Registration Dossier
Registration Dossier
Data platform availability banner - registered substances factsheets
Please be aware that this old REACH registration data factsheet is no longer maintained; it remains frozen as of 19th May 2023.
The new ECHA CHEM database has been released by ECHA, and it now contains all REACH registration data. There are more details on the transition of ECHA's published data to ECHA CHEM here.
Diss Factsheets
Use of this information is subject to copyright laws and may require the permission of the owner of the information, as described in the ECHA Legal Notice.
EC number: 242-670-9 | CAS number: 18917-91-4
- Life Cycle description
- Uses advised against
- Endpoint summary
- Appearance / physical state / colour
- Melting point / freezing point
- Boiling point
- Density
- Particle size distribution (Granulometry)
- Vapour pressure
- Partition coefficient
- Water solubility
- Solubility in organic solvents / fat solubility
- Surface tension
- Flash point
- Auto flammability
- Flammability
- Explosiveness
- Oxidising properties
- Oxidation reduction potential
- Stability in organic solvents and identity of relevant degradation products
- Storage stability and reactivity towards container material
- Stability: thermal, sunlight, metals
- pH
- Dissociation constant
- Viscosity
- Additional physico-chemical information
- Additional physico-chemical properties of nanomaterials
- Nanomaterial agglomeration / aggregation
- Nanomaterial crystalline phase
- Nanomaterial crystallite and grain size
- Nanomaterial aspect ratio / shape
- Nanomaterial specific surface area
- Nanomaterial Zeta potential
- Nanomaterial surface chemistry
- Nanomaterial dustiness
- Nanomaterial porosity
- Nanomaterial pour density
- Nanomaterial photocatalytic activity
- Nanomaterial radical formation potential
- Nanomaterial catalytic activity
- Endpoint summary
- Stability
- Biodegradation
- Bioaccumulation
- Transport and distribution
- Environmental data
- Additional information on environmental fate and behaviour
- Ecotoxicological Summary
- Aquatic toxicity
- Endpoint summary
- Short-term toxicity to fish
- Long-term toxicity to fish
- Short-term toxicity to aquatic invertebrates
- Long-term toxicity to aquatic invertebrates
- Toxicity to aquatic algae and cyanobacteria
- Toxicity to aquatic plants other than algae
- Toxicity to microorganisms
- Endocrine disrupter testing in aquatic vertebrates – in vivo
- Toxicity to other aquatic organisms
- Sediment toxicity
- Terrestrial toxicity
- Biological effects monitoring
- Biotransformation and kinetics
- Additional ecotoxological information
- Toxicological Summary
- Toxicokinetics, metabolism and distribution
- Acute Toxicity
- Irritation / corrosion
- Sensitisation
- Repeated dose toxicity
- Genetic toxicity
- Carcinogenicity
- Toxicity to reproduction
- Specific investigations
- Exposure related observations in humans
- Toxic effects on livestock and pets
- Additional toxicological data

Endpoint summary
Administrative data
Key value for chemical safety assessment
Additional information
For Aluminium trilactate a reverse gene mutation assay in bacteria (Ames test), a mammalian cell gene mutation assay and an in vitro micronucleus test are available. Data on other Aluminium compounds have also been taken into account for hazard assessment, as it is assumed that after intake Aluminium trilactate is changed to ionic Aluminium and Lactate and that only ionic Aluminium is determining biological activities. Thus, a read-across to other Aluminium compounds is appropriate.
Ames test:
In a reverse gene mutation assay in bacteria according to OECD guideline 471, adopted July 21, 1997, strains TA 1535, TA 1537, TA 98, TA 100 of S. typhimurium and E. coli WP2uvrA were exposed to Aluminium trilactate (93.1% a.i.) at concentrations of 3, 10, 33, 100, 333, 1000, 3330 and 5000 μg/plate in the absence and presence of mammalian metabolic activation (S9-mix).
Aluminium trilactate was tested up to limit concentration (5000 µg/plate).
Toxicity was observed at 3330 µg/plate and higher in all Salmonella tester strains in the absence of S9 mix and at 5000 µg/plate inTA1535 in the presence of S9 mix; no toxicity was observed in E. coli WP2uvrA at all tested concentrations. The positive controls induced the appropriate responses in the corresponding strains. There was no evidence of induced mutant colonies over background. Aluminium trilactate was not mutagenic in this bacterialreverse gene mutation assay.
