Registration Dossier
Registration Dossier
Data platform availability banner - registered substances factsheets
Please be aware that this old REACH registration data factsheet is no longer maintained; it remains frozen as of 19th May 2023.
The new ECHA CHEM database has been released by ECHA, and it now contains all REACH registration data. There are more details on the transition of ECHA's published data to ECHA CHEM here.
Diss Factsheets
Use of this information is subject to copyright laws and may require the permission of the owner of the information, as described in the ECHA Legal Notice.
EC number: 231-887-4 | CAS number: 7775-09-9
- Life Cycle description
- Uses advised against
- Endpoint summary
- Appearance / physical state / colour
- Melting point / freezing point
- Boiling point
- Density
- Particle size distribution (Granulometry)
- Vapour pressure
- Partition coefficient
- Water solubility
- Solubility in organic solvents / fat solubility
- Surface tension
- Flash point
- Auto flammability
- Flammability
- Explosiveness
- Oxidising properties
- Oxidation reduction potential
- Stability in organic solvents and identity of relevant degradation products
- Storage stability and reactivity towards container material
- Stability: thermal, sunlight, metals
- pH
- Dissociation constant
- Viscosity
- Additional physico-chemical information
- Additional physico-chemical properties of nanomaterials
- Nanomaterial agglomeration / aggregation
- Nanomaterial crystalline phase
- Nanomaterial crystallite and grain size
- Nanomaterial aspect ratio / shape
- Nanomaterial specific surface area
- Nanomaterial Zeta potential
- Nanomaterial surface chemistry
- Nanomaterial dustiness
- Nanomaterial porosity
- Nanomaterial pour density
- Nanomaterial photocatalytic activity
- Nanomaterial radical formation potential
- Nanomaterial catalytic activity
- Endpoint summary
- Stability
- Biodegradation
- Bioaccumulation
- Transport and distribution
- Environmental data
- Additional information on environmental fate and behaviour
- Ecotoxicological Summary
- Aquatic toxicity
- Endpoint summary
- Short-term toxicity to fish
- Long-term toxicity to fish
- Short-term toxicity to aquatic invertebrates
- Long-term toxicity to aquatic invertebrates
- Toxicity to aquatic algae and cyanobacteria
- Toxicity to aquatic plants other than algae
- Toxicity to microorganisms
- Endocrine disrupter testing in aquatic vertebrates – in vivo
- Toxicity to other aquatic organisms
- Sediment toxicity
- Terrestrial toxicity
- Biological effects monitoring
- Biotransformation and kinetics
- Additional ecotoxological information
- Toxicological Summary
- Toxicokinetics, metabolism and distribution
- Acute Toxicity
- Irritation / corrosion
- Sensitisation
- Repeated dose toxicity
- Genetic toxicity
- Carcinogenicity
- Toxicity to reproduction
- Specific investigations
- Exposure related observations in humans
- Toxic effects on livestock and pets
- Additional toxicological data

Biodegradation in soil
Administrative data
Link to relevant study record(s)
- Endpoint:
- biodegradation in soil: simulation testing
- Type of information:
- experimental study
- Adequacy of study:
- key study
- Study period:
- 2004-03-04
- Reliability:
- 1 (reliable without restriction)
- Rationale for reliability incl. deficiencies:
- other: Study generated according to generally valid and internationally accepted testing guideline and performed under GLP.
- Principles of method if other than guideline:
- Method: other: OECD proposal for a new guideline 307 "Aerobic and anaerobic transformation in soil" and SETAC Europe (1995) Procedures for assessing the environmental fate and ecotoxicity of pesticides
- GLP compliance:
- yes
- Test type:
- laboratory
- Radiolabelling:
- no
- Oxygen conditions:
- aerobic/anaerobic
- Soil classification:
- other: Landwirtschaftliche Untersuchungs- und Forschungsanstalt Speyer, Obere Langgasse 40, 67346 Speyer, Germany.
