Registration Dossier
Registration Dossier
Data platform availability banner - registered substances factsheets
Please be aware that this old REACH registration data factsheet is no longer maintained; it remains frozen as of 19th May 2023.
The new ECHA CHEM database has been released by ECHA, and it now contains all REACH registration data. There are more details on the transition of ECHA's published data to ECHA CHEM here.
Diss Factsheets
Use of this information is subject to copyright laws and may require the permission of the owner of the information, as described in the ECHA Legal Notice.
EC number: 257-098-5 | CAS number: 51274-00-1 This substance is identified in the Colour Index by Colour Index Constitution Number, C.I. 77492.
- Life Cycle description
- Uses advised against
- Endpoint summary
- Appearance / physical state / colour
- Melting point / freezing point
- Boiling point
- Density
- Particle size distribution (Granulometry)
- Vapour pressure
- Partition coefficient
- Water solubility
- Solubility in organic solvents / fat solubility
- Surface tension
- Flash point
- Auto flammability
- Flammability
- Explosiveness
- Oxidising properties
- Oxidation reduction potential
- Stability in organic solvents and identity of relevant degradation products
- Storage stability and reactivity towards container material
- Stability: thermal, sunlight, metals
- pH
- Dissociation constant
- Viscosity
- Additional physico-chemical information
- Additional physico-chemical properties of nanomaterials
- Nanomaterial agglomeration / aggregation
- Nanomaterial crystalline phase
- Nanomaterial crystallite and grain size
- Nanomaterial aspect ratio / shape
- Nanomaterial specific surface area
- Nanomaterial Zeta potential
- Nanomaterial surface chemistry
- Nanomaterial dustiness
- Nanomaterial porosity
- Nanomaterial pour density
- Nanomaterial photocatalytic activity
- Nanomaterial radical formation potential
- Nanomaterial catalytic activity
- Endpoint summary
- Stability
- Biodegradation
- Bioaccumulation
- Transport and distribution
- Environmental data
- Additional information on environmental fate and behaviour
- Ecotoxicological Summary
- Aquatic toxicity
- Endpoint summary
- Short-term toxicity to fish
- Long-term toxicity to fish
- Short-term toxicity to aquatic invertebrates
- Long-term toxicity to aquatic invertebrates
- Toxicity to aquatic algae and cyanobacteria
- Toxicity to aquatic plants other than algae
- Toxicity to microorganisms
- Endocrine disrupter testing in aquatic vertebrates – in vivo
- Toxicity to other aquatic organisms
- Sediment toxicity
- Terrestrial toxicity
- Biological effects monitoring
- Biotransformation and kinetics
- Additional ecotoxological information
- Toxicological Summary
- Toxicokinetics, metabolism and distribution
- Acute Toxicity
- Irritation / corrosion
- Sensitisation
- Repeated dose toxicity
- Genetic toxicity
- Carcinogenicity
- Toxicity to reproduction
- Specific investigations
- Exposure related observations in humans
- Toxic effects on livestock and pets
- Additional toxicological data

Endpoint summary
Administrative data
Description of key information
Solubility data indicate that iron hydroxide oxide yellow is poorly soluble (< 2 µg/L). Furthermore, iron is relatively immobile under most environmental conditions, mainly due to the very low solubility of iron (III) hydroxide in its various forms. Thus, the rate and extent to which iron hydroxide oxide yellow produces soluble (bio)available ionic and other iron-bearing species in environmental media is limited. Hence, dissolution is not considered to be an important process for iron hydroxide oxide yellow in the environment. Further, the poor solubility of iron hydroxide oxide yellow is expected to determine its behaviour and fate in the environment.
Regarding the partitioning of iron in the environment, the median total iron content of European stream sediment expressed as Fe (XRF analysis) is 2.50% ranging from 0.08 to 12.80% whereas iron concentrations of the < 45 µm fraction of European stream waters are highly variable ranging from < 5 to > 3600 μg/L with a median of 67 µg/L (Salminen et al. 2005). A similarly high potential to partition into the sediment (or other solid phases) may be assumed for the poorly soluble iron hydroxide oxide yellow.
