intensity of the ototoxic response (Campo et al., 2001). On this basis, it is considered that the NOAEC values of 500 and 300 ppm for 4 weeks in sedentary/ordinary and active rats respectively are a more accurate starting point for risk characterisation than the NOAEC of 200 ppm for 13 weeks.
There is no convincing evidence for repeated inhalation exposure to styrene producing damage to, or dysfunction of the central nervous system in rats (Albee et al., 1992; Yamamoto et al., 1997; Teramoto et al., 1993; Seppäläinen, 1978; Savolainen et al., 1980). CNS depression (narcosis) has been observed, but this was a transient and reversible acute effect rather than the consequence of repeated exposure (Savolainen and Pfäffli, 1977; Fumiko, 1988; Kulig, 1989; Coccini et al., 1999). Variable results have been obtained in studies investigating elements of brain biochemistry (Mutti et al., 1984; Coccini et al., 1996; 1999; Savolainen et al., 1980; Rosengren and Haylid, 1989; Savolainen and Pfäffli, 1977), but none of the differences detected between styrene-exposed and control animals are interpretable within an established framework of well-validated neurotoxicological criteria.
In one single non-standard investigation (Vettori et al., 2000), rather minor effects on the number of the large amacrine cells and on the content of neuramines and glutathione of the retina of rats exposed repeatedly to 300 ppm styrene for 12 weeks have been reported. However, it is considered that, in the absence of any associated conventional histopathology and given the lack of information on what are the ‘normal’ levels of dopamine and glutathione in the retinal cells of rats, the rather minor morphometric and biochemical changes observed are unlikely to represent a toxic response.
A literature search from 1998 to January 2010 overlapping the date of the UK RAR (June 2008) was conducted. Overall, this did not lead to a modification of the conclusions reached by the UK RAR and reinforced some of the aspects elaborated above.
Related to nasal toxicity:
The metabolism of styrene in relation to the mode of action for lung carcinogenicity in mice and nasal toxicity in mice and rats has been reviewed by Cruzan et al. (2009). This review included a comparison with other chemicals with a similar toxicological profile (coumarin, naphthalene, ethylbenzene, cumene, divinylbenzene, benzofuran). The authors concluded that the effects noted for these chemicals, especially also for styrene, is driven by CYP2F isoforms of the cytochrome P450 family, namely CYP2F2 in nasal and lung tissues of mice and CYP2F4 in nasal tissues of rats. Importantly, the CYP2F1 isozyme expressed in humans has a low/no capacity to metabolize styrene. Metabolites resulting from the action of CYP2F4 in rats are cytotoxic leading to nasal toxicity. Inhibition of these CYP2F4 by 5-phenyl-1-pentyne prevented these effects (Green, 1999c).
Related to ototoxicity:
Campo et al., (2007) investigated the underlying mechanism for the synergistic effects on hearing of a simultaneous exposure to noise and aromatic solvents, including styrene. After i.v. application of the aromatic solvent it was found that the solvent can inhibit the protective middle and inner ear acoustic reflexes of the middle ear muscles. This acoustic reflex is elicited by intense sounds and serves to prevent cochlear damage by diminishing the penetration of high acoustic energies into the inner ear.
An update of the literature until May 2022 provided the following new information:
Campo et al. (2011) studied age related hearing loss in rats which similar to humans, lose sensitivity to high frequencies faster than to medium frequencies. Rats at the age of 6 months were either exposed to noise at 85 dB or to styrene at 300 ppm (6 h/d, 5 d/week over 4 weeks). Hearing capacity was measured by otoacoustic emissions, histology of the cochlea (light and transmission electron microscopy), and density calculation of the spiral ganglion at of 6, 8, 12, 18, and 24 months of age. Normal aging is accompanied by decreased otoacoustic emissions, outer hair cell loss in the cochlea, and cell loss in the spiral ganglion. In comparison to control rats, noise exposure led to reduced amplitudes of otoacoustic emissions and reduced cell density of spiral ganglions while no effects were noted for the cochlea. After styrene exposure no clear effects were noted for the otoacoustic emissions or the cochlea, while there was a cell loss in the spiral ganglion after 24 months. The authors concluded that for the aging rat there was a clear effect on hearing loss by noise while the effects of styrene were less conclusive. But the findings for the spiral ganglion after 24 months may be indicative for a possible neurotoxic, rather than ototoxic effect.
Campo et al. (2014) studied the effect of continuous (85 dB) and impulse noise (80 dB) with or without coexposure to styrene (300 ppm) on hearing ability in rats by otoacoustic emissions and histology of the cochlea. The animal were exposed 6 h/d, 5 d/week over 4 weeks and effects on hearing were measured after 4 weeks of recovery. Exposure of styrene or noise (continuous and impulse) led to reductions of otoacoustic emissions. Unexpectedly, coexposure to styrene and continuous noise led to an attenuation of the effects while coexposure to impulse noise potentiated the traumatic impact. As regards histology of the cochlea, styrene plus continuous noise did not lead to alterations compared to unexposed rats. Impulse noise was more damaging that continuous noise and styrene potentiated the effect of impulse noise. Thus, coexposure with styrene was less damaging than continuous noise alone, but enhanced the traumatic effect of impulse noise.
Related to unspecific neurotoxic effects:
Gagnaire et al. (2006) did not find any effects on extracellular and tissue levels of dopamine, serotonin and their metabolites in the brain of rats exposed to 750 and 1000 ppm over 4 weeks (6 h/d; 5 d/week). They concluded that an exposure level of 50 ppm is unlikely to cause a disruption of the mechanisms controlling the extracellular concentrations of dopamine or serotonin.
When rats were exposed to 300 ppm (6h/d, 5 d/week over 2 weeks) the animals were slightly somnolent with a crouched posture and closed eyes during treatment, but these effects rapidly disappeared in the post exposure period (De Piceis Polver, et al., 2003). This finding confirms that inhalation exposure may lead to an acute reversible depression of the central nervous system.
Summary of repeated inhalation exposure studies in mice
As with acute toxicity, the mouse as a species is peculiarly sensitive to toxicity arising from repeated inhalation exposure to styrene, although there is some variation between different strains. Three well characterised target sites have been identified: the lung, the nasal epithelium and the liver.
In relation to the lung, a NOAEC has not been identified, with damage to the lung epithelium (hyperplasia in the terminal bronchioles, focal bronchiolar-alveolar hyperplasia, fibrosis extending to the alveolar ducts and extension of non-ciliated cells from the terminal bronchiole to the alveolar duct) having been seen on long-term exposure of mice to 20 ppm, the lowest exposure concentration tested (Cruzan et al.,2001). Nasal epithelium damage (respiratory metaplasia of the olfactory epithelium, hyperplasia and hypertrophy of the Bowman’s gland, and atrophy of the olfactory nerve fibres) also appeared at 20 ppm (the lowest exposure level tested) and above (Cruzan et al.,2001). Work with the cytochrome P450 inhibitor 5-P-1-P has shown that metabolic activation of styrene to styrene oxide and other reactive metabolites (e.g the downstream metabolites of 4-vinylphenol) and subsequent detoxification of styrene oxide are crucial elements of the mouse respiratory tract toxic response (Foster, 1999b; Green, 1999c; 1999d; Green et al., 2001a; 2001b). In this context it is important to consider that cytochrome P450-catalysed metabolic activation of styrene in human respiratory tract tissue appears to be negligible (see toxicokinetic section). Besides these metabolic differences, there are also significant anatomic and histological differences in the respiratory tract of mice and humans. Hence, it can be concluded that these respiratory tract findings in mice reflect a toxic process that will not occur to any significant extent in humans at relevant levels of exposure.
Exposures in the range 200-250 ppm have also produced fatal hepatotoxicity in mice (Morgan et al., 1993a; 1993c; 1995). Again, it appears that the metabolic activation of styrene to styrene oxide is a crucial stage in the hepatotoxic response. In this context, it is evident that the toxicokinetic information available suggests that the mouse would be more susceptible than humans to styrene-induced hepatotoxicity. This is borne out by the observation of mouse fatalities at exposure levels to which humans have been regularly exposed in the past, with no reports of hepatotoxicity or death.
Overall, the data suggests that the mouse is a poor model for predicting the effects of repeated inhalation exposure to styrene in humans.
A literature search from 1998 to January 2010 overlapping the date of the UK RAR (June 2008) was conducted. Overall, this did not lead to a modification of the conclusions reached by the UK RAR and reinforced some of the aspects elaborated above.
In vitro experiments were carried out by Carlson (2004) with microsomal preparations of mouse liver and lung in the presence of various cytochrome P450 inhibitors. A strong inhibition of the metabolism of 4-vinylphenol to unknown downstream metabolites was found by 5-phenyl-1-pentyne, an inhibitor of CYP2F2, especially for lung microsomes. There was good evidence that also other isozymes, not identified in this study, are involved in the metabolism of 4-vinylphenol.
Summary of Repeated Oral Exposure Studies in Animals
Most of the available repeated oral exposure studies have been performed in rats. Few of these are of the quality, breadth and thoroughness required in standard regulatory test guidelines. Hence, most of the data do not facilitate the derivation of an overall NOAEL of the type normally sought in relation to a 90 day (or longer) exposure period. However, there is reliable information from a carcinogenicity bioassay in the rat (NCI, 1979), in which there was no evidence of treatment related toxicity in animals administered 1000 mg/kg/day for two years; a marked increase in mortality was evident at 2000 mg/kg/day. In another 2 year study (Beliles et al., 1985), styrene did not produce any clear evidence of toxicity when administered in drinking water at a dose of 21 mg/kg/day (250 ppm in drinking water), the highest dose level used. However, it is noted that potential effects on the ear were not investigated in these studies. Ototoxicity, a clearly established effect of styrene in the rat, was seen in one study at 800 mg/kg/day (and perhaps, although less convincingly, at 400 mg/kg/day), for 2 weeks (Wang, 1998). A range of different CNS related observations have also been made with repeated oral dosing in this range (Zaprianov and Bainova, 1979; Husain et al., 1980; 1986; Agrawal et al., 1982; Bushnell, 1994; Khanna et al., 1994; Wang, 1998; Wang et al., 1998), but unfortunately each one stands in isolation and lacks a surrounding, well established and validated framework within which the results can be interpreted, and in some cases contradictory results have been reported in different studies. Overall, there is no convincing evidence of clear, styrene induced neurological lesions induced by repeated oral dosing in rats. Two studies by the same authors (Srivastava et al., 1989; 1992b) have reported testicular damage in rats at 200 and 400 mg/kg/day styrene. It is noted that in earlier repeated oral studies and in well conducted 2 year inhalation studies in rats at equivalent and higher doses than those used by Srivastava et al. no testicular changes or indications of any testicular effects were observed. Also, no effects on the testis and fertility parameters have been observed in a recent well-conducted OECD- and GLP-compliant rat inhalation 2-generation study with exposures up to 500 ppm (»300 mg/kg/day) styrene. Therefore, despite these individual publications by the same authors reporting testicular damage, the weight of evidence indicates that styrene is not a testicular toxicant.
In mice, the most reliable information comes from a cancer bioassay (NCI, 1979), in which increased mortality and hepatic necrosis were observed at the highest dose of 300 mg/kg/day, and a NOAEL of 150 mg/kg/day was identified. The one significant observation from the remaining studies is that of toxicity towards the lung epithelium (Green, 1999b), adding further support to the concept that the lung toxicity of styrene in mice, following oral and inhalation exposure, results from local metabolism of styrene to styrene oxide and other reactive metabolites (e.g. the downstream metabolites of 4-vinylphenol). The one report of styrene induced decrease in serum testosterone levels in young mice dosed with 12 mg/kg/day for 4 weeks (50 ppm in drinking water) is likely to be a chance finding (Takao et al., 2000).