Mammalian cell gene mutation test:
In a mammalian cell gene mutation assay according to OECD guideline 467, adopted July 21, 1997 (thymidine kinase (TK)), L5178Y mouse lymphoma cells cultured in vitro were exposed to Aluminium trilactate (93.1%) in DMSO in the following concentrations in the presence and absence of mammalian metabolic activation (S9 mix):
First experiment
Without S9-mix: 3, 10, 33, 100, 333, 1000, 1500, 2000μg/mL
With 8% (v/v) S9-mix: 0.1, 1, 3.3, 10, 33, 100, 333 and 1000μg/mL
Second experiment
Without S9-mix: 3, 10, 33, 100, 333, 750, 1000μg/mL
With 12% (v/v) S9-mix: 0.1, 1, 3, 10, 33, 100, 333 and 1000μg/mL
Aluminium trilactate was tested up to cyctotoxic concentrations. The positive controls induced the appropriate response. There was no evidence of induced mutant colonies over background.
In vitro chromosome aberration assay:
In a mammalian cell micronucleus assay according to OECD guideline 487, July 22, 2010, primary human lymphocyte cultures were exposed to Aluminium trilactate (93.1% a.i.) in DMSO at the following concentrations:
Experiment 1A: 100, 1000, 2000, 2600, 2700 and 2800 µg/mL
Experiment 1B: 100, 2000, 2600, 2625, 2650, 2675 and 2700 µg/mL
Experiment 1C: 100, 1000, 2000, 2600, 2650 and 2700 µg/mL
Experiment 2: 500, 1000, 1500, 2000, 2500 and 3000 µg/mL
Aluminium trilactate was tested up to cytotoxic concentrations.
In the first cytogenetic assay in the absence of S9-mix, at the 3 hours exposure time, the test substance induced a statistically significant increase in the number of binucleated cells with micronuclei at the highest dose level of 2600 µg/mL. In the presence of S9-mix, the test substance induced a dose dependent statistically significant increase in the number of binucleated cells with micronuclei.
In the second cytogenetic assay with a 24 hours continuous exposure time, the test substance induced a dose dependent, statistically significant increase in the number of binucleated cells with micronuclei. There was evidence of micronucleated cells induced over background.
Aluminium trilactate was not mutagenic in bacterial or mammalian cell gene mutation assays.
However, the available data on Aluminium-induced DNA damage is ambiguous; some in vitro as well as in vivo studies and publications on Aluminium genotoxicity show positive responses for the induction of micronuclei, chromatid type aberrations and sister chromatid exchanges while others do not. These are considered in a weight of evidence approach.
In vitro chromosome aberration assays, DNA damage assays:
Banasik (2005):
In a mammalian cell micronucleus assay primary human lymphocyte cultures were exposed to Aluminium chloride at concentrations of 0, 1, 2, 5, 10, and 25 µg AlCl3/mL (corresponding to 0, 0.20, 0.40, 1.01, 2.02 and 5.06 µg Al/mL based on an Al content of 20.24%) during different stages of the cell cycle.
Al induced micronuclei and apoptosis at all concentrations tested. With an increasing concentration of Al (> 2 µg Al/mL), the frequency of micronuclei decreased, however, the apoptosis rate increased. The frequencies of micronuclei in cells treated during G0/G1 or S/G2 were comparable. The treatment in G0/G1 lasted only for 4 h, whereas that in S/G2 lasted for 62 h. This, and the low frequency of apoptosis in cells treated during S/G2, suggests that the G0/G1 phase of the cell cycle is especially sensitive to Al.
There was a statistically significant, dose dependent increase of micronucleated cells induced over background.Therefore, Aluminium might be considered an aneugenic or clastogenic compound in vitro.
Lima et al. (2007):
In a mammalian cell chromosome aberration assay primary human lymphocyte cultures were exposed to Aluminium chloride at concentrations of 0, 5, 10, 15 and 25 µM AlCl3 (corresponding to 0, 0.135, 0.270, 0.405 and 0.675 mg Al/L) during different stages of the cell cycle.
At G1 and G1/S, the frequency of CAs was significantly increased with all the tested concentrations of AlCl3. During G1 and G2 phase treatments, significantly increased endoreduplication and polyploidy were observed. AlCl3 treatment during S phase resulted in highly significant increases in the frequency of CAs in most concentrations. The treatment at G2 phase also induced a significant increase in the frequency of CAs.
