Registration Dossier
Registration Dossier
Data platform availability banner - registered substances factsheets
Please be aware that this old REACH registration data factsheet is no longer maintained; it remains frozen as of 19th May 2023.
The new ECHA CHEM database has been released by ECHA, and it now contains all REACH registration data. There are more details on the transition of ECHA's published data to ECHA CHEM here.
Diss Factsheets
Use of this information is subject to copyright laws and may require the permission of the owner of the information, as described in the ECHA Legal Notice.
EC number: 237-280-0 | CAS number: 13718-59-7
- Life Cycle description
- Uses advised against
- Endpoint summary
- Appearance / physical state / colour
- Melting point / freezing point
- Boiling point
- Density
- Particle size distribution (Granulometry)
- Vapour pressure
- Partition coefficient
- Water solubility
- Solubility in organic solvents / fat solubility
- Surface tension
- Flash point
- Auto flammability
- Flammability
- Explosiveness
- Oxidising properties
- Oxidation reduction potential
- Stability in organic solvents and identity of relevant degradation products
- Storage stability and reactivity towards container material
- Stability: thermal, sunlight, metals
- pH
- Dissociation constant
- Viscosity
- Additional physico-chemical information
- Additional physico-chemical properties of nanomaterials
- Nanomaterial agglomeration / aggregation
- Nanomaterial crystalline phase
- Nanomaterial crystallite and grain size
- Nanomaterial aspect ratio / shape
- Nanomaterial specific surface area
- Nanomaterial Zeta potential
- Nanomaterial surface chemistry
- Nanomaterial dustiness
- Nanomaterial porosity
- Nanomaterial pour density
- Nanomaterial photocatalytic activity
- Nanomaterial radical formation potential
- Nanomaterial catalytic activity
- Endpoint summary
- Stability
- Biodegradation
- Bioaccumulation
- Transport and distribution
- Environmental data
- Additional information on environmental fate and behaviour
- Ecotoxicological Summary
- Aquatic toxicity
- Endpoint summary
- Short-term toxicity to fish
- Long-term toxicity to fish
- Short-term toxicity to aquatic invertebrates
- Long-term toxicity to aquatic invertebrates
- Toxicity to aquatic algae and cyanobacteria
- Toxicity to aquatic plants other than algae
- Toxicity to microorganisms
- Endocrine disrupter testing in aquatic vertebrates – in vivo
- Toxicity to other aquatic organisms
- Sediment toxicity
- Terrestrial toxicity
- Biological effects monitoring
- Biotransformation and kinetics
- Additional ecotoxological information
- Toxicological Summary
- Toxicokinetics, metabolism and distribution
- Acute Toxicity
- Irritation / corrosion
- Sensitisation
- Repeated dose toxicity
- Genetic toxicity
- Carcinogenicity
- Toxicity to reproduction
- Specific investigations
- Exposure related observations in humans
- Toxic effects on livestock and pets
- Additional toxicological data

Endpoint summary
Administrative data
Link to relevant study record(s)
Description of key information
- Transformation into selenophosphate and selenocysteinyl tRNA, resulting in the incorporation of Se in selenoproteins,
- Trandsformation into methylated metabolites of selenide which are then excreted via the urine (Lobinski et al. 2000).
-
glutathione peroxidases, related to the protection of cells from oxidative damage;
-
type 1iodothyronine deiodinase
-
thioredoxin reductase
General introduction
Selenium is an essential micro-nutrient that is used in the body in enzymes that protect the body from oxidative damage, or in enzymes related to growth and metabolism.
The majority of the daily intake of selenium occurs via oral uptake (food, and to a lesser extent, via drinking water), but can also be taken up via inhalation. Organic selenium compounds in food (e.g. selenomethionine and selencysteine in grains, cereals, and forage crops) are easily absorbed by the human body, and selenium is made available where needed. Inorganic selenium compounds – such as sodium selenate and sodium selenite, are the main speciation forms that are found in drinking water, and are also absorbed from the digestive tract. These inorganic forms are then transformed into (organic) forms that can be used by the human body.