A repeated oral study in dogs detected Heinz bodies in circulating erythrocytes (suggesting oxidative damage) at doses of 400 and 600 mg/kg/day, with minimal effects in females at 200 mg/kg (Quast et al., 1979).
Overall, in relation to repeated oral exposure, the NOAEL of 150 mg/kg/day identified from a 2 year cancer bioassay in the mouse should also be considered. But in extrapolation to humans careful consideration has to be taken of the specifics of mouse metabolism and the high sensitivity of this species for liver toxicity as compared to e.g. the rat.
A literature search from 1998 to January 2010 overlapping the date of the UK RAR (June 2008) was conducted. Overall, this did not lead to a modification of the conclusions reached by the UK RAR and reinforced some of the aspects elaborated above.
- Related to ototoxicity:
In a comparative oral study Gagnaire and Langlais (2005) investigated the ototoxicity of 21 aromatic solvents at a dose level of 8.47 mmol/kg bw/d (corresponding to 880 mg/kg bw/d) over two weeks (5 d/week). Loss of outer hair cells of the cochlea was used as parameter for ototoxicity. The following order of potency was obtained: alpha-methylstyrene < trans-beta-methylstyrene = toluene < p-xylene < n‑propylbenzene < styrene = ethylbenzene < allylbenzene.
After continuous oral application of 800 mg/kg bw to rats Chen et al. (2007) observed outer hair cell loss in the cochlea after 7 days but not after 5 days. Small losses of outer hair cells were already found after 3 weeks (5 d/week) at 200 mg/kg. It was demonstrated that Deiters cells are an especially vulnerable target of styrene. The authors further reported that the styrene concentrations in the cochlea varied along with the basilar membrane with the lowest level in the basal turn being consistent with the lowest styrene induced threshold shift and hair cell loss in this region.
Furthermore, after oral exposure of rats to styrene (200, 300, 400, and 800 mg/kg, 5 d/week over durations varying between 3 and 12 weeks), Chen et al. (2008) found that a loss of outer hair cells of <33% did not result in a significant shift of hearing threshold.
The protective effect of N-acetyl-L-cysteine (NAC) on styrene induced hearing impairment was studied by Yang et al., (2009). Rats orally exposed to 400 mg/kg bw/d styrene (5 d/week over 3 weeks) revealed audiometric threshold shifts and loss of outer hair cells by necrosis and apoptosis, the latter being the major pathway for cell death. Concomitant intraperitoneal application of NAC reduced styrene induced hearing loss and outer hair cell death. The authors suggest involvement of oxidative stress in generation of cochlear damage.
A new literature search up to March 2013 was carried out. The following papers were identified. Thereby some further indication for an interaction of styrene exposure and noise was obtained.
Chen & Henderson (2009) exposed rats to continuous noise (100 dB SPL, 6 h/d, 5 d/week over 3 weeks) superimposed by an impact noise (up to 110 dB SPL, duration 30 msec at a rate of 1/sec). 300 or 400 mg/kg bw styrene were given by gavage (5 d/week over 3 weeks). Cochlear compound action potential (CAP) served to assess hearing ability/loss and hair cells in the cochlea were examined by histopathology. The exposure levels to styrene only induced a limited loss of outer hair cells (OHC) without a significant hearing loss. Exposure to noise alone led to a clear hearing loss as measured by CAP but only to slight OHC losses. The combined exposures induced OHC and hearing losses that were greater than the sum caused by styrene or noise alone. Apoptosis of Deiters cells was investigated after an exposure of 7 days to noise and styrene (800 mg/kg bw). Again, a synergistic noise-styrene interaction was observed for OHC loss as well as for apoptosis of Deiters cells, although noise alone did not affect Deiters cells. Thus, this study shows that styrene leads to ototoxicity not only after inhalation but also after oral dosing. In addition, a styrene/noise synergism was demonstrated even at exposures that lead to no or only marginal effects on the parameters investigated by either noise or styrene alone.
- Related to testicular effects:
Chun et al. (2005) reported reduced sperm counts and motility and increased sperm abnormalities after oral treatment of adult rats (3 animals/group) with 0, 50, 100, and 500 mg/kg bw over 5 days. At 100 mg/kg testicular sperm head counts were significantly reduced and sperm head counts were numerically decreased in epididymis; the other parameters were significantly affected at 500 mg/kg. Weights of testes and epididymis did not show an exposure related effect. The authors suggested that these effects may be related to oxidative stress caused by styrene. Again, these results are not reflected by those obtained in well conducted long term OECD- and GLP compliant 2-generation studies.
Summary of Repeated Dose Toxicity Studies in Animals
A variety of repeated inhalation exposure studies in different animal species are available. However, among these species, the rat and mouse have been the most extensively investigated. Four well characterised target sites of toxicity have been identified: the nasal epithelium (in rats and mice), the lung (in the mouse), the liver (in the mouse) and the ear (in the rat).
In relation to nasal epithelium damage, a NOAEC has not been identified, with chronic inflammatory changes (mild in degree and confined to the olfactory epithelium with no effects on Bowman’s glands or olfactory nerve fibres), having been seen in the rat with long term daily exposure to 50 ppm styrene, the lowest concentration explored in a 2-year study. Nasal toxicity has also been reported in mice. In a similar 2-year study, respiratory metaplasia of the olfactory epithelium, hyperplasia and hypertrophy of the Bowman’s gland and atrophy of the olfactory nerve fibres were observed starting from 20 ppm, the lowest concentration tested. It is clear that the nasal lesions induced by styrene exposure are a lot more severe in the mouse compared to the rat. Over the years a number of investigative studies have been undertaken to characterise and explain these species differences and to investigate the relevance of these findings to humans. The results of these investigations have shown that:
1) Nasal toxicity in the mouse is reduced considerably by pre-treating the animals with 5-phenyl-1-pentyne (5-P-1-P), a specific inhibitor of cytochrome P450 2F2 (Green, 1999c; Green et al., 2001b).
2) Metabolism of styrene to styrene oxide (to both R- and S-isomers) by olfactory and respiratory microsomes is similar in the mouse and rat (approximately 7 nmol SO/min/mg protein). Examination of nine human nasal samples (8 as S9 fractions and 1 as a microsomal fraction) failed to detect metabolism to styrene oxide (detection limit 0.04 nmol SO/min/mg protein which indicates a difference between rodents and humans in the production of SO of at least 2 orders of magnitude) (Green, 1999c; Green et al., 2001b).
3) Detoxification of styrene oxide is more efficient in human and rat nasal tissues as compared with mice. For example while human nasal tissue does not appear to possess any or very little P450 (activation) capacity, it does possess epoxide hydrolase activity, which is about 3-fold higher than that measured in mouse nasal tissues. The activity of epoxide hydrolase for both of the isomers in rat nasal tissue is higher (by a factor of approximately 10) than in the mouse, especially in the olfactory epithelium. Thus, while both mouse and rat nasal tissues appear to be equally efficient at “activating” styrene to styrene oxide, the rat nasal tissue is more efficient at deactivating/detoxifying styrene oxide. Glutathione conjugation appears to be an important detoxification pathway in both rat and mouse nasal tissue with glutathione-S-transferase activity in rat nasal tissue being about 3-fold higher than the mouse (Green, 2000c; Green et al., 2001b).
4) Differences in the distribution of CYP2F2 in mouse and rat nasal tissues explain the histopathological differences seen in the mouse and rat. For example the mouse shows high levels of CYP2F2 expression in the apical cytoplasm of the olfactory tissue with low to moderate expression in some basal cells and in Bowman's glands. All of these cells show histopathological damage in the mouse after styrene exposure. Conversely in the rat such cells showed low expression of the metabolizing enzymes and no tissue damage (Green, 1999c, Green et al., 2001b).
5) 4-Vinylphenol (4-VP), a metabolite of styrene, that rapidly undergoes further metabolic degradation, has been shown to be pneumotoxic to metabolically competent cells such as Clara cells (Kaufmann et al, 2005) and presumably to nasal epithelial cells which also contain CYP2F2. As inhibition of CYP2F2 activity inhibits 4-VP toxicity in the lung (Carlson, 2002b), it appears that downstream metabolites of 4-VP rather than 4-VP itself are the cytotoxic species. Studies with rat, mouse and human lung microsomes have shown that the oxidative metabolites of 4-VP are ring hydroxylated and side-chain epoxide products of 4-VP and that the relative formation rates of these chemical species indicate that the mouse ability to produce these metabolites is greater than in the rat (14-79% of the mouse concentrations) and even greater than in humans (1.5-5% of the mouse concentrations) (Bartels et al., 2004). A similar toxification of styrene via 4-VP and its downstream metabolites may also be presumed to be mediated by CYP2F2 in the nasal tissue especially of mice.
6) Uptake of styrene by nasal tissue is enhanced by the ability of the tissue to metabolise styrene (Morris, 2000). The lack of the primary step of styrene metabolism in human nasal tissue serves as a further reason for dismissing the relevance to humans of these rodent nasal lesions.
7) Besides these metabolic differences, anatomically, there are significant differences in the nasal passages of rodents and humans, which result in significant different volumes and airflow patterns. Thus, although inhaled styrene may be deposited in the nasal passages of humans, it is likely that high levels will not be deposited in the olfactory area.
8) Human investigations have shown that exposure up to 77 ppm (8h TWA) styrene as occurring in the UP-resin industry is not associated with impairment of olfactory function (Dalton et al, 2003; see ‘studies in humans’, ‘studies on olfaction’).
Overall, it can be concluded that the differences in nasal toxicity between rat and mouse can be explained by the greater ability of the rat nasal epithelium to detoxify reactive metabolites of styrene formed via CYP2F2 metabolism. These reactive/toxic intermediates include styrene oxide and most probably the downstream metabolites of 4-VP. Detoxification of toxic species by epoxide hydrolase is 10-fold higher in the rat olfactory tissue as compared to the mouse while glutathione S-transferase activity is approximately 3-fold higher in the rat nasal tissue as compared to the mouse. It is important to consider that cytochrome P450-catalysed metabolic activation of styrene to styrene oxide in human nasal epithelial tissue appears to be negligible (see toxicokinetics section). Also, since uptake of styrene by nasal tissue is enhanced by the ability of the tissue to metabolise styrene, the lack of the primary step of styrene metabolism in human nasal tissue serves as a further reason for dismissing the relevance to humans of these rodent nasal lesions. Besides these metabolic differences, anatomically, there are significant differences in the nasal passages of rodents and humans, which result in significant different volumes and airflow patterns. Thus, although inhaled styrene may be deposited in the nasal passages of humans, it is likely that high levels will not be deposited in the olfactory area. Furthermore, human investigations have shown that exposure up to 77 ppm (8h TWA) styrene as occurring in the UP-resin industry is not associated with impairment of olfactory function, and in several human health surveys of workers repeatedly exposed to styrene up to approximately 700 ppm, no nasal lesions have been described (see RDT, human studies section). Hence, it can be concluded that rodent nasal epithelium damage induced by styrene is not of relevance in relation to the potential repeated dose effects of styrene in humans at relevant levels of exposure.