Biodegradation is not relevant for metals and metal compounds that are not biodegradable, including iron hydroxide oxide yellow.
For iron an essential, homeostatically controlled element, the bioaccumulation potential is considered to be low. A similarly low potential is assumed for the poorly soluble iron hydroxide oxide yellow.
The dispersion stability of nano-alpha FeOOH and nano-gamma FeOOH was screened in studies performed similar to the OECD TG 318. The stability of alpha FeOOH and gamma-FeOOH nanoparticles in dispersion after 6 h highly varied depending on the composition of the medium, i.e. nano-alpha FeOOH and nano-gamma FeOOH can be both considered of condition-dependent intermediate dispersion stability (10% < x < 90%) according to OECD 318. However, after 72 h, the dispersion stability of nano-alpha FeOOH further decreased to < 5% of test item in solution in almost all conditions investigated, except at low ionic strength – high pH conditions (0 mM Ca(NO3)2, pH 9), indicating low dispersion stability of nano-alpha FeOOH after 72 h. Similarly, after 72 h, the dispersion stability of nano-gamma FeOOH further decreased to < 5% of test item in solution in almost all conditions investigated, except at low ionic strength – neutral to high pH conditions (0 mM Ca(NO3)2, pH 7 and 9), indicating low dispersion stability of nano-gamma FeOOH after 72 h.
The main environmental processes determining the environmental fate of iron hydroxide oxide yellow separated in four categories are of different importance (see Table).
Table: Importance of environmental processes
| Environmental process | Low | Medium | High |
Chemical processes | Solubility/dissolution | x | - | - |
Physical processes | Aggregation/Agglomeration | - | - | x |
| Sedimentation | - | - | x |
Adsorption/desorption | Soil retention | - | - | x |
| Retention in sewage treatment plants | - | - | x |
Biologically mediated processes | Biodegradation | Not relevant | - | - |
Additional information
Environmental solubility
Poor water solubility of iron hydroxide oxide yellow was determined at a loading of 10 g/L at pH 8 (Daphnia medium, ISO 6341) with dissolved iron concentrations being < 1 µg/L (OECD TG 105; Prüm, 2009). A poor solubility (fraction < 3 kDa) without any increase in dissolved Fe concentrations was observed for iron hydroxide oxide yellow also in water over 25 days with 1.02 μg/L for Sicovite yellow 10 E172® 212P E172 (SSA: 18.6 m²/g; D50: 50 nm) (OECD TG 105; Klawonn, 2018).
Ubiquitousness and environmental chemistry of iron
Iron is the fourth most abundant element with a crustal average of 7%. It has lithophile and chalcophile properties, forming several common minerals, including pyrite FeS2, magnetite Fe3O4, haematite Fe2O3and siderite FeCO3. It is present in many rock-forming minerals. Secondary hydrous oxides are the dominant Fe phases of sedimentary rocks although primary oxides may account for some of the iron.
Iron has two main oxidation states (2+ and 3+). Iron is relatively immobile under most environmental conditions, mainly due to the very low solubility of iron (III) hydroxide in its various forms. Its solubility is strongly influenced by redox conditions. The Fe2+ion is more soluble in strong acid or reducing conditions. However, dissolved Fe precipitates rapidly with increasing pH or Eh and forms hydrous oxide (coatings on particles) in aerobic environments (Salminen et al. 2005).
Iron speciation in the simple system Fe-O-H without (left) and with (right) the effect of sulfur are presented in the attached Figure (Eh-pH diagrams for F-O-H and fe-S-O-H systems.pdf) . Hematite (Fe2O3) is shown as the stable Fe(III) species, since Fe(OH)3 and FeO·OH will eventually age to Fe2O3 although the kinetics for this aging may be very slow.
Ferrous iron (Fe2+) is reasonably soluble at neutral pH in anoxic environments, but in the presence of oxygen aqueous Fe2+is rapidly converted to relatively insoluble ferric (Fe3+) oxide-hydroxide. Ferric iron (Fe3+) is almost insoluble at neutral pH but can be solubilized by acidification (< pH 3).