Being an essential element, the human body has several mechanisms that regulate the internal Se-concentration levels (uptake and elimination mechanisms). However, if exposure levels are very high, or if exposure occurs over a long period of time, selenium built-up in the body can take place (in liver, kidney, blood, lungs, heart, testes, nails and hair), resulting in adverse dermal and neurological effects referred to as selenosis. Se-deficiency also results in adverse health effects (muscle pain, heart problems, lung-effects in premature babies). Two endemic diseases in seleniumpoor regions of China are related to Se-deficiency: Keshan Disease and KashinBeck Disease.
When looking at inorganic forms, selenite is absorbed by simple diffusion whereas selenate is absorbed by a co-transport sodium selenate and exchange selenate/OH-. The uptake of organic forms (Se-methionine, Se-cysteine) follows the typical mechanisms of amino-acid uptake. As a result of these differences in uptake mechanisms, the absorption efficiency of selenite is 79 and 80% in poultry and pork, respectively, whereas the uptake of selenates and Se-methionine is >90% for poultry and monogastrics (Meschy, 2010).
Fate and speciation of Se in the human body
The metabolism of selenium in mammals is fairly well understood. To become incorporated into seleniumspecific proteins (e.g., glutathione peroxidase, thioredoxin reductase, iodothyronine 5'deiodinase) through a co-translational mechanism requires that selenium be in the form of selenide (Sunde 1990). All forms of selenium can be transformed to selenide, although the rates of transformation vary. For example, selenate is not converted to selenide as readily as selenite. Further research is required to determine which (in)organic selenium metabolites or intermediates lead to toxicity. Figure 1 presents an overall overview of the various pathways that have been identified.
Inorganic Se-compounds are reduced stepwise to the assumed key intermediate hydrogen selenide (or related species). This speciation form(s) then undergoes one of the two following processes:
It can thus be concluded that selenium in the mammalian body is mainly present in forms of covalent carbonselenium bonds. The most relevant organic Se-compounds are selenoprotein P (the principal selenoprotein in plasma) and selenoenzymes. Examples of the latter group are (Holmgren and Kumar 1989; Lobinski et al. 2000):
Selenide, which can non-specifically bind to proteins, is thus the selenium species that plays a central role in the various pathways related to the formation and degradation of selenium proteins. It can be formed from selenite by reduction via glutathione following uptake in red blood cells. Such a transformation pathway has been identified in both humans (Lee et al. 1969; Mas and Sarkar, 1989) and rats (Gasiewicz and Smith, 1978). Reduction of selenite or selenate to dimethyl selenide requires glutathione and the methylating agent Sadenosylmethionine. Selenide is thus the form of selenium that is transferred to the liver upon binding to albumin in blood plasma. Once in the liver, the selenide is methylated for excretion via urine (mono-, di-, or tri-methylated selenide, depending on the selenide concentration) or incorporated into proteins.
Selenate is transformed to selenite. There is no evidence that (inorganic) selenate is incorporated non-specifically into plasma protein, suggesting that this form is metabolized by specific selenium metabolic processes. Suzuki and Ogra (2002) did report that selenate could also be taken up directly by the liver or excreted via urine. Nevertheless, data from an injection study with sodium selenate and sodium selenite in rats (Hirooka and Galambos 1966) showed that dimethyl selenide was the primary metabolite that was excreted via respiration, and the transformation process from selenate to methyl selenide (via selenite) appears to take place in the liver (Nakamuro et al. 1977). Pathways for the formation of dimethyl selenide from selenite in animals have been proposed by Ganther (1971). Intraperitoneal administration of several Se-substances to rats, including sodium selenite (Byard and Baumann 1967) and sodium selenate (Palmer et al. 1970) also resulted in a common predominant urinary metabolite, namely the trimethylselenonium ion. These observations confirm that different inorganic Se-compounds are rapidly transformed into common Se-forms, and therefore potential systemic adverse effects due to increased Se-levels are expected to be similar for the inorganic Se-compounds that enter the body.