In relation to the lung, no effects were seen in rats exposed up to 1000 ppm, but in mice a NOAEC was not identified, with damage to the lung epithelium (hyperplasia in the terminal bronchioles, focal bronchiolar-alveolar hyperplasia, fibrosis extending to the alveolar ducts and extension of non-ciliated cells from the terminal bronchiole to the alveolar duct) having been seen from 20 ppm, the lowest exposure concentration tested in a 2-year study. Work with the cytochrome P450 inhibitor 5-P-1-P has shown that metabolic activation of styrene to styrene oxide and other reactive metabolites (e.g the downstream metabolites of 4-vinylphenol) and the subsequent detoxification of styrene oxide are crucial elements of this toxic response. This is supported by the observation that it is the metabolically active Clara cells that are the initial focus of damage. These non-ciliated bronchiolar epithelial cells are mainly involved in the metabolism of xenobiotics, but also in the secretion of surfactants and in the renewal process of the bronchiolar epithelium (Komaromy and Tigyi, 1988). Early biochemical changes, sustained cell damage and regenerative cell proliferation were observed in lung Clara cells of mice exposed to 40 and 160 ppm styrene for up to 4 weeks. In this context it is important to consider that cytochrome P450-catalysed metabolic activation of styrene in human lung tissue appears to be minimal (see toxicokinetic section) even though the two main P450 isoforms involved in styrene metabolism, CYP2E1 and CYP2F1, have been detected; that the number of Clara cells in human lung is very low; and that their most important fucntions in human lung are shared by other cell types (e.g. Type II cells). Hence, it can be concluded that these lung tissue findings in mice reflect a toxic response that will not occur to any significant extent in humans at relevant levels of exposure.
In relation to the liver, exposures in the range 200-250 ppm have produced fatal hepatotoxicity in mice. Again, it appears that the metabolic activation of styrene to styrene oxide is a crucial stage in the hepatotoxicity process. In this context, it is evident that the toxicokinetic information available suggests that the mouse would be more susceptible than humans to styrene induced hepatotoxicity. This is borne out by the observation of mouse fatalities at exposure levels to which humans have been regularly exposed in the past, with no reports of hepatotoxicity or death.
In relation to effects on the ear, clear evidence of ototoxicity (both functional and histological has been seen in sedentary/ordinary rats repeatedly exposed to styrene by inhalation at concentrations of 600 ppm and above. In three different studies, no such effects were seen at 200 ppm (13 weeks), 300 ppm or 500 ppm, although in the latter two cases the studies were only of four weeks’ duration and a small hair cell loss in row 3 of the cochlea was still observed at 500 ppm. One study in active rats exposed to styrene for 4 weeks showed that styrene-induced ototoxicity tend to occur at lower exposure concentrations than those at which ototoxicity is observed if sedentary/ordinary rats are employed. This is considered to be due to the increased styrene uptake, which is the consequence of the increased ventilation rate and, in turn, of the increased physical activity. In this study ototoxic effects were seen at 400 ppm and above, but not at 300 ppm apart from a small hair cell loss in row 3 of the cochlea. Comparative studies using rats and guinea pigs exposed to 1000 ppm for 5 days indicate an obvious species-difference, as similar findings were not observed in guinea pigs (Lataye et al., 2003). Ototoxicity has been seen at similar exposure levels with other aromatic organic solvents, such as toluene and xylene. The underlying toxicological mechanism has not been clearly elucidated. This effect should be regarded as of potential relevance to human health. The histological damage consists in the destruction of the outer hair cells (OHC; especially of row 3) of the cochlea. These changes are accompanied by an elevation of the hearing thresholds in the mid-frequency range (10-20 kHz). The destruction of the hair cells is irreversible and occurs at slightly lower exposure concentrations than those producing the audiometric hearing threshold shifts. Mechanistic investigations indicate that styrene reaches the sensory hair cells of the cochlea via the blood stream and that styrene itself and/or its metabolites cause a serious disturbance of the membranous organisation of these target cells. A number of studies suggest that styrene-induced ototoxicity can be potentiated by exposure to noise. The available evidence also shows that ototoxicity appears after relatively short exposures (1 week) and that continued treatment (4 weeks up to 19 months) does not enhance the intensity of the ototoxic response. On this basis, it is considered that the NOAEC values of 500 and 300 ppm for 4 weeks in sedentary/ordinary and active rats respectively are a more accurate starting point for risk characterisation than the NOAEC of 200 ppm for 13 weeks.
In one single non-standard investigation, rather minor effects on the number of the large amacrine cells and on the content of neuramines and glutathione of the retina of rats exposed repeatedly to 300 ppm styrene for 12 weeks have been reported. However, it is considered that, in the absence of any associated conventional histopathology and given the lack of information on what are the ‘normal’ levels of dopamine and glutathione in the retinal cells of rats, the rather minor morphometric and biochemical changes observed are unlikely to represent a toxic response.
Overall, the available inhalation repeated dose toxicity studies have identified ototoxicity as the most sensitive and relevant effect of styrene repeated inhalation exposure with NOAEC values of 500 ppm (2165 mg/m3) and 300 ppm (1300 mg/m3) for 4 weeks in sedentary/ordinary and active rats respectively.
Most of the available repeated oral exposure studies have been performed in rats and mice. Information from a carcinogenicity bioassay in the rat has shown no evidence of treatment related toxicity in animals administered 1000 mg/kg/day for two years; a marked increase in mortality was evident at 2000 mg/kg/day. In another 2-year study, styrene did not produce any clear evidence of toxicity when administered in drinking water at a dose of 21 mg/kg/day, the highest dose level tested. However it is noted that potential effects on the ear were not investigated in these studies. Ototoxicity, a clearly established effect of styrene in the rat, was seen in one study at 800 mg/kg/day (and perhaps, although less convincingly, at 400 mg/kg/day) for 2 weeks.
In mice, the most reliable information comes from a cancer bioassay, in which increased mortality and hepatic necrosis were observed at the highest dose of 300 mg/kg/day; a NOAEL of 150 mg/kg/day was identified from this study. The one significant observation from the remaining studies is that of toxicity towards the lung epithelium, adding further support to the concept that the lung toxicity of styrene in mice, following oral and inhalation exposure, results from local metabolism of styrene to styrene oxide and to other reactive metabolites (e.g. the downstream metabolites of 4-vinylphenol).
Overall, in relation to repeated oral exposure, the NOAEL of 150 mg/kg/day identified from a 2-year cancer bioassay in the mouse should also be considered. But in extrapolation to humans careful consideration has to be taken of the specifics of mouse metabolism and the high sensitivity of this species for liver toxicity as compared to e.g. the rat.
No repeated dermal studies are available, although low systemic toxicity would be predicted in most conventional experimental species with the possible exception of some strains of the mouse.
With regard to DNEL derivation based on ototoxicity three aspects have to be critically evaluated:
- influence of exposure duration on NOAEC
- sensitivity of histopathological and (electro)physiological effects
- anatomical/histological differences of target tissues between rats and humans.
Ototoxicity leading to an increase of audiometric threshold and specific histopathological alterations with destruction of the outer hair cells in the cochlea (predominantly in row 3) is not a specific effect only of styrene, but has been described for a variety of other aromatic solvents. In a comparative oral study such histopathological effects were observed in the following order of potency for alpha-methylstyrene < trans-beta-methylstyrene = toluene < p-xylene < n‑propylbenzene < styrene = ethylbenzene < allylbenzene (Gagnaire and Langlais, 2005). After inhalation exposure qualitatively similar hearing impairments were found by reflex modification audiometry for styrene, toluene and mixed xylenes (Crofton et al., 1994). The inhalation of styrene and toluene again led to similar electrophysiological and histopathological findings (Loquet et al., 1999). Permanent hearing loss as determined by behavioral and electrophysiological methods was also described after inhalation exposure to toluene (Pryor et al., 1984), mixed xylenes, and styrene (Pryor et al., 1987).
The weight of evidence obtained from experiments with styrene and other aromatic solvents indicates that ototoxicity is exerted by the lipophilic aromatic parent chemical rather than by a metabolite:
Similar ototoxic effects were found for a range of lipophilic organic solvents. As all of these chemicals are biotransformed to different metabolites, indirect evidence is thereby obtained that the parent chemicals themselves most probably lead to ototoxicity.
When rats were exposed to toluene, pre-treatment with phenobarbital prior to toluene inhalation resulted in a marked reduction in blood toluene levels and prevented hearing loss (Pryor et al., 1991) showing induction of metabolism reduces ototoxicity.
A comparative study of ototoxicity in rats and guinea pigs showed much higher blood levels of styrene in the rat (i.e. 23 µg/g) leading to ototoxicity, while in the guinea pig the blood levels were about 4-fold lower (i.e. about 5 µg/g) with no evidence of ototoxicity (Lataye et al., 2003). This finding compares well with that of Cappaert et al. (2002) attributing the low sensitivity of guinea pigs for ototoxicity against ethylbenzene to the low blood concentration of the parent chemical in comparison to rats.
Inhalation to toluene induced auditory impairment as determined by auditory brainstem response in rats but not in chinchillas under similar exposure conditions. This was explained by species differences in hepatic microsomal metabolism of toluene being highest in chinchillas (Davis et al., 2002).
No changes in auditory brainstem response were seen in rats treated with phenylglyoxylic acid, a major metabolite of styrene, in drinking water over 3 months at doses up to 5000 mg/l, corresponding to 293 mg/kg bw/d (Ladefoged et al., 1998).
The higher sensitivity of young rats as compared to old ones for ototoxicity was explained by Lataye et al. (2004) by age related increases in styrene metabolism.
In conclusion, the overall evidence indicates that the mechanism of aromatic solvent induced ototoxicity is very similar for these chemicals, notwithstanding different potencies, and driven by the parent compound and not by their hydrophilic metabolites. A read across approach is therefore indicated to fill specific data gaps for the risk assessment of styrene.
There is strong evidence that the maximum of hearing impairment is already reached after one to a few weeks of exposure and that ototoxicity does not increase with prolongation of the exposure period. Campo et al. (2001) exposed rats to 1000 ppm styrene (6h/d, 5d/week) for 1, 2, 3, or 4 consecutive weeks. Permanent hearing loss was observed using electrophysiological examinations 6 weeks after the end of each exposure period. An exposure duration of 1 week was enough to obtain the maximal hearing deficit without any further increase by prolongation of exposure. This was confirmed by histopathology of the cochlea carried out 6 weeks post-exposure: hair cell loss was virtually the same after an exposure duration of 1 or 3 weeks.
The conclusion that maximal hearing impairment is already reached after a few weeks of exposure can also be indirectly inferred by comparison of the results of Albee et al. (1992) and Mäkitie et al. (2003). Albee et al. (1992) exposed male Fisher-344 rats (6h/d, 5d/week) to 0, 50, 200 and 800 ppm styrene over 13 weeks. Effects on the auditory system were determined by electrophysiology and histopathology of the cochlea a few days after the end of exposure. Clear effects were noted at an exposure of 800 ppm and the NOAEC was 200 ppm. This compares well to the NOAEC of 300 ppm determined in male Wistar rats by Mäkitie et al. (2003) after an exposure to 0, 100, 300 and 600 ppm (12h/d, 5d/week) over only 4 weeks.