Chromatid gaps and chromatid breaks were the most frequent CAs.
The cytotoxic effects of AlCl3 were observed as decrease in the MI of lymphocyte in cultures treated during the G1, G1/S, S and G2 phases of the cell cycle.
The total chromosome aberrations (including gaps) were significantly higher in all cultures treated with AlCl3 in cells in the G1 and G1/S phase, the S phase and also the G2 phase. However, the biological relevance of these results is unclear due to the high cytotoxicity in the cultures.
There was a statistically significant, dose dependent increase of chromosome aberrations induced over background.
In the same publication, DNA damage after exposure to Aluminium was examined:
In a mammalian cell single cell gel/comet assay primary human lymphocyte cultures were exposed to Aluminium chloride at concentrations of 0, 5, 10, 15 and 25 µM AlCl3 (corresponding to 0, 0.135, 0.270, 0.405 and 0.675 mg Al/L) for 3 h. The alkaline version of the comet assay was performed.
A significant increase in DNA damage was observed at all concentrations compared to the vehicle control, however, the level was equally high at all concentrations. No information is available on the apoptosis rate. Thus, no discrimination of effects from cytoxicity from effects due to genotoxicity is possible.
Caicedo et al. (2008):
In a mammalian cell single cell gel/comet assay Human CD4+ T cells (Jurkat cells) were exposed to Aluminium chloride at concentrations of 0.0 (control), 0.05, 0.1, 0.5, 1, and 5 mM (corresponding to 0, 1.35, 2.7, 13.5, 27 and 135 mg Al/L) for 48 h.
The indices of DNA damage was < 60 for all concentrations of Al tested. At 5 mM Al, significant apoptosis was observed, but not at lower concentrations. Viability and proliferation of the cells were not significantly influenced by Al-treatment.
Aluminium chloride did not induce significant DNA-damage in the single cell gel/comet assay.
In vivo chromosome aberration assays, DNA damage assays:
Roy et al. (1991):
In a rat bone marrow micronucleus assay, 5 male rats/dose were treated orally with Aluminium sulphate (Al2(SO4)3 x 18H2O) at doses of 0, 212, 265, 353, 530, 1060, 2120 mg Al2(SO4)3x18H2O/kg bw/d, corresponding to 0, 17, 22, 28, 43, 85, 172 mg Al/kg bw/d
daily for 7, 14 or 21 days. Bone marrow cells were harvested at 24 h post-treatment. The vehicle was deionised water.
In the bone marrow cells, the mitotic index decreased in direct proportion to the dose used. The decrease was highly pronounced in animals treated with the two higher doses.
The frequency of abnormal cells increased with dose and duration of exposure to Aluminium sulphate. During all three sampling times the trend test p value was found to be highly significant. A duration dependent effect was not found with the lowest (7 mg Al/kg bw/d) dose. Most aberrations were chromatid breaks. Translocations were recorded in higher doses.
There was a significant increase in the frequency of micronucleated polychromatic erythrocytes in bone marrow after any treatment time.
Balasubramanyam et al.(2009):
In a Wistar rat peripheral blood micronucleus assay according to OECD guideline 474, adopted 21st July 1997, 5 female rats/dose were treated orally (gavage) with Al2O3 as bulk material, or nanoscaled Al2O3 (30 nm, 40 nm) (>90% a.i.) at doses of 0, 500, 1000 and 2000 mg Al2O3/kg bw (corresponding to 0, 264.6, 529.2 and 1058.4 mg Al/kg bw). Peripheral blood was collected from retro-orbital plexus 48 and 72 h post-treatment. The vehicle was 1% Tween80 in water.
There were no signs of toxicity during the study. No statistically significant dose-dependent increase in the frequency of micronuclei was observed for the bulk material. Whereas treatment with the nanoscaled Al2O3 resulted in a statistically significant dose-dependent increase in the frequency of micronuclei. Al2O3 accumulated in all examined tissues: whole blood, liver, heart, kidneys, brain and spleen in the groups of animals sampled 14 days after treatment with all three doses (500, 1000 and 2000 mg/kg bw) of Al2O3-30 nm, Al2O3-40 nm and Al2O3-bulk.
The biodistribution of Al2O3 showed statistically significant increases in different tissues and urine after treatment with 1000 and 2000 mg/kg bw Al2O3-30 nm and Al2O3-40 nm compared to the control. The maximum amount of accumulation was seen in the kidneys followed by whole blood, liver and brain of animals after treatment with all three doses of Al2O3-30 nm and Al2O3-40 nm.