Further support for this conclusion can be found in several studies that reported on distribution patterns in the body upon uptake. Overall they reported similar distribution patterns, regardless of the form in which it was administered. The fact that all inorganic Se-compounds are converted into a common selenide-form can explain this observation. It is noteworthy, however, that internal concentration levels are generally higher for doses delivered as an organic form of selenium (e.g. selenomethionine) than for the same dose delivered as an inorganic form (Behne et al. 1991; Butler et al. 1990; Grønbaek and ThorlaciusUssing 1992; Ip and Hayes 1989; Salbe and Levander 1990; Shiobara et al. 1998). It should also be noted that the conversion of selenate to dimethyl selenide is apparently not as readily in comparison to the conversion of selenite to dimethylselenide. Studies with e.g. yeast indicate that selenate must be activated prior to conversion to selenite (Bopp et al. 1982). Unfortunately, studies of selenate metabolism in mammals are limited.
Adsorption rate of selenites in humans and animals after ingestion often exceeds 80% of the administered dose. Thomson (1974) and Thomson et al (1977) observed absorption rates of 90-95%. Frankenberger and Benson (1994) described a somewhat lower absorption rate ( 50%) for sodium selenite administered to humans as food fortificant. Overall, it may be assumed that oral absorption of selenites is more or less complete (>>80%).
Three major routes of Se-excretion have been observed: via urine, feces, and expired air (Griffiths et al. 1976; Hawkes et al. 1992, 1994; McConnell and Roth 1966; Thomson and Stewart 1974), and the initial rate of excretion appears to be dose dependent (McConnell and Roth 1966; Thomson and Stewart 1974). Decreasing these urinary or fecal excretions appears to be the homeostatic mechanism by which the body maintains sufficiently high internal concentration levels of selenium (e.g. Martin et al., 1989).
Physiologically Based Pharmacokinetic (PBPK)/Pharmacodynamic (PD) models have been developed for selenite (Patterson et al, 1989) and selenomethionine (Swanson et al, 1991), but not for selenate.
Toxicity of Se – mode of action
The exact molecular mechanism of toxic action by selenium and selenium compounds is not known, but several theories and reliable hypotheses have been discussed in scientific literature. One theory is that at a biochemical level, selenium inactivates sulfhydryl enzymes leading to depression of cellular oxidative processes (Lombeck et al. 1987; Shamberger 1981). No information was located on established therapies designed to interfere with this possible mechanism of action of selenium. Halverson et al (1962) did observe that inorganic sulfate fed simultaneously with selenite or selenate in the diet protected rats from the toxicity of selenium as measured by body weight gain. The added sulfate, however, did not protect against liver necrosis caused by selenium.
The target organs and adverse health effects are generally similar across species. The liver appears to be the primary target organ for the oral toxicity of selenium in animals following intermediate and chronic exposure (Baker et al. 1989; BioulacSage et al. 1992; Fitzhugh et al. 1944; Halverson et al. 1969; Harr et al. 1967; Hasegawa et al. 1994; Kolodziejczyk et al. 2000; Nelson et al. 1943; Palmer and Olson 1974; Sayato et al. 1993; Schroeder and Mitchener 1972; Skowerski et al. 1997; Turan et al. 1999), whereas liver cirrhosis or dysfunction have not been found in reports of chronic selenosis in humans (Longnecker et al. 1991).
Conclusion
Until now, specific studies that focused on the selenate metabolism in mammals are limited. Based on the available information, however, it has been concluded that all inorganic Se-compounds are metabolised to selenide, which is then incorporated in various types of organic molecules (e.g. proteins, enzymes).
Key value for chemical safety assessment
Additional information
Information on Registered Substances comes from registration dossiers which have been assigned a registration number. The assignment of a registration number does however not guarantee that the information in the dossier is correct or that the dossier is compliant with Regulation (EC) No 1907/2006 (the REACH Regulation). This information has not been reviewed or verified by the Agency or any other authority. The content is subject to change without prior notice.
Reproduction or further distribution of this information may be subject to copyright protection. Use of the information without obtaining the permission from the owner(s) of the respective information might violate the rights of the owner.
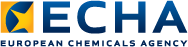