The conclusion that solvent induced ototoxicity already appears after a relatively short exposure duration and that continued exposure does not enhance the intensity of the response or reduces the NOAEC is also supported by studies with toluene. In a review Johnson and Nylen (1995) described for toluene that a time-integrated concentration between 12000 and 16000 ppm x h/d over 3 days was sufficient to cause loss of auditory sensitivity, while 6000 ppm x h/d over 18 month failed to produce ototoxic effects. Pryor et al. (1984) showed that a 2 week exposure to 1000 ppm toluene (14h/d, 7d/week) produced ototoxicity in Fischer rats while no effects were seen for a similar exposure schedule over 16 weeks to 400 and 700 ppm. Even 3 days at 1500 ppm (14h/d) or at 2000 ppm (8h/d) were sufficient to induce hearing loss. Nylen et al. (1987) demonstrated that the ototoxicity of toluene is governed by intensity of exposure and not by duration or cumulative exposure. Impairment of auditory function as determined electrophysiologically was found in male Sprague Dawley rats after exposure to 1000 ppm (22h/d, 7d/week) over 1 month corresponding to 0.62 million ppm x h. On the other hand, no effects were found after a cumulative exposure of 2.46 million ppm x h achieved by a shorter weekly exposure regime to 1000 ppm (6h/d, 5d/week) over 19 month.
Further direct evidence that maximal hearing impairment will already be reached after a few weeks of exposure is also obtained by the data of Gagnaire et al. (2007) with ethylbenzene and mixed xylenes. Hearing deficits recorded electrophysiologically 4, 8, and 13 weeks after start of exposure (6h/d, 6d/week) and after additional 8 weeks of exposure free observation were all essentially the same: there was no increase of hearing loss over time and the NOAEC did not change.
In summary, an exacerbation of hearing deficits is not to be expected by prolongation of exposure beyond a few weeks to life time.
In the overall assessment it has also be taken into consideration that the NOAEC for ototoxicity as defined by auditory dysfunction is clearly higher than that defined by histopathological effects on the outer hair cells of the cochlea. Numerous investigations have shown that audiometric hearing deficits occur at higher exposure concentrations than (small) losses of hair cells in the outer row 3 of the cochlea. This was described in detail by Gagnaire et al. (2007) for ethylbenzene and two different mixed xylenes. The NOAECs/LOAECs were found at the following concentration: ethylbenzene: auditory threshold - NOAEC 200 ppm; histopathology - LOAEC 200 ppm; mixed xylene (1): auditory threshold - NOAEC 500 ppm; histopathology - LOAEC 250 ppm; mixed xylene (2): auditory threshold - NOAEC 1000 ppm; histopathology - NOAEC 500 ppm.
Similar findings were reported for styrene by Loquet et al. (1999), Lataye et al. (2005),Pouyatos et al.(2002), orLataye et al. (2001). In this respect the findings ofLataye et al. (2001) are important. After exposure to styrene at 1000 and 1500 ppm there was a clear cell loss in the medium spiral ganglion, but not at 750 ppm corresponding better to the dose response relationship of hearing loss (by electrophysiology) than the outer hair cell loss in the cochlea. Thus, the functional results are in better agreement with the morphological results at the level of the spiral ganglion than with those obtained at the level of the cochlea.
The inconsistency in the dose response relationship of the degenerative process of hair cells along the cochlea on the one hand with electrophysiologically measured changes of the auditory threshold on the other hand can be explained by the anatomical situation. To begin with, the auditory message comes mainly from the inner hair cells which are much less prone to damage by organic solvents than the outer hair cells, especially those in row 3. Secondly, the functional results of hearing loss are in better agreement with histopathological changes in the spiral ganglion rather than with those at the level of the cochlea. In this respectLataye et al. (2001) point to an investigation of Prosen et al. (1990) who showed that a massive loss of outer hair cells (up to 50% in the 30% apical region of the cochlea) did not cause hearing loss at any frequency. They conclude that the functional role played by the apical outer hair cells appears to be secondary in the rat. Furthermore, after oral exposure of rats to styreneChen et al. (2008) found that a loss of outer hair cells of <33% did not result in a significant shift of hearing threshold.
Thus, taking very small losses of outer hair cells in row 3 as the decisive endpoint is a very conservative approach for risk assessment based upon hearing impairment.
The mechanism was clarified for styrene by Campo et al.(1999), Loquet et al. (1999), and Campo et al. (2001): Styrene, transported by blood coming from the stria vascularis or the spiral prominence, diffuses through the outer sulcus to reach the lipid-rich Hensen’s cells. These cells are in close connection with the Deiters cells that are directly located under the outer hair cells. Thus, the target cells are reached by diffusion of styrene. This explains why hair cells are lost in a sequence from the outer row 3 to row 1 as diffusion continuous and why the inner hair cell are the least sensitive. Specifically, the involvement of Hensen’s and Deiters cells was supported by histopathology of semi-thick sections of the cochlea (Campo et al., 2001) and Chen et al. (2007) demonstrated that Deiters cells are an especially vulnerable target of styrene. The latter authors further reported that the styrene concentrations in the cochlea varied along with the basilar membrane with the lowest level in the basal turn being consistent with the lowest styrene induced threshold shift and hair cell loss in this region.
The mode of action for hearing loss induced by styrene is well-defined and the weight of evidence indicates that it also applies to other aromatic solvents. As shown above, ototoxicity is governed by direct impact of the (unmetabolised) parent compound on the hair cells of the cochlea. Passage to these target cells occurs via diffusion of the solvent from blood through Hensen’s and Deiters cells. Taking into account the identical target cells (outer hair cells, Hensen’s cells, Deiters cells) and the identical structure of the target organ (cochlea) and even its blood supply, the toxicodynamics for ototoxicity caused by aromatic solvents are very similar between species. This also applies to the comparison between humans and rats if, as a conservative assumption, humans are considered to be of comparable sensitivity as rats.
As regards classification and labeling: Irreversible damage of auditory function and of sensory cells of the cochlea is a serious health concern. Minimal effects on the outer hair cells (row 3) of the cochlea were still observed at 500 ppm (2.2 mg/l) in sedentary rats and at 300 ppm in active rats. Thus, the classification limit for STOT RE (H372) is formally not attained. Nevertheless, such a classification is justified taking into account that the experimental ototoxicity of styrene is comparable to that of ethylbenzene and less than that of toluene and that both of these chemicals have been proposed or assigned STOT RE. This proposal also considers observations on hearing effects in humans at the workplace and thus, leading to a STOT RE Cat. 1.
STUDIES IN HUMANS
Summary of mortality studies (non-malignant disease)
In the few studies that have reported on patterns of mortality from non-malignant disease in occupational groups exposed to styrene the authors of these studies have signalled findings that have been proposed to be worthy of further exploration (Kolstad et al., 1995; Welp et al., 1996a; 1996b; Mataniski and Tao, 2003; Ruder et al., 2004). However, a critical appraisal of the studies, taken together, suggests that they present no convincing evidence that styrene exposure has enhanced the incidence of mortality from any particular disease (Wong and Trent 1999; Delzell et al., 2005). Delzell et al. (2005) is the publication of the reference given in the UK Risk Assessment Report (Delzell, 2004; unpublished). This study was specifically carried out to investigate the association reported by Matanoski and Toa (2003) between ischemic heart disease and exposure of workers in two synthetic rubber plants. The findings of Delzell et al. (2005) did not indicate that exposure to styrene causes fatal ischemic heart disease.
Summary of worker health surveys
In the available worker health survey studies, consistent evidence of an increase in the self-reporting of symptoms of eye and nasal irritation (Rosen et al., 1978; Härkönen, 1977) and CNS disturbance (drowsiness, headache, light-headedness) (Lorimer et al., 1976; 1978; Lilis et al., 1978; Härkönen, 1977) comes through. Unfortunately, the quality of the exposure data means that it is not possible to relate these effects to reliable levels of styrene exposure, particularly as it is possible that these effects are related more to short-term peaks in exposure, rather than to workshift averages. No reliable evidence for any other effects of styrene is indicated by these studies.
Summary of studies focussed on haematological, immunological, hormonal or renal endpoints
From the studies available there is no convincing evidence of a clear, interpretable and toxicologically significant effect of styrene having occurred in exposed workers, in relation to these biological areas (Stengel et al., 1990; Checkoway and Williams, 1982).
A literature search from 1998 to January 2010 overlapping the date of the UK RAR (June 2008) was conducted. Overall, this did not lead to a modification of the conclusions reached by the UK RAR.
Jahnova et al. (2002) studied 29 styrene exposed workers in comparison to 19 control persons. The participation rate was low (69% for exposed and 54% for controls) and exposure data is only available for the day of investigation (mean approximately 30 ppm). There was no difference in hematological parameters between exposed and control workers apart form a decreased percentage and absolute number of monocytes but still being in the normal range for the exposure group. Analysis by flow cytometry revealed elevated levels of most of the assessed surface adhesion molecules of lymphocytes, monocytes, and granulocytes while the level of the soluble adhesion molecule ICAM-1 was decreased. The authors concluded that exposure to styrene appears to increase activation of the immune system and alters leukocyte adherence. The toxicological meaning of this finding remains unclear while no adverse effect was observed for hematological parameters.
Kuricova et al., (2005) compared immunological parameters in styrene exposed workers (mean exposure level 22 ppm) to those in control workers. By hematology a significantly higher number of monocytes and a decreased number of lymphocytes was found in workers although the numbers were still within the normal range. Humoral immunity analysis showed an increase in the C4 component of complement while other parameters did not differ. The proliferative activity of lymphocytes upon stimulation with Concanavalin A was decreased. Some associations between immune parameters and genetic polymorphism were observed. The authors note the limited size of the cohort so that more detailed studies are needed. Overall, the toxicological meaning of the isolated findings related to the immune system is unclear.
Thyroid function and exposure to styrene was investigated by Santini et al. (2008) by examination of 38 exposed workers manufacturing fiberglass reinforced boats in comparison to 123 control male workers. The average exposure duration was 16.1 +/-7.3 years and the exposure level was said to have been constantly below the ACHIG TLV of 1986 for the last 20 years. The following parameters were determined: thyrotropin (basal level and after stimulation with thyrotropin-releasing hormone), free thyroxine (T4), free triiodothyronine (T3), anti-thyroglobulin, thyroid peroxidase antibody, and calcitonin. Thyroid ultrasound examination was also performed. The exposed workers showed a positive correlation between duration of exposure and thyroid volume, but there was no difference in comparison to the control group. Furthermore, in the exposed workers a positive correlation was found between the urinary concentration of the styrene metabolites (mandelic and phenylglyoxylic acid) and T4 as well as the T4/T3 ratio. The authors conclude that styrene could interfere with the peripheral metabolism of thyroid hormones by inhibiting T4 to T3 conversion, but these observations can only be regarded as preliminary and need to be confirmed by lager studies.
Summary of mortality studies related to the central nervous system
Mortality related to the central nervous system was investigated in three large cohort studies. Two epidemiology investigations in European workers in the reinforced plastics industries reported an increased mortality rate ratio for degenerative disorders of the nervous system (Kolstad et al., 1995) for several exposure metrices (Welp et al., 1996c). The observations are difficult to assess, especially due to crude estimates of exposure and the lack of information on important possible confounders and the authors themselves admit that the findings must be interpreted with caution. No relationship was observed between exposure of workers in reinforced plastics plants and mortality from non-malignant diseases of the nervous system by Wong and Trent (1999).
Summary of electroencephalographic studies
Several studies have been conducted on EEG patterns in styrene-exposed workers (Seppäläinen and Härkönen, 1976; Härkönen et al., 1978a; Matikainen et al., 1993; Rosen et al., 1978). Overall, the collective findings do not provide robust evidence for the absence or presence of styrene-induced EEG changes in exposed workers. Furthermore, although the approach is valuable in that it provides measures of nervous system function that are independent of the level of collaboration of the subject, no clear criteria for interpretation of the health significance of any EEG changes that might have occurred are available. If styrene was the causal agent of EEG changes in workers covered by these studies, the most likely interpretation is that the effects were due to the general CNS depressant action of styrene.