Significant levels of H2S and CO2 in solution influence the pH-Eh conditions for mineral stability, decreasing the solubility of Fe under more reducing conditions particularly at near-neutral pH. The complexation with chloride, fluoride, nitrate, phosphate, sulfate and natural organic materials further affects dissolved Fe concentrations of stream water. The median total iron content of European soils expressed as Fe (XRF analysis) is 2.45% ranging from 0.11 to 15.60% in topsoil. The median total iron content of European stream sediment expressed as Fe (XRF analysis) is 2.50% ranging from 0.08 to 12.80% whereas iron concentrations of the < 45 µm fraction of European stream waters are highly variable ranging from < 5 to > 3600 μg/L with a median of 67 µg/L (Salminen et al. 2005).
Iron essentiality
Iron is essential for almost all living organisms as it is involved in a wide variety of important metabolic processes including oxygen and electron transport, gas sensing and DNA repair and replication and regulation of gene expression. Thus, iron is critical to the survival of living organisms, including plants, bacteria, animals and humans, to transport oxygen through the haemoglobin in animals and humans and to produce energy through electron transfer in the mitochondrial respiratory chain. Iron is a major constituent of the cell redox systems such as haeme proteins (e.g. cytochromes, catalase, peroxidase, leghaemoglobin) and iron sulfur proteins (e.g. ferredoxin, superoxide dismutase).
Due to its poor solubility under environmentally relevant conditions, iron is not readily available, and organisms have developed sophisticated pathways to import, chaperone, sequester, and export iron. Microorganisms, for example, employ various iron uptake systems, and there is considerable variation in the range of iron transporters and iron sources utilised by different microbial species. Iron as essential element for all plants has many important biological roles in biochemical processes including photosynthesis, chloroplast development and chlorophyll biosynthesis. Also, vertebrates have high requirements for iron, the majority of which is used by red blood cells for hemoglobin production.
Bioaccumulation
The existence of saturable uptake mechanisms, the presence of significant amounts of stored metal in organisms, and the ability of some organisms to regulate bioaccumulated metal within certain ranges are all thought to be responsible for the inverse relationship that has been frequently reported between bioaccumulation factors (BAFs) and metal exposure concentrations. In these cases, higher BAFs are associated with lower exposure concentrations and also can be associated with lower tissue concentrations within a given BAF study. This is contrary to the implicit assumption that higher BAFs indicate higher metal hazard. Nearly all metals, including iron, have BAFs >1000 in natural, healthy ecosystems with aqueous iron concentrations at background. Bioaccumulation factors for metals are clearly inversely related to water, sediment and soil concentrations (Adams, 2011).
For iron an essential, homeostatically controlled element, the bioaccumulation potential is considered to be low. Differences in iron uptake rates are related to essential needs, varying with the species, size, life stage, seasons etc. Iron homeostatic mechanisms are applicable across species with specific processes being active depending on the species, life stages. The available evidence shows the absence of iron biomagnification across the trophic chain in aquatic and terrestrial food chains. The existing information suggests that iron does not biomagnify, but rather that it tends to exhibit biodilution. Differences in sensitivity among species are not related to the level in the trophic chain but to the capability of internal homeostasis and detoxification (Vangheluwe & van Nederkassel, 2010).
References:
Adams B, 2011. Bioaccumulation of metal substances by aquatic organisms, OECD meeting, Paris September 7-8, 2011.
Salminen R et al. 2005. Geochemical Atlas of Europe. Part 1: Background Information, Methodology and Maps.http://weppi.gtk.fi/publ/foregsatlas/index.php.
Vangheluwe M & van Nederkassel J, 2010.White Paper on waiving for secondary poisoning for Fe and Al compounds. Final unpublished report February 2010.
Information on Registered Substances comes from registration dossiers which have been assigned a registration number. The assignment of a registration number does however not guarantee that the information in the dossier is correct or that the dossier is compliant with Regulation (EC) No 1907/2006 (the REACH Regulation). This information has not been reviewed or verified by the Agency or any other authority. The content is subject to change without prior notice.
Reproduction or further distribution of this information may be subject to copyright protection. Use of the information without obtaining the permission from the owner(s) of the respective information might violate the rights of the owner.
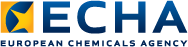