Al2O3-bulk did not show statistically significant accumulation in any of the tissues and urine compared to the control. It showed largest retention in feces.
There was no significant increase in the frequency of micronucleated polychromatic erythrocytes in peripheral blood after any treatment time for bulk Al2O3.
In the same study a comet assay was performed: Peripheral blood was collected from retro-orbital plexus 4, 24, 48 and 72 h post-treatment. The vehicle was 1% Tween80 in water.
There were no signs of toxicity during the study. No differences in % Tail DNA were observed for the bulk material whereas treatment with the nanoscaled Al2O3 resulted in a statistically significant increase in % Tail DNA.
There was no statistically significant increase in % Tail DNA in peripheral blood cells after any observation time for bulk Al2O3.
Nanoscaled Al2O3 is orally absorbed and distributed in the body to a much higher extent than the bulk material. Only the nanoscaled Al2O3 caused micronuclei and DNA damage, but not the bulk material.
Dhir et al. (1990):
In a Swiss mouse bone marrow chromosome aberration assay, 6 animals/dose (sex not specified) were treated intraperitoneally with Aluminium sulphate at doses of 0, 250, 500, 1000 mg Al2(SO4)3 x 18H2O/kg bw, corresponding to 0, 20, 40, 80 mg Al/kg bw. Bone marrow cells were harvested at 24 h post-treatment. The vehicle was saline.
A dose-dependent increase in chromosome aberrations was observed in mouse bone marrow cells after intraperitoneal injection of Aluminium sulphate. The mitotic index was reduced in the 500 and 1000 mg/kg bw dose group (corresponding to 40 and 80 mg Al/kg bw).
Oral administration of Phyllanthus fruit extract (PFE) for 7 days before exposure of Aluminium increased the frequency of cell division and reduced the frequency of chromosome breaks. Comparable doses of synthetic ascorbic acid were less effective, bur still protected against the clastogenic effect of Aluminium.
The positive control induced the appropriate response. There was a significant increase in the frequency of chromosome aberrations in bone marrow cells after any treatment time.
Manna, Das (1972):
In a mouse bone marrow chromosome aberration assay, 4 animals/dose (sex not specified) were treated intraperitoneally with Aluminium chloride at doses of 0, 0.01 M, 0.05 M, 0.1 M AlCl3 al 1 mL/30 g bw, corresponding to 0, 9, 45, 90 mg Al/kg bw.
Bone marrow cells were harvested at 1, 2, 4, 8, 12, 16, 20, 24, 48 and 72 h in the 0.1 M dose group and at 20 h in the 0.05 and 0.01 M dose groups. The vehicle was water.
A dose-dependant increase in chromosome aberrations was observed in mouse bone marrow cells after intraperitoneal injection of Aluminium chloride at all sampling times. However, no information in cytotoxicity is available.
Roy et al. (1992):
In a Swiss mouse bone marrow micronucleus assay, 6 animals/dose (sex not specified) were treated intraperitoneally with Aluminium sulphate at doses of 0, 250, 500 mg Al2(SO4)3x18H2O/kg bw/d, corresponding to 0, 20, 40 mg Al/kg bw/d for two consecutive days. Bone marrow cells were harvested at 24 h and 48 h after the last treatment. The vehicle was saline.
A dose-dependent increase in chromosome aberrations was observed in mouse bone marrow cells after intraperitoneal injection of Aluminium sulphate. The increase of micronuclei frequency was not significant at 250 mg/kg bw, but significant (p<0.05) at 500 mg/kg bw.
Oral administration of Phyllanthus fruit extract or ascorbic acid for 7 days before exposure of Aluminium reduced the frequency of micronuclei. The positive control induced the appropriate response. There was a dose-dependant increase in the frequency of micronuclei in bone marrow cells after treatment with Aluminium sulphate.
In the ECHA database of registered substances in vivo micronucleus tests with Aluminium chlorohydrate (ECHA a) and Aluminium hydroxide (ECHA b) are listed.
In an in vivo micronucleus test in mice Locron P (Aluminium chlorohydrate) was administered at 2000 mg/kg bw by oral gavage twice in an 24 h interval. No toxic effects were reported in the study, however, in a 7 day dose range finding study, slight toxic effects (not specified) were observed. In this study no micronuclei were induced over background.