Summary of nerve conduction studies
The available nerve conduction studies have produced inconsistent results for different groups of workers exposed to similar levels of styrene. Some studies have indicated a correlation between styrene exposure and small (<10%) decreases in nerve conduction velocity, compared with unexposed controls (Lilis et al., 1978; Cherry and Gautrin, 1990; Stetkarova et al., 1993). Others have shown similar nerve conduction velocities in styrene-exposed and unexposed workers (Seppäläinen and Härkönen, 1976; Triebig et al., 1985). Overall, it is not clear if styrene exposure can produce a small decrease in nerve conduction velocity; furthermore, if it can, the underlying basis for the effect could not be deduced from the available information. Also, the clinical significance of this effect is questionable, as all subjects appeared to be healthy workers.
Summary of otoneurological and audiometry studies
A few studies are available which were designed to investigate any effect of styrene on hearing function (Morata et al., 2002; Sliwinska-Kowalska et al., 2003). When confounding factors such as age and noise exposure were taken into account, no relationship between styrene exposure and hearing loss was found (Sass-Kortsak et al., 1995). There is limited evidence that styrene exposure may have caused minor effects on vestibular reflexes in some workers (Möller et al., 1990; Calabrese et al., 1996). However, the quality of the exposure data is such that it is not possible to relate these effects to reliable levels of styrene exposure. Therefore, although these human data cannot be used for risk characterisation purposes, nevertheless they indicate that the observations of ototoxicity in animals may be relevant to humans.
A literature search from 1998 to January 2010 overlapping the date of the UK RAR (June 2008) was conducted. The most important findings are summarized below.
Several authors have reviewed the information on the relationship of styrene exposure and hearing deficits in humans coming to slightly different conclusions regarding the strength of evidence. Morata and Campo (2002) conclude that “styrene must be considered an ototoxic chemical agent” and “that exposure to styrene, or to styrene associated to noise, may dramatically impact occupational hearing conservation practices and legislation”. According to Lawton et al. (2005) “the results are equivocal” and “hearing deficits due to occupational exposure at low concentrations have not been demonstrated by scientifically reliable argument”. While Johnson (2007) concludes that “human studies provide evidence for the ototoxicity of styrene”, Hoet and Lison (2008) differentiate between animal and human data: “While both solvents appear clearly ototoxic in rats, human data are less straightforward and the existing evidence does not allow characterization of the dose-response/effect relationships”.
Sliwinska-Kowalska et al., (2005) studied hearing deficits in 290 workers exposed predominantly to styrene by pure-tone audiometry. Only crude exposure data are reported. The odds ratio of hearing loss in the exposed workers was 3.9 (95% CI 2.4-6.2) and the odds of developing hearing deficits increased substantially in the case of combined exposure to noise.
Johnson et al., (2006) report hearing deficits in styrene exposed workers recruited from the fiberglass products industries. Current styrene exposures were 0.2-96 (mean 16) mg/m³ and the lifetime exposure index was 1303 (mg x years/m³), but details are missing on exposure levels in former years. As regards exposure assessment the authors also refer to Morata et al. (2002). Different methods used to assess hearing ability like pure tone audiometry, distortion product otoacoustic emissions (DPOAE), interrupted speech and speech recognition indicated to impaired hearing ability.. Interrupted speech and speech recognition were found to be especially sensitive. These findings indicate that styrene affects hearing both in the peripheral and central part of the auditory system leading not only to cochlear but also to retrocochlear, central dysfunctions.
Similarly, Zamyslowska-Sznytke et al. (2009) suggest an association between styrene exposure and central auditory dysfunction characterized by a temporal processing disorder. They studied 59 workers from factories using fiberglass in comparison to 50 non exposed subjects. Individual styrene exposure levels covering more than 20 years were used to calculate the work life averaged mean exposure being 38 +/-24 mg/m³. Besides pure-tone audiometry three temporal processing tests (gaps-in-noise, frequency pattern, duration pattern) were carried out. Styrene exposed subjects presented worse audiometric hearing thresholds for most of the frequencies tested. ANCOVA analysis including hearing level and age as covariates showed that the exposed had significantly poorer performances in two of the three temporal processing tests than the non-exposed persons.
The most comprehensive study on styrene induced hearing loss with detailed past and present exposure data has been reported by Triebig et al. (2009). No hearing deficits were found for exposures of 12.5 ppm and 50 ppm at the time of investigation going back to about 10 years. On the other hand, there was an indication for styrene induced hearing losses in a subgroup of “high-long” exposed workers assumed to be exposed at 25-33 ppm over about 15 years (range up to 26 years). Higher exposures of 80-100 ppm existed for a time more than 10 years before this study was initiated. Taking into account the irreversibility of styrene induced hearing deficit, these effects are considered to stem from the former high exposures.
Toppila et al. (2006) investigated postural stability as an indication for vestibulotoxicity in reinforced plastic boat plant workers. At the time of investigation the mean exposure of 109 laminators was 116 mg/m³ and of 143 nonlaminators 17 mg/m³. No information of former exposure levels is given. On a static (not moving) platform the postural stability of laminators was not impaired in comparison to nonlaminators. But there was a significant difference between both groups when the platform was tilting. This effect was noted when there was no visual input to the subjects as well as when virtual reality images were presented. The effect was age related for the laminators indicating to an adverse effect of styrene.
In conclusion, the potential ototoxicity, and possibly also vestibulotoxicity, of styrene has been confirmed in recent investigations. Recently some indications were obtained that not only cochlea function but also central processing might be affected by styrene exposure. As animal experiments have demonstrated an irreversible effect of styrene on the outer hair cells of the cochlea, a quantitative exposure related evaluation has to take into account not only present but also potentially higher former exposures. Only the study of Triebig et al. (2009) provides sufficient information in this respect. This study indicates that exposure levels at the time of investigation of 12.5 up to 50 ppm did not induce ototoxicity in workers. On the other hand there was an indication of hearing impairment in workers exposed to higher concentrations years ago.
A new literature search up to March 2013 was carried out. The following papers were identified but did not change the former conclusions.
Zamyslowska-Szmytke, Sliwinska-Kowalsky (2011) investigated vestibular/balance effects in 74 workers exposed to styrene and dichloromethane in comparison to 49 controls byvideonystagmography and static posturography. For exposure assessment the cumulated exposure index (in ppm x years) was calculated based on exposure data over the last 20 years and the work-life exposure was obtained by dividing the cumulated exposure index by the total years of exposure. The mean work-life exposures were 36.8 mg/m³ (range 4.2-108.1) for styrene and 41.7 mg/m³ (range 0-101.1) for dichloromethane. 64% of exposed workers (compared to 28% of controls) revealed abnormal results in videonystagmography with several of the individual parameters investigated being statistically significantly different. Significant differences were also found by static posturography. No relationship between exposure and vestibular and balance test results was observed. The authors conclude that these findings indicate deficits in the central part of vestibular system but bilateral (peripheral) vestibular hypofunction might also be a consequence of solvent exposure. In the discussion the authors point to the high exposure concentrations ofdichloromethane compared to its occupational exposure limits and its vestibulotoxicity observed in an animal experiment. In summary, this study points to a possible vestibular toxicity of styrene, but no clear differentiation is possible whether the adverse effects observed were due to styrene or dichloromethane.
Morata et al. (2011) studied hearing loss in 862 workers exposed to styrene (0-309 mg/m³), alone or with noise (70-84 dB(A)) in comparison to 400 workers exposed to noise only and to 358 controls. Exposures were assessed by passive sampling for a full shift for styrene and by personal noise measurements. The audiometric thresholds of styrene exposed workers as determined by pure-tone audiometry were significantly poorer than those in published studies and styrene exposure (besides age and gender) met the significance level criterion by multiple logistic regression for hearing loss. Exposure to noise significantly interacted with styrene exposure while noise alone at 80-84 dB(A) had no significant effect on hearing. An important weakness of the study, as noted by the authors themselves, is that exposure histories are lacking. Thus, high exposures in the past may have been an important factor for the findings reported here. In summary, in this study hearing deficits were observed in workers exposed to styrene with an interaction with exposure to noise. An important weakness is missing historical exposure data that would allow discriminating effects caused by former high exposures from those caused by present exposures.
A literature update until Oct. 01, 2015, yielded the following new information:
Sisto et al. (2013) studied effects on hearing ability in 15 workers simultaneously exposed to noise and styrene in a fiberglass manufacturing facility in comparison to a control group of 13 unexposed subjects. No information is available on selection criteria of the enrolled subjects or for the participation rate and no data are given on former exposure conditions, especially as the organization of work tasks had been changed some time ago. The exposed workers were subdivided into one group with high noise and low styrene exposure and a group with low noise and higher styrene (close to 20 ppm) exposure. Otoacoustic emissions showed a statistically significant negative correlation with the urinary MA+PGA concentrations. The authors admitted that because of the small group size the results need to be confirmed by studying larger groups.
Chiaramello et al. (2014) reported a preliminary analysis for the application of otoacoustic emissions to a smll group of 7 workers exposed to styrene (in the range of 20 ppm) in a fiberglass manufacturing facility compared to 27 normal hearing young adults not exposed to styrene or noise. The amplitude of quadratic frequency couplings in Transient Otoacoustic Emissions showed differences between exposed workers and controls. No details are available for the selection criteria for the participants or for matching of exposed and control subjects.
Tognola et al. (2015) studied similar or possibly the same groups of subjects as Sisto et al. (2013) and Chiaramello et al. (2014). Personal noise and styrene measurements were carried out during the workshift and the workers were assigned to to a subgroup (N=9) with low noise (<85 dB) and higher styrene exposure (in the order of 85 mg/m³) and a subgroup (N=6) with high noise (>85 dB) and low styrene (<<10 mg/m³) exposure. Two control groups (N=9; N=6) were perfectly matched by age and sex to these exposure groups. Two advanced analysis techniques were compared to detect subclinical changes in otoacoustic emissions. The authors concluded that low levels of styrene may induce modifications in otoacoustic emission patterns but not a change in latency. This paper describes a very sophisticated analysis of otoacoustic emisssions and regarding the groups investgated the same critiques apply as for the studies of Sisto et al. (2013) and Chiaramello et al. (2014).
Fischer at al. (2014) report a case of transient vestibular dysfunction of a 76-year old man exposed to low levels of styrene in his bedroom (1.35 mg/m³) after renovation works. Eight months after cessation of exposure the symptoms had significantly improved. The authors suppose that this low exposure over a relatively short time may have led to the vestibular dysfunction. No conclusion can be drawn from this singular case exposed to these very low concentrations.
Vyskocil et al. (2012) developed a database for industrial chemicals possibly interacting with the auditory system taking into account realistic exposure levels, i.e. up to short term exposure limits or 5 times the 8 h TWA for studies on humans or 100 times the 8 h TWA for animals studies. On this basis, a weight of evidence analysis indicated by human and animal studies that styrene is possibly ototoxic at concentrations that are relevant to occupational settings.
Summary of studies on olfaction
Dalton et al. (2003) studied odor detection thresholds and odor identification ability of 52 styrene exposed workers from the GRP industry in comparison to 52 unexposed workers. The current mean exposure was 26 ppm, range 10-60 ppm. No impairment of olfactory performance was noted for the styrene exposed workers apart from a statistically significant increase of odor detection threshold for styrene itself. According to the authors, this effect is most likely indicative of an exposure induced adaptation. These results are confirmed by a further similar investigation in a reinforced plastic boat manufacturing facility in 30 workers Dalton et al., 2007). The exposures during the last two years before the time of investigation were 15-25 ppm with historical exposures up to 85 ppm.