- Details on soil characteristics:
- - Soil type: Loamy sand, loam, sandy loam and clay
- Supplier: Landwirtschaftliche Untersugungs-und Forschungsanhalt Speyer, Speyer, Germany
- Characteristics of the soils: Loamy sand Loam Sandy loam Clay
Organic carbon (%): 2.3±0.2 2.6±0.7 1.6±0.0 2.0±0.4
pH (0.01 M CaCl2): 5.6±0.4 7.1±0.0 7.0±0.1 6.8±0.1
CEC (mval/100 g): 11±2 19±5 13±1 18±1
Particle sizes
(USDA) (%)
< 0.002 mm 8.1±1.2 16.9±0.1 9.4±2.3 41.0±1.9
0.002-0.05 mm 16.6±1.4 35.9±2.2 29.8±1.1 36.3±2.5
0.05-2.0 mm 75.3±2.0 47.3±2.3 60.9±1.2 22.8±0.6
Microb. biomass t=0
(mg bio-C/g soil) 0.32 0.36 0.23 0.28
Microb. biomass
t=120 (mg bio-C/g) 0.22 0.33 0.15 0.18
- Preconditioning: The soils were incubated for one week at 20 °C at 30% of their water holding capacity.
- In the bottles aerobic conditions in the soils were maintained thorough diffusion. Anaerobic conditions were established in one soil (loam). Aerobic conditions were maintained for 28 days. After this period the loam was water logged (1-3 cm water layer). In addition the oxygen from the gas
phase of the bottle was removed through flushing the gas phase with nitrogen gas.
- Soil temperature: 20 ± 1 °C
- Soil humidity: 50% of their water holding capacity
- Remark: Because chlorate is an oxidizing agent, reducing conditions will not occur until chlorate has disappeared. Monitoring the redox potential was therefore not carried out. - Duration:
- 148 d
- Initial conc.:
- 0.173 - 0.19 g/kg soil d.w.
- Based on:
- test mat.
- Parameter followed for biodegradation estimation:
- test mat. analysis
- Details on experimental conditions:
- Culturing apparatus: Bottles closed with septa. Side tubes were not required because carbon dioxide was not used as a measure to assess the
degradation of chlorate.
Number of culture flasks per concentration: 2 replicates
Measuring equipment: ion chromatograph
METHOD OF PREPARATION OF TEST SOLUTION: the test substance was added to the soils using solutions of 2.46 g/L (loamy sand), 2.56 g/L (loam), 2.80 g/L (sandy loam) and 2.67 g/L (clay). Total 200 g soil per bottle.
Addition of the stock solutions also resulted in water contents of 50% of their water holding capacity.
DURATION OF THE TEST: 148 days
SAMPLING: Small samples (10g) of aerobic soil were withdrawn for analyses of chlorate (four soils), chlorite (loam) and chloride (loam) at day 0, 7, 14, 28, 42, 56, 84 and 120. After 120 days 100 mg of sodium acetate was added per flask to provide a reducing power. The chlorate reduction in the
presence of the electron donor was measured at day 134 and 148.
The following time intervals were adopted for the anaerobic bottles starting after introduction of the anaerobic conditions: day 0, 4, 7, 10, 14, 21, 28, 35, 42, 56, 84 and 120.
Route of degradation: Sodium chlorite, with a purity of 80% and sodium chloride, with a purity of >99% were used. - DT50:
- 52 d
- Type:
- (pseudo-)first order (= half-life)
- Remarks on result:
- other: loamy sand, no or very low concentrations of nitrate
- DT50:
- 39 d
- Type:
- (pseudo-)first order (= half-life)
- Remarks on result:
- other: loam, no or very low concentrations of nitrate
- DT50:
- 58 d
- Type:
- (pseudo-)first order (= half-life)
- Remarks on result:
- other: sandy loam, no or very low concentrations of nitrate
- DT50:
- 47 d
- Type:
- (pseudo-)first order (= half-life)
- Remarks on result:
- other: clay, no or very low concentrations of nitrate
- DT50:
- 7.5 d
- Type:
- (pseudo-)first order (= half-life)
- Remarks on result:
- other: loam (anaerobic); no or very low concentrations of nitrate
- Transformation products:
- yes
- No.:
- #1
- Details on transformation products:
- chlorate was stoichiometric converted into chloride
- Evaporation of parent compound:
- no
- Volatile metabolites:
- no
- Details on results:
- Initial concentration: loamy sand 0.175 mg chlorate/g soil, 0.182 mg chlorate/g soil in loam, 0.174 mg chlorate/g soil in sandy loam and
0.166 mg chlorate/g soil in clay.