In a further in vivo micronucleus test Aluminium hydroxide was administered to mice by oral gavage at a dose of 2000 mg/kg bw twice in 24 h interval. No toxic effects were observed, no micronuclei were induced over background.
Some data are available concerning a possible mechanism of genotoxicity of Al: In vitro, interactions of Al with DNA have been reported, such as cross-linking of chromosomal proteins to DNA (Wedrychowski, 1986; EFSA, 2008). Zhang et al. (2002) determined the binding site of Al to be the Phosphate backbone of the DNA. It is further described, that the interaction is primarily electrostatic and reversible rather than covalent (Martin, 1986).
This is in line with the opinion of EFSA (2008) concluding that “Aluminium compounds were non-mutagenic in bacterial and mammalian cell systems, but some produced DNA damage and effects on chromosome integrity and segregation in vitro. Several indirect mechanisms have been proposed and the Panel noted that these indirect mechanisms of genotoxicity, occurring at relatively high levels of exposure, are unlikely to be of relevance for humans exposed to aluminium via the diet.”
Considering the low oral bioavailability of Aluminium of ca. 1%, systemic exposure due to the use of Aluminium trilactate is low. However, the most relevant route of exposure for Aluminium trilactate is the dermal route. Dermal absorption of Al is even lower (ca. 0.1% as was shown in more detail in section “Toxicokinetics, metabolism and distribution”) leading to a lower systemic availability of Al due to dermal exposure.
In addition to low systemic availability, Al is efficiently eliminated from the organism. Al absorbed from the blood is eliminated primarily by the kidneys, and excreted in the urine. In animal studies reviewed by EFSA (2008) it was found, that Al is not equally distributed throughout the body of rats following dietary exposure. Al was found to a larger extent in spleen, liver, bone, and kidneys than in brain, muscle, heart, and lung. Three days after withdrawal of Al from the diet, Al concentrations in these tissues decreased significantly.
Moreover, Aluminium occurs naturally in a broad range of food. Several Al compounds are also used as food additives (e.g. E554Sodium aluminium silicate, E555 Potassium aluminium silicate, E556 Calcium aluminium silicate, E559 Aluminium silicate).EFSA (2008, 2011) has reviewed the safety of aluminium from dietary intake. “Mean dietary exposure from water and food in non-occupational exposed adults showed large variations between the different countries and, within a country, between different surveys. It ranged from 1.6 to 13 mg aluminium per day, corresponding to 0.2 to 1.5 mg/kg body weight (bw) per week in a 60 kg adult” (EFSA, 2008).The dietary exposure to Al from treated drinking water is estimated to be in the range of up to 0.4 mg/d. Normal serum levels of Aluminium are reported to be ranging from 1 to 3 µg/L (EFSA, 2008). In patients with dementia an increase in serum Aluminium levels up to 18 µg/L was found as cited by EFSA (2008).
In relation to Aluminium exposure from natural sources (food, drinking water), the additional Al uptake from Aluminium trilactate is low as can be taken from the exposure data in chapter 9 of the Aluminium trilactate CSR.
Considering the low systemic bioavailability of Aluminium and low exposure to Aluminium trilactate, we follow the argumentation of EFSA (2008) and come to the conclusion, that the positive findings in chromosome aberration and micronucleus assays do not pose a risk to humans as these effects are not biologically relevant for humans. The described effects have been observed at concentration levels which are highly unlikely to be achieved under normal physiological conditions.