Summary of studies on colour vision
The most well reported and conducted studies are those by Triebig et al. (2001) in German workers, and the 3 studies each in Italian (Gobba et al., 1991; Gobba and Cavalleri, 1993; 2000) and Japanese workers (Eguchi et al., 1995; Kishi et al., 2001; Gong et al., 2002). These studies provide some evidence to support the view that styrene does cause changes in colour discrimination relative to age-matched controls. However, it should be noted that, as a single colour vision test rather than a testing battery approach was used, these findings are not sufficiently robust to reliably characterise the scale and the nature of the effect. Generally, the effect was on the tritan (blue-yellow) type, although some workers also had evidence of red-green colour vision deficiency. However, there is a lack of information on whether the effect is related to short-term or repeated long-term exposures to styrene. There is also a lack of information on the magnitude of the effect in workers exposed to styrene concentrations > 100 ppm (8h TWA), although performance in colour discrimination tests at these high exposures is likely to be subject to confounding because of transient CNS depression and eye irritation. Having concluded that styrene exposure does cause changes in colour discrimination, key issues that need to be resolved are whether a threshold exposure level can be identified and whether the effect is reversible or persistent.
Regarding threshold exposures, the results of the Triebig et al. (2001) study suggest that no deficiencies in colour discrimination would be expected in those exposed to less than about 20 ppm styrene (8 h TWA). At all time points in this 2-phase study, effects on colour discrimination were described by the study authors as “mild”, and the styrene-exposed workers were said to have no conscious awareness of any impairment. Of the Italian studies, analysis indicated that workers exposed to >50 ppm styrene had significantly poorer colour discrimination that those exposed to <50 ppm (Gobba et al., 1991). The latter group had a median CCI value of 1.09, similar to control values. In one of the Japanese studies (Gong et al, 2002) workers were placed into a high or low exposure group according to whether urinary MA+PGA levels were above or below 240 mg/g creatinine, corresponding to an airborne styrene concentration of about 12 ppm (8 h TWA). The results suggested that colour discrimination would only be impaired in those exposed to above 12 ppm, but a precise threshold could not be determined. Taking all these results together, it appears that changes in colour discrimination would not be expected with 8 h TWA exposures below 20 ppm.
In relation to reversibility, the Triebig et al. (2001) study provides the most rigorous and systematic investigation of this issue, in that in the same workforce colour discrimination was assessed pre-shift at the start of a working week, post-shift on a Thursday, and after a 4-week exposure free period, with a repetition of these same test conditions one year later after a reduction in exposure levels at the plant. In both phases of the study, the results of post-shift testing showed a markedly higher proportion of subjects making test errors in the styrene-exposed group compared to controls. Colour discrimination in exposed workers tended to be worse post-shift on a Thursday than at the start of the working week. After a 4-week exposure-free period, there were no differences between styrene-exposed and control workers suggesting that the impairment of colour discrimination was fully reversible. The reproducibility of this pattern of findings after 12 months strengthens the evidence from this study. In contrast, in one of the three Italian studies, colour discrimination was tested in a sub-group of workers before and immediately after a one-month holiday (Gobba and Cavalleri, 2002). There was no improvement in mean CCI in this sub-group after the holiday, suggesting a persistence of the effects of styrene. However, the control workers were not tested after a one-month holiday to confirm the stability of the control test scores, and so this study was not as rigorous as the Triebig et al. (2001) study. The Japanese studies are of little help in resolving the issue of reversibility, in that in each of these studies, colour discrimination testing was only conducted pre-shift, specifically to avoid the potential for any acute effects of styrene. In summary the body of data on the issue of reversibility of the effect of styrene is not extensive and is inconclusive. The results from the most rigorous study in which this was investigated point to a reversibility of effect (Triebig et al., 2001), whereas results from a somewhat less well-conducted study suggest a persistence of effect (Gobba and Cavalleri., 2000). Overall, no firm conclusions can be drawn regarding reversibility.
In relation to mechanisms, one possible mechanism by which solvents might reversibly affect colour discrimination is by a transient effect on electrical membrane potentials in the retina. A study in anaesthetised cynomolgus monkeys used an electroretinogram to study the effects of solvents on the electrophysiology of the retina (Skoog & Nilsson, 1981). Within 20 minutes of intravenous administration of styrene and toluene at doses of 3 to 15 mg/kg and 0.3 to 60 mg/kg respectively, there was a hyperpolarisation of the electrical potentials of the retinal membrane and the eye. It has been calculated that these intravenous concentrations will be produced by 20 minutes exposure to airborne concentrations of approximately 50 ppm styrene and 100 ppm toluene respectively. Although such an effect of solvents on signal transduction at the retinal membrane could be responsible for acute effects on colour discrimination, since in human volunteer studies 30 minutes inhalation exposure to 300-350 ppm toluene was found to have no acute effect on colour discrimination (Muttray et al, 1999), there is doubt on the relevance of these findings in animals to the mechanism of colour discrimination impairment in humans. Another possible mechanism is by an effect on the content of neuramines in the retina. A recent non-standard study (Vettori et al., 2000; see study in animals) in rats exposed by inhalation to 300 ppm styrene (the only concentration tested) for 12 weeks has shown that the content of neuramines (dopamine and DOPAC) and glutathione in the retinal cells of the exposed animals was lower than in controls. However, again, the relevance of these findings in animals to the mechanism of colour discrimination deficiency in humans is unknown, especially if it is considered that rats are dichromatic and humans are trichromatic.
Another issue that needs to be addressed is the health significance of these changes in colour vision observed in styrene-exposed workers. Age-related reference CCI values obtained in healthy individuals with the D-15d test have been reported in the literature; the reference values of Muttray et al (1998a) have been reproduced in Table (b).
Table (b). Distribution of age-related CCI scores with the D-15d test in a population of healthy workers (taken from Muttray et al, 1998a)
Age No of workers | 19-25 (n=57) | 26-35 (n=93) | 36-45 (n=73) | 46-55 (n=56) | 56-62 (n=17) | |||||
Eye | 1st eye | 2nd eye | 1st eye | 2nd eye | 1st eye | 2nd eye | 1st eye | 2nd eye | 1st eye | 2nd eye |
50th percentile | 1.0 | 1.0 | 1.0 | 1.0 | 1.06 | 1.0 | 1.12 | 1.07 | 1.20 | 1.17 |
90th percentile | 1.18 | 1.16 | 1.22 | 1.12 | 1.19 | 1.18 | 1.41 | 1.31 | 1.72 | 1.41 |
95th percentile | 1.22 | 1.25 | 1.29 | 1.21 | 1.25 | 1.22 | 1.55 | 1.34 | 1.72 | 1.61 |
Some investigators have used the 95th percentile of these CCI reference as a criterion for judging “abnormal” colour discrimination. Thus, CCI scores that are markedly above the normal age-related reference values can be judged as abnormal and can give some indication of the severity of effect. Moreover, one way of judging the functional significance of any CCI difference is to relate it to the changes that normally occur as a result of ageing (Sheedy, 1997). For example, a comparison of CCI values reported for the D-15d test in the Triebig et al. (2001) study with the age-related norms in Table (b) indicates that the styrene-exposed workers, who had a mean age of 38, had CCI values in the range expected for individuals aged about 60.
One possible criterion for assessing the health significance of this effect could be whether or not the changes are severe enough to affect a worker’s ability to carry out a particular job, such as colour matching in paint manufacturing or printing. Information on this aspect is available from a recent study (Muttray et al, 1998b). The ability of a group of rotogravure print workers to mix inks correctly was compared with the results of colour discrimination testing assessed in these workers by a battery of tests including an anomaloscope and the D-15d and Ishihara tests under standard illumination. The ability to mix red, yellow and blue inks to a required composition was assessed by the senior staff member who was known to have perfect colour discrimination. The workforce consisted of 38 printers and print preparation staff and had a mean age of 41 years (range 19 to 66). Of these 38 workers 5 were found to have congenital colour vision deficiency, and had difficulties matching colour. One of these workers was already aware of his condition, and in the past had sought help when colour matching. None of the remaining 33 workers without congenital colour vision deficiency had difficulties matching colour. Of these workers 17 had perfect scores with the D-15d test (CCI = 1), 5 made only minor errors and 11 who were found to have abnormally high CCIs, due to tritan errors. For this last group mean CCI values were in the range 1.05 to 1.29. This study suggests that workers making errors with the D-15d test of the magnitude reported among styrene exposed workers may well be able to perform jobs that require good colour discrimination.
Another criterion for assessing the health significance of this effect could be whether or not the worker is consciously aware of it. Information on such a factor can be obtained from one of the studies available. Triebig et al (2001) reported that styrene-exposed workers making errors in colour discrimination tests were not aware of any deficit.
The issue of reversibility will also influence views on the health significance of styrene-induced colour discrimination changes. The most rigorous study (Triebig et al, 2001) suggests that effects on colour discrimination are fully reversible within weeks following cessation of exposure and showed significant improvement after an exposure-free weekend.
Overall, although there are findings suggesting changes in colour discrimination at relatively high levels of exposure to styrene (350 ppm, 8h TWA), it should be noted that the available studies are not sufficiently robust to reliably characterise the scale and nature of the effect. Also, given the very mild nature (the affected individuals were not even aware of any deficit) and the likely reversibility of the effect which appears not to affect performance in jobs that require good colour vision, it is deemed that the slight changes in colour discrimination detected should not be considered as an adverse health outcome of styrene exposure. It can be concluded that, since the effects observed at 50 ppm (216.5 mg/m3; 8h TWA) are not yet adverse, this exposure value can be considered a NOAEC.
A literature search from 1998 to January 2010 overlapping the date of the UK RAR (June 2008) was conducted. Overall, this did not lead to a modification of the conclusions reached by the UK RAR.
In a review regarding the effects of occupational exposure to organic solvent Lomax et al. (2004)[B37] come to a similar conclusion as the UK RAR. The data support a robust conclusion that styrene does impair color discrimination. The limited information on exposure relationships indicates that such an impairment would not be expected at exposures <20 ppm (8h TWA). Data on reversibility are limited and inconclusive, but the most robust study (Triebig et al., 2001) points to reversibility after an exposure free period of 4 weeks. Overall, the effects of styrene on color discrimination are subtle. A review of Good and Nichols (2006) basically came to the same conclusion that styrene levels above 50 ppm may lead to alterations in color vision.
On the other hand, on the basis of a meta-analysis Benignus et al., (2005) concluded that there is an increase of the color confusion index (CCI) after 8 years of exposure to 20 ppm which is roughly equivalent to 1.7 additional years of life. But according to Seeber et al. (2009) this may well be related to the inhomogeneity of measurements of the CCI as found by Paramei et al. (2004).
A meta-analysis by Paramei et al. (2004) on the influence of solvent exposure (styrene, toluene, solvent mixtures) on color vision impairments measured by the Lanthony D-15 test led to the following main conclusions:(i) CCIs obtained from the LanthonyD-1 5d diverge more among the studies than could be expected from within-study sampling error; and (ii) the average effect sizes show a negative trend for the effect of exposure on color vision but this effect is not significant. The effects of solvent exposure on color vision should be seen with some caveats. The Lanthony D-15 test is very sensitive to many potential confounders that may be difficult to control, for example illumination, number of training sessions, language skills, explanation of test procedure, different investigators / instructors. Therefore careful interpretation is necessary, especially if the investigations did not include a concurrent control group or were carried out at different times for comparison purposes.