Significant degradation of chlorate was noted during the first four weeks under aerobic conditions. However, significant nitrate formation occurred
in this system which would competitively inhibit chlorate reduction and although no quantitative analysis of nitrate was performed, this was probablyresponsible for the levelling off of the chlorate degradation.
The soil was water logged from day 28 to the end of the test. Under these conditions chlorate reduction did not resume immediately but
recommenced after the nitrate was significantly reduced. In water logged loam in the absence of nitrate, remainder of the chlorate was reduced withinapproximately 6 weeks.
The time for 50, 75 and 90% dissipation under aerobic conditions can not be estimated because after approximately four weeks the reduction of
chlorate in all soils decreased significantly due to an artefact of the study (increase of nitrate concentration in the test soils). However, kinetics
results and initial concentrations of chlorate in soils may be determined using data from the first weeks (from day 0 to 28) of the test period.
In soils, the aerobic degradation of chlorate appears to follow first order kinetics during the first weeks.
Dissipation time:
DT50 (loamy sand) = 52 days
DT50 (loam) = 39 days
DT50 (sandy loam) = 58 days
DT50 (clay) = 47 days
DT50 (loam, anaerobic) = 7.5 days
Remark: These half-lives are only valid in soils with "environmentally realistic" nitrate concentrations.
Dissipation: Chlorate concentration (mg/g) (average of duplicates)
Time (d) Loamy sand Loam Sandy loam Clay
0 0.194 0.190 0.173 0.177
7 0.157 0.171 0.168 0.157
14 0.140 0.148 0.153 0.125
21 0.142 0.142 0.139 0.133
28 0.128 0.111 0.126 0.115
32
35
39
42 0.133 0.094 0.125 0.112
49
56 0.125 0.079 0.125 0.104
63
70
84 0.114 0.077 0.115 0.096
112
120 0.120 0.069 0.112 0.091
134 0.125 0.076 0.122 0.104
148 0.131 0.080 0.127 0.107
Loam (water logged from day 28) (average of duplicates)
Time (d) Chlorate conc. (mg/g) Chloride conc. (mg/g)
0 0.198 0.006
7 0.159 0.017
14 0.119 0.037
21 0.119 0.031
28 0.108 0.031
32 0.111 0.035
35 0.113 0.036
39 0.116 0.035
42 0.116 0.043
49 0.119 0.043
56 0.095 0.056
63 0.065 0.073
70 0.032 0.091
84 0.008 0.115
112 0.000 0.100
Repeatability of the analytical method (measured chlorate concentrations):
- Loamy sand: 4 samples between 0.190 and 0.196 mg chlorate/g (1 outlyer: 0.164 mg chlorate/g)
- Loam: 5 samples between 0.187 and 0.191 mg chlorate/g
- Sandy loam: 5 samples between 0.165 and 0.176 mg chlorate/g
- Clay: 5 samples between 0.172 and 0.185 mg chlorate/g
Mass balance for loam:
Time (d) Chlorate conc. Chloride conc. Sum (umol/g)
(umol/g) (umol/g)
0 2.274 0.187 2.461
7 2.054 0.314 2.368
14 1.776 0.472 2.248
21 1.700 1.179 2.879
28 1.331 0.866 2.197
42 1.132 0.991 2.123
56 0.943 1.048 1.991
84 0.918 1.306 2.224
120 0.833 1.530 2.363
134 0.906 1.500 2.406
148 0.953 1.599 2.552 - Conclusions:
- The result indicates that the lowest chlorate reduction
occurs in soils with the lowest initial biomass and the
lowest organic matter. Formation of nitrate was probably responsible for the leveling off of the chlorate
degradation. In water-logged loam in the absence of nitrate , remainder of chlorate was reduced within approximately 6 weeks. - Executive summary:
The biotic degradation (route and rate) of chlorate in four soils was assessed according to slightly modified OECD guidelines for testing of chemicals (proposal for a new guideline 307) and Procedures for assessing the environmental fate and ecotoxicity of pesticides (SETAC, 1995) and in compliance with the OECD principles of Good Laboratory Practice. Four soils were used for this investigation i.e. loamy sand, loam, sandy loam, and clay. The chosen soil types correspond to soil types required in the OECD guideline 307. The reduction of chlorate was determined by measuring the chlorate depletion. No lag periods were observed for chlorate degradation in soils. The times required for 50, 75 and 90% degradation of chlorate (DT50, DT75, and DT90) in loamy sand, loam and sandy loam and clay can not be estimated because after approximately four weeks the reduction of chlorate in all soils was negligible. Accumulation of nitrate is probably responsible for the negligible chlorate reduction. In the presence of "high" levels of nitrate, chlorate in soils is not biodegradable or biodegraded at low rates. Half-lives were calculated using the data of the initial weeks of incubation period. These half-lives range from 39 to 58 days. These half-lives are only valid in soils with "environmentally realistic" nitrate concentrations. Chlorate is stoichiometrically converted to chloride. In water-logged loam in the absence of nitrate, the remainder of the chlorate was reduced within approximately 6 weeks. The half-life of chlorate in loam under anaerobic conditions was 7.5 days. The microbial viability was demonstrated by the biodegradation of D-glucose. The quality of the test was also shown by a mass balance of chlorine in loam demonstrating that all chlorate converted was recovered as chloride.
Reference
Description of key information
Key value for chemical safety assessment
Additional information
Assimilatory nitrate reductases
Assimilationis the conversion nitrate into ammonium for anabolic reactions. Nitrate is reduced for this purpose by enzymes to nitrite (assimilatory nitrate reductases), and then to ammonia. The assimilatory nitrate reductases are molybdenum-containing enzymes, which are widespread in bacteria, fungi, yeasts, and algae (Cambell, 2001; Inokuch et al, 2002; Joseph-Horne et al, 2001; Siverio, 2001).Nitrate and chlorate are structurally analogous to each other and may potentially be incorporated into the same enzyme active site, as is evidenced by various assimilatory nitrate reductasesof micro-organisms and plants. Chlorate reduction by assimilatory nitrate reductases has been detected in bacteria (Escherichia coli) using a mutant (Motohara et al, 1965). Balch (1987) experimented with36Cl chlorate as a tracer to study nitrate uptake. In Skeletonema costatum and Nitzschia closterium chlorate was transported into the cells. The ability to reduce chlorate in whole cells has been shown in Ankistrodesmus braunii and Chlorella fusca both algae (Rigano, 1970; Tromballa and Broda, 1971). Chlorate is also a substrate for assimilatory nitrate reductase of Chloralla vulgaris (Salmonson and Vennesland, 1972). The assimilatory nitrate reductases convert chlorate to a toxic product chlorite. These results demonstrate that there is a potential for chlorate reduction under aerobic conditions provided that organisms are present capable of utilizing nitrate as nitrogen source.