References
Balasubramanyam et al.(2009) In vivo genotoxicity assessment of aluminium oxide nanomaterials in rat peripheral blood cells using the comet assay and micronucleus test. Mutagenesis 24(3):245–251
Banasik et al. (2005) Aluminum-Induced Micronuclei and Apoptosis in Human Peripheral-Blood Lymphocytes Treated during Different Phases of the Cell Cycle. Environ Toxicol. 20(4):402-6
Caicedo et al. (2008) Analysis of metal ion-induced DNA damage, apoptosis, and necrosis in human (Jurkat) T-cells demonstrates Ni2+ and V3+ are more toxic than other metals: Al3+, Be2+, Co2+, Cr3+, Cu2+, Fe3+, Mo5+, Nb5+, Zr2+. Journal of Biomedical Materials Research Part A 86(4):905-913
Dhir et al. (1990) Modification of clastogenicity of lead and aluminium in mouse bone marrow cells by dietary ingestion of Phyllanthus emblica fruit extract. Mutation Research 241: 305-312
ECHA a: http://apps.echa.europa.eu/registered/data/dossiers/DISS-9eb3d71d-f83e-1b7e-e044-00144f67d031/AGGR-0e36333c-c7c3-4184-958c-8aa47c33c9a8_DISS-9eb3d71d-f83e-1b7e-e044-00144f67d031.html#AGGR-0e36333c-c7c3-4184-958c-8aa47c33c9a8
ECHA b: http://apps.echa.europa.eu/registered/data/dossiers/DISS-9eb72770-3cba-0c3b-e044-00144f67d031/AGGR-5857e19c-3d2e-452b-b553 -7831cd422fbe_DISS-9eb72770-3cba-0c3b-e044-00144f67d031.html#AGGR-5857e19c-3d2e-452b-b553-7831cd422fbe
EFSA (2008) Safety of aluminium from dietary intake, The EFSA Journal 754, 1-34, available via internet: http://www.efsa.europa.eu/de/efsajournal/pub/754.htm
EFSA (2011a) STATEMENT OF EFSA On the Evaluation of a new study related to the bioavailability of aluminium in food, EFSA Journal 2011;9(5):2157, available via internet: http://www.efsa.europa.eu/de/efsajournal/pub/2157.htm
Lima et al. (2007) Genotoxic effects of aluminum chloride in cultured human lymphocytes treated in different phases of cell cycle.Food and Chemical Toxicology 45, 1154–1159
Manna, Das (1972) Chromosome aberrations in mice induced by aluminium chloride. The nucleus 15(3): 180-186Martin, RB (1986) The Chemistry of Aluminum as related to biology and medicine. Clin. Chem. 32/10, 1797-1806
Roy et al. (1991) Effects of aluminium salts on bone marrow chromosomes in rats in vivo. Cytobios 66: 105-111
Roy et al. (1992) Modification of metal-induced micronuclei formation in mouse bone marrow erythrocytes by Phyllanthus fruit extract and ascorbic acid. Toxicology Letters 62: 9-17
Wedrychowski A (1986)The in Vivo Cross-linking of Proteins and DNA by Heavy Metals. Journal of biological chemistry,Vol. 261, No.7,pp. 3370 -3376
Zhang RY et al. (2002) Spectroscopic and voltammetric study on the binding of Aluminium (III) to DNA. Analytical sciences 18(7):761-6
Justification for selection of genetic toxicity endpoint
No single key study has been selected, instead all available data are considered in a weight of evidence approach.
Short description of key information:
Aluminium trilactate is not mutagenic in the bacterial reverse mutation assay (Ames test) and the mammalian cell gene mutation assay (TK test) using L5178Y mouse lymphoma cells.
The available data on Aluminium-induced DNA damage is ambiguous; some in vitro as well as in vivo studies and publications on Aluminium genotoxicity show positive responses for the induction of micronuclei, chromatid type aberrations and sister chromatid exchanges while others do not. These are considered in a weight of evidence approach.
Taking together the low systemic bioavailability of Aluminium and low exposure to Aluminium trilactate, it is concluded, that the positive findings in chromosome aberration and micronucleus assays do not pose a risk to humans due to lacking biological relevance. The described effects have been observed at concentration levels which are highly unlikely to be achieved under normal physiological conditions.
Endpoint Conclusion: Adverse effect observed (positive)
Justification for classification or non-classification
Based on the available data we come to the conclusion, that the positive findings in chromosome aberration and micronucleus assays do not pose a risk to humans as these effects are not biologically relevant for humans. The above described effects have been observed at concentration levels which are highly unlikely to be achieved under normal physiological conditions. Thus, Aluminium trilactate does not need to be classified for mutagenicity according to the criteria given in regulation (EC) 1272/2008 or the former European directive on classification and labelling 67/548/EEC. No labelling is required.
Information on Registered Substances comes from registration dossiers which have been assigned a registration number. The assignment of a registration number does however not guarantee that the information in the dossier is correct or that the dossier is compliant with Regulation (EC) No 1907/2006 (the REACH Regulation). This information has not been reviewed or verified by the Agency or any other authority. The content is subject to change without prior notice.
Reproduction or further distribution of this information may be subject to copyright protection. Use of the information without obtaining the permission from the owner(s) of the respective information might violate the rights of the owner.
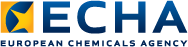