Nichols and Good (2006) assessed the potential impact of small color discrimination deficiencies as caused by occupational exposure to styrene on quality of life. Their evaluation was based on the fact that only individuals with relatively severe congenital color vision defects report any substantial detrimental effects in everyday life. In contrast, the small color vision deficiencies are generally not realized by affected styrene exposed workers. Therefore, the authors conclude that the effects reported for styrene exposure might show little-to-no impairments in quality of life and functional ability.
There are two new studies not included in the UK RAR or in the reviews mentioned above.
Iregren et al. (2005) studied 108 workers in reinforced plastic plants. Acute exposures are given for the time of investigation (0.3-96 mg/m³) and past exposures were estimated by questionnaire to enable calculation of cumulative exposure (18-4455 mg x years/m³) and life-time weighted average exposure (5-129 mg/m³). Analysis of variance revealed an exposure related effect on color vision. Because exposures were found to be on the average below 90 mg/m³, the authors concluded that even levels below 90 mg/m³ may affect color vision negatively. A major problem of this study is that a control group of unexposed workers was not included.
The strongest and most recent study is that of Seeber et al. (2009[B38] ). 242 workers of a boat building plant were assigned to groups of low, medium, and high exposures according to urinary excretion of styrene metabolites on the day of investigation. As exposure data were available dating back to 1982, in addition age-matched subgroups of low-short and long-high exposures were defined based on life time weighted mean exposure. Color vision and contrast sensitivity were examined during normal working days and during the company holidays after an exposure free interval. Both long term exposures to 27 ppm (range of standard deviation up to 44 ppm with higher exposure levels in the past) for a period of about 15 years and acute exposure levels of 40 ppm (range of standard deviation up to 54 ppm) were not identified as causing elevated risks for color vision or contrast sensitivity. As regards acute exposures, at the day of investigation at least two hours were spent in the exposure-free examination room. Therefore the real exposure on ”normal” working days were estimated as about 25% higher.
In conclusion, a NOAEC for color discrimination of 50 ppm can be derived from by weight of evidence analysis of studies available for workers in the reinforced plastic industry.
A new literature search up to March 2013 was carried out. The following paper was identified strengthening the conclusion that styrene exposure does not lead to color vision or contrast discrimination deficiencies.
Vyskocil et al. (2012) investigated 104 workers (51 men, 53 women) in the reinforced plastics industry. The subjects were divided into three groups based on their average exposure concentration during the work shift. On average, the “Control group” was exposed to 7 mg/m3, the “Average group” to 137 mg/m3, and the “High group” to 333 mg/m3. The maximum exposure was 520 mg/m³. The workers were also classified according to their exposure to peaks: “Control group” (the same as previously), “Group without peaks” (not exposed to large variations in the concentration in the air), and “Group with peaks” (exposed to large variations). The subjects carried out the following tests: Self-evaluation questionnaire (mood and symptoms), neuropsychological tests (simple reaction time, response time to stimuli, symbol digit matching, digit span memory, joystick tracking) and sensory tests (Lanthony desaturated colour D-15, Vistech VCTS 6000 for contrast discrimination).Important exposure peaks were observed. Workers exposed to styrene at average concentrations of 137mg/m3reported irritation symptoms more frequently than the “Control group” or the “Average group.” The other tests (including neurobehavioral and visual tests for color and contrast discrimination) and symptoms showed no relationship with styrene exposure. Also, there was no difference between the “Group with peaks” and the “Control group” and the “Group without peaks.” In summary, this study showed that occupational exposure to styrene is associated with symptoms of sensory irritation. No effects were found in neuropsychological tests or in visual tests for color and contrast discrimination.
Summary of biochemical studies related to nervous system functioning
This is an area of research which is at a very early stage in its development and is therefore not using standard methods. There is no recognised framework available for the interpretation of the results. There are indications of lower levels of serum dopamine ß-hydroxylase (DBH) activity (Bergamaschi et al., 1996; 1997) and higher levels of serum prolactin (PRL) (Bergamaschi et al., 1996; 1997; Luderer et al., 2004) with styrene exposure (median levels of 9 and 25 ppm). There are also indications that increased PRL secretion is an acute rather than a chronic effect of styrene exposure (Luderer et al., 2004).
Gelbke et al. (2015) evaluated potential endocrine activities of styrene. In vitro and in vivo screening studies, as well as non-guideline and guideline investigations in experimental animals indicate that S is not associated with (anti)estrogenic, (anti)androgenic, or thyroid-modulating activity or with an endocrine activity that may be relevant for the environment. Studies in exposed workers have suggested elevated PRL levels that have been further examined in a series of human and animal investigations. While there is only one definitively known physiological function of PRL, namely stimulation of milk production, many normal stress situations may lead to elevations without any chemical exposure. Animal studies on various aspects of dopamine (DA), the PRL-regulating neurotransmitter, in the central nervous system did not give mechanistic explanations on how Styrene may affect PRL levels. Overall, a neuroendocrine disruption of PRL regulation cannot be deduced from a large experimental database. The effects in workers could not consistently be reproduced in experimental animals and the findings in humans represented acute reversible effects clearly
below clinical and pathological levels. Therefore, unspecific acute workplace-related stress is proposed as an alternative mode of action for elevated PRL levels in workers.
A literature search from 1998 to January 2010 overlapping the date of the UK RAR (June 2008) was conducted. No further studies relating to this endpoint were identified.
Summary of results from neurobehavioural tests
Volunteer studies indicate that a single exposure to styrene levels of 200 ppm for 90 minutes or 350 ppm for 30 minutes can produce some slight reduction in performance in reaction time tests (a slower response time) and other similar parameters. These effects are related to the general CNS depressant potential of styrene (see single exposure section).
Numerous workplace studies using neurobehavioural testing are available. It is noted that a range of significant confounding factors has not always been addressed. The results obtained have been variable, with some studies reporting effects and others no effects for workers within similar exposure ranges. Performance was adversely affected in several studies in only a small proportion (1-3 of the 6-20) of the tests administered. Also, different types of neurobehavioural test (representing various functional domains of the CNS) were apparently sensitive to styrene exposure in different studies. This lack of a clearly consistent effect on particular functional domains indicates that there is only weak evidence for a causal relationship. In some studies, there is a possibility that effects similar to those detected in volunteer studies i.e. slightly slower response times were observed and these might be related to brief peaks of styrene exposure of hundreds of ppm that had occurred during the previous shift. Overall, there is insufficient information on which to base any conclusions about the potential long-term effects of styrene exposure on neurobehavioural parameters. There are only four studies in which the potential for acute effects can be entirely excluded. One of these studies suggests a possible deficit in a single neurobehavioural parameter (manual dexterity) (Flodin et al., 1989); another provides no evidence for a functional deficit in reaction time (Cherry and Gautrin, 1999); and the two most recent studies (Viaene et al., 1998 and 2001) failed to demonstrate that the effects seen were due to styrene exposure because of inadequate adjustment/matching for educational level. In view of this, it is not possible to discern a clear dose-response relationship for any of the effects observed in neurobehavioural tests in workers.
Furthermore, at present there is not a clearly established, widely accepted interpretational framework into which these results can be fitted. Where apparent deficits in neurobehavioural test performance have been measured, the underlying toxicological processes involved, the consequences for the health and safety of the individual, and the effect of styrene in comparison with that of other experiences and phenomena regularly encountered in everyday life, have not been established.
Taking all of these points into consideration, the rapporteur proposes that the crucial issue in relation to the impact of styrene on the nervous system is the need to avoid acute CNS depressant effects and associated symptomatology.
A literature search from 1998 to January 2010 overlapping the date of the UK RAR (June 2008) was conducted. The most important findings are summarized below.
Bagirzadeh et al. (2006) reviewed the literature 1990-2003 on neuropsychological effects of styrene exposure. Without differentiation between irreversible and acute effects that may normalize after an exposure free period they concluded that “styrene has the potential to cause unpleasant symptoms, and probably changes in test performance, at levels of exposure close to 50 ppm”.
Sato et al. (2009) examined vibration perception threshold (VPT) in 67 workers exposed to styrene in a fiberglass reinforced plastic boat plant in comparison to 151 unexposed subjects. Exposure data (end of shift urinary metabolites of styrene) were available for the past 8 years and the time of investigation. The VPT was significantly higher for the exposed as compared to the non-exposed group. Multiple regression analysis revealed that past maximum exposure level and age were significant factors for explaining the variation of VPT. By division of the exposed workers into high- and low-level groups for the maximum past exposure levels by a cutoff according to 50 ppm, the authors concluded that effects of styrene on VPT are likely to persist if the maximum exposure in the past exceeded 50 ppm.
The strongest and most recent study is that of Seeber et al. (2009[B39] a). 213 workers of a boat building plant were assigned to groups of low, medium, and high exposures according to urinary excretion of styrene metabolites on the day of investigation. As exposure data were available dating back to 1982, in addition age-matched subgroups of low-short and long-high exposures were defined based on life time weighted mean exposure. Besides evaluation of symptoms by questionnaire the following tests were carried out during normal working days and during the company holidays after an exposure free interval: visual retention, symbol digit substitution and digit span for cognitive functions as well as choice reaction, aiming, peg board, tapping, and steadiness for psychomotor functions. Symptoms were not related to exposure. The tests for cognitive functions generally revealed no exposure related associations. Only the linear regressions of test results showed a significant correlation with parameters of chronic exposure which was still evident as a tendency in the exposure free period. Most tests for psychomotor functions also revealed no relationship with exposure. Only the peg board test results showed significant correlations with chronic exposure which disappeared during holidays. The (physical) activity as a laminator – considered in addition to exposure parameters – was significant as a factor to explain the variability of psychomotor variables. In conclusion, both long term exposures to 27 ppm (+/-25 ppm) for a period of about 15 (+/-7) years and acute exposure levels of 40 ppm (+/-14 ppm) were not associated with elevated risks of impaired cognitive and psychomotor functions or increased symptom levels. There were two possible exceptions: performance in the test and a finger dexterity test were associated with parameters of long-term exposure but not with current exposure.
In conclusion, for long-term exposure a NOAEC for neurobehavioral effects and exposure related symptoms in the range of 50 ppm can be derived from by weight of evidence analysis of studies available for workers in the reinforced plastic industry.
A new literature search up to May 2022 was carried out. The following papers were identified strengthening the conclusion that styrene exposure does not lead to neurobehavioral effects.
Vyskocil et al. (2012) investigated 104 workers (51 men, 53 women) in the reinforced plastics industry. The subjects were divided into three groups based on their average exposure concentration during the work shift. On average, the “Control group” was exposed to 7 mg/m3, the “Average group” to 137 mg/m3, and the “High group” to 333 mg/m3. The maximum exposure was 520 mg/m³. The workers were also classified according to their exposure to peaks: “Control group” (the same as previously), “Group without peaks” (not exposed to large variations in the concentration in the air), and “Group with peaks” (exposed to large variations). The subjects carried out the following tests: Self-evaluation questionnaire (mood and symptoms), neuropsychological tests (simple reaction time, response time to stimuli, symbol digit matching, digit span memory, joystick tracking) and sensory tests (Lanthony desaturated colour D-15, Vistech VCTS 6000 for contrast discrimination). Important exposure peaks were observed. Workers exposed to styrene at average concentrations of 137mg/m3reported irritation symptoms more frequently than the “Control group” or the “Average group.” The other tests (including neurobehavioral and visual tests for color and contrast discrimination) and symptoms showed no relationship with styrene exposure. Also, there was no difference between the “Group with peaks” and the “Control group” and the “Group without peaks.” In summary, this study showed that occupational exposure to styrene is associated with symptoms of sensory irritation. No effects were found in neuropsychological tests or in visual tests for color and contrast discrimination.
Iversen et al. (2020) investigated the long-term risk of encephalopathy and unspecified dementia following styrene exposure. We followed 72,465 workers in the reinforced plastics industry in Denmark (1977–2011) and identified incident cases of encephalopathy (n = 228) and unspecified dementia (n = 565) in national registers. Individual styrene exposure levels were modeled from information on occupation, measurements of work place styrene levels, product, process, and years of employment. Adjusted analyses were performed using a discrete survival function. A positive trend for encephalopathy (P < 0.01) and a negative trend for unspecified dementia (P = 0.03) were seen with cumulative styrene exposure accrued during the recent period of up to 15 years. For unspecified dementia and the combination of unspecified dementia and encephalopathy, a positive trend was indicated when applying a 30-year exposure lag (P = 0.13 and P = 0.07). The risk patterns seen following recent
exposure probably reflect diagnostic criteria for encephalopathy requiring recent industrial solvent exposure and referral bias rather than association with styrene exposure, while the increasing risk observed for unspecified dementia and the combination of encephalopathy and unspecified dementia following distant exposure indicates an increased risk of dementia following styrene exposure with a long latency period. However, since exposures were only modelled and possible genetic or lifestyle factors, electromagnetic fields, or other occupational factors could not be taken into account, the conclusions are of questionable relevance.
Sati et al. (2011) studied pulmonary function of 34 styrene exposed male workers from a plastic factory (no further details) employed between 1-10 years in comparison to 30 age and sex matched controls (no further details). Lung function tests showed a statistically significant reduction in most of the parameters for lung volumes, capacities and flow rates of exposed workers in comparison to the controls. It is to be noted that the matching criteria for the control subjects are very sparse. In addition, although smoking was mentioned to have been analyzed as potential confounder in the statistical analysis, no indication is given about verification of smoking status. Importantly, no exposure data are available and the small group size is noted.
Helal and Elshafi (2012) compared 40 male workers (age 18-33 years) from an Egyptian plastic factory (no further details given) with 40 unexposed controls from the same employer matched for age, sex, socioeconomic status, and smoking habits (no indication how smoking status was verified). All subjects participated voluntarily but no information is given for the selection criteria from the total workforce or for the participation rate. The styrene exposed workers worked for 12 h/d with 1 day off without any protection equipment. Urine was taken during workshift but no further specification is available and time of blood sampling is unknown. Styrene blood levels were 1117±64,52µg/l and urinary mandelic acid 246±21,60µmol/l, but these data are difficult to interpret because of uncertainties about sampling times. There was a statistically significant impairment of lung function parameters when comparing the exposed vs. the reference population and a significant correlation with exposure duration. In addition, creatinine in blood andß2-microglobulin in urine were increased as indication for effects on the kidney. The long working shift for the workers is noted as well as the unclear participation rate.
Obliterative bronchiolitis is a rare and potentially fatal disease. Cullinan et al (2013) identified 6 cases all working fibreglass styrene resins. Their average age was 34 years and 5 of them had worked with fiberglass panels for 12 months or less. The cases were assembled from clinical experience of 2 of the authors. Four cases were confirmed by histology. The size of the total population under consideration is unknown as well as the exposure concentrations to glassfibres and styrene.
Cytokeratin 18 (CK18) is a serologic marker for liver disease. Cave et al. (2011) found that CK18 was elevated in 39% of 82 workers employed in 2 factories for acrylonitrile/butadiene/styrene polymerization (ABS). The elevated CK18 levels were not explained by alcohol or obesity (except in potentially 4 cases) and the pattern was consistent with toxicant-associated steatohepatitis. TNFα, IL-6, IL-8, monocyte chemotactic protein-1, and plasminogen activator inhibitor-1 were increased in these workers as compared with those with normal CK18 levels. The analysed serum samples were collected between the mid 1970s and late 1980. Cumulative chemical exposure indices were determined by assignment of jobs based on the level of exposure to ABS. No information is available on the potential exposure to styrene. The authors suggest a high prevalence of liver disease in elastomer/polymer workers with elevated proinflammatory cytokines.
Strafella et al. (2013) measured stress-related cytokines on the protein (in serum) and mRNA expression level (in lymphocytes) in 96 styrene exposed workers in comparison to 54 office workers matched for age, gender, and life style. No information is available for the selection criteria of the study population and for the participation rate. Exposure was determined by 8 h air sampling on 3/4 days for 15 workers and by biomonitoring MA+PGA in urine. Clinical hepatic parameters (ALT, ASP,γ-GT, AP) were measured in serum. Median exposure parameters were 50.7 mg/m³for styrene in air and 137.8 mgMA+PGA/g creatinine in urine. The exposed workers were divided into a high and low exposure group (>/< 200 mgMA+PGA/g creatinine). An exposure related upregulation of geneexpression was observed for EGR1, IL-6, and TNFα. On the protein level there was an exposure response relation for TNSF10 and TNFα. All hepatic parameters showed a dose related increase, albeit they never exceeded normal clinical levels. Multiple regression analysis adjusted for various potential confounders confirmed an association between exposure and stress proteins and mRNA levels and the hepatic indicators ALT and ASP. The authors conclude that styrene exposure even below the 8 h TWA induces EGR1 gene expression preceding IL-6 and TNFα that are involved in liver regeneration after hepatotoxic exposure.
Endocrine activity
Gelbke et al. (2015) evaluated potential endocrine activities of styrene. In vitro and in vivo screening studies, as well as non-guideline and guideline investigations in experimental animals indicate that S is not associated with (anti)estrogenic, (anti)androgenic, or thyroid-modulating activity or with an endocrine activity that may be relevant for the environment. Studies in exposed workers have suggested elevated PRL levels that have been further examined in a series of human and animal investigations. While there is only one definitively known physiological function of PRL, namely stimulation of milk production, many normal stress situations may lead to elevations without any chemical exposure. Animal studies on various aspects of dopamine (DA), the PRL-regulating neurotransmitter, in the central nervous system did not give mechanistic explanations on how Styrene may affect PRL levels. Overall, a neuroendocrine disruption of PRL regulation cannot be deduced from a large experimental database. The effects in workers could not consistently be reproduced in experimental animals and the findings in humans represented acute reversible effects clearly
below clinical and pathological levels. Therefore, unspecific acute workplace-related stress is proposed as an alternative mode of action for elevated PRL levels in workers.
Borgert (2022) evaluated for its potential to disrupt estrogen, androgen, thyroid, and steroidogenic (EATS) endpoints using a rigorous WoE methodology that included problem formulation, systematic literature search and selection, data quality evaluation, relevance weighting of endpoint data, and application of specific interpretive criteria. Sufficient data were available to assess the endocrine disruptive potential of styrene based on endpoints that would respond to EATS MoAs in some Tier 1-type and many Tier 2-type reproductive,
developmental, and repeat dose toxicity studies. Responses to styrene were inconsistent with
patterns of responses expected for chemicals and hormones known to operate via EATS MoAs, and thus, styrene cannot be deemed an endocrine disruptor, a potential endocrine disruptor, or to exhibit endocrine disruptive properties. Because Tier 1 EDSP screening results would trigger Tier 2 studies, like those evaluated here, subjecting styrene to further endocrine screening would produce no additional useful information.
References not listed in the basic data set
Bogert, C. (2022) Hypothesis-driven weight of evidence evaluation indicates
styrene lacks endocrine disruption potential, Critical Reviews in Toxicology, in press
Cappaert, N.L., et al. (2002). Differential susceptibility of rats and guinea pigs to the ototoxic effects of ethyl benzene. Neurotoxicol Teratol. 24(4): 503-10.
Crofton K.M. et al. (1994). Solvent-induced ototoxicity in rats: an atypical selective mid-frequency hearing deficit. Hearing Research 80:25–30.
Davis R. (2002) Susceptibility to the ototoxic properties of toluene is species specific. Hearing Research 166(1): 24-32.
Ladefoged O. et al. (1998). Toxicity of the styrene metabolite, phenylglyoxylic acid, in rats after three months' oral dosing. Neurotoxicology 19 (4-5): 721-737.
Lataye R. et al. (2001). Cochlear pathology induced by styrene. Neurotoxicology and Teratology 23(1): 71-79.
Muttray A Wolters V Jung D Konietzko J. 1999. Neurotoxicol Teratol. 1999 Jan-Feb;21(1):41-5.
Nylen, P. et al. (1987). Inhalation exposure to toluene; effect of daily dose on auditory sensitivity and nerve conduction velocity in rats. Proceedings of the XXII International Congress on Occupational Health.1987.
Pouyatos B. et al. (2002). Use of DPOAEs for assessing hearing loss caused by styrene in the rat. Hearing Research 265:156-164.
Prosen C.A. et al. (1990). Apical hair cells and hearing. HearingResearch 44: 179-194.
Pryor, G.T. et al. (1984). Factors affecting toluene-induced ototoxicity in rats. Neurobehav Toxicol Teratol. 6(3): 223-38.
Pryor, G., et al. (1991). The hearing loss associated with exposure to toluene is not caused by a metabolite. Brain Res Bull. 27(1): 109-13.
Yanagiba Y. et al. 2005. Environmental Health Perspectives 116: 740-745
Zamyslowska-Szmytke et al. (2009). Temporal Processing Disorder Associated with Styrene Exposure. Audiol Neurotol. 14:296–302.
Justification for classification or non-classification
In November 2012 RAC (2012) assessed the evidence for impairment of hearing ability and colour discrimination. For ototoxicity it was concluded “that appropriate studies in experimental animals in combination with reliable epidemiological studies provide evidence for adverse effects on hearing (ototoxicity), and that this is a sufficient basis for classification with STOT RE. Regarding the category, the RAC finds that the evidence for ototoxicity occurring in humans at concentrations below 50 ppm warrant classification in category 1, even though the concentrations needed to cause ototoxicity in rats would suggest a lower category.” The possibility that deficits for colour discrimination may be caused by exposure to styrene was assessed as follows: “The effects of styrene on colour vision in humans can be viewed as supportive of the STOT classification, but the degree to which this effect can be considered to be adverse is difficult to establish based on the proposal. Therefore, the RAC does not find that this effect, as described in the CLH proposal, as such is a sufficient basis for classification.”
Overall, the following classification was proposed by RAC (2012): STOT RE 1; H372 “Causes damage to the hearing organs through prolonged or repeated exposure” (corresponding to Xn; R48/20/22). This proposal corresponds to that given in the CSR.
References
RAC (2012). Opinion proposing harmonised classification and labelling at EU level of styrene; adopted 28 November 2012.
Information on Registered Substances comes from registration dossiers which have been assigned a registration number. The assignment of a registration number does however not guarantee that the information in the dossier is correct or that the dossier is compliant with Regulation (EC) No 1907/2006 (the REACH Regulation). This information has not been reviewed or verified by the Agency or any other authority. The content is subject to change without prior notice.
Reproduction or further distribution of this information may be subject to copyright protection. Use of the information without obtaining the permission from the owner(s) of the respective information might violate the rights of the owner.
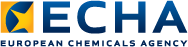