Dissimilatory nitrate reductases
Denitrification is a process by which bacteria convert nitrate to dinitrogen that is lost to the atmosphere. Denitrifying bacteria use nitrate instead of oxygen in the metabolic processes. Denitrification takes primarily place where oxygen is depleted and where there is ample organic matter to provide energy for bacteria. Two types of dissimilatory nitrate reductases have been found. One of them is coupled to a complete denitrifying pathway (membrane-bound nitrate reductases; nitrate reductase A), and the other is a periplasmic protein whose physiological role seems to be the dissipation of excess reducing power. Periplasmic nitrate reductases, responsible for denitrification under aerobic conditions are specific for nitrate and not capable of reducing chlorate (Berks et al, 1994; McEwan et al, 1987). Chlorate reduction in denitrifying bacteria is primarily due to membrane-bound nitrate reductase (nitrate reductase A) activity (Iobbi et al, 1987; Morpeth and Boxer, 1985). The reduction of nitrate and chlorate in cell-free extracts of nitrate-grown Bacillus cereus was investigated by Hackenthal (1965). Chlorate reduction rates in cell-free extract were approximately twice as high as the nitrate reduction rates. De Groot and Stouthamer (1969) found that Proteus mirabilis formed different reductases including a chlorate reductase (chlorate reductase C). Chlorate reductase purified from Proteus mirabilis could only use chlorate as a substrate (Oltman et al, 1976). It was found that chlorate reductase was produced constitutively while nitrate reductases were produced inductively. However, the chlorate reduction in cell-free extracts of nitrate-grown bacteria is primarily due to membrane-bound nitrate reductases (de Groot and Stouthamer, 1969).
Chlorite is produced from chlorate by denitrifying microorganisms (Quastel et al, 1925; Karki and Kaiser, 1979). It was found that the absorption spectrum of dissimilatory nitrate reductase obtained from Escherichia coli after oxidation by chlorate was different from that of normal oxidized cytochrome. It was assumed that this was due to the oxidative deformation of the haem by the reduction product chlorite (Itagaki et al, 1963). Chlorite formed by denitrifying bacteria is degraded through chemical reactions with reducing agents such as the protein of nitrate reductase. In conclusion, chlorate reduction associated with nitrate-respiring organisms is a cometabolic process. The rate of chlorate reduction by denitrifying bacteria is therefore directly linked to the rate of denitrification.
Growth linked biodegradation (anaerobic)
It is now well-known that bacteria have evolved that can grow by the anaerobic reductive dissimilation of chlorate into innocuous chloride. Bacteria capable of growing with chlorate as electron acceptor are widely spread nature. This has been shown by (per)chlorate reduction with various energy substrates with a number of enrichment cultures (Bryan and Rohlich 1954; van Ginkel et al, 1995; Logan, 1998). The ubiquity of (per)chlorate reducing microorganisms was also shown quantitatively by enumerating the (per)chlorate-reducing bacteria in very diverse environments, including soils, aquatic sediments, sludges, and lagoons. In all of the environments tested, the acetate-oxidizing (per)chlorate reducing bacteria represented a significant population, whose size ranged from 2.3 × 103to 2.4 × 106cells per g of sample (Coates et al, 1999; Wu et al, 2001). Existence of (per)chlorate respiring bacteria have also been demonstrated in marine waters (Logan et al, 2000)
(Per)chlorate reducing microorganisms are easily enriched and isolated from many environments. All of these organisms could grow anaerobically by coupling complete oxidation of reducing agents to reduction of chlorate at high rates (Table). Under fully aerobic conditions, chlorate is not reduced by capable bacteria. Nitrate can also interfere with chlorate reduction (Chaudhuri et al 2002).
Table Growth ratesof various cultures capable of reducing chlorate.
Culture |
Reducing agent |
Rate (h-1) |
Reference |
Azospira oryzoa GR-1 |
Acetate |
0.1 |
Rikken et al (1996) |
Dechloromonas Agita sp CKB |
|
0.28 |
Bruce et al (1999) |
Azospira sp KJ |
Acetate |
0.26 |
Logan et al (2001) |
Dechlorosomonas sp PDX |
Acetate |
0.21 |
Logan et al (2001) |
Dechlorosomonas sp PDX |
Lactate |
0.15 |
Logan et al (2001) |
PDA |
Acetate |
0.18 |
Logan et al (2001) |
PDB |
Actate |
0.21 |
Logan et al (2001) |
Mixed culture |
Acetate |
0.56 |
Logan et al (1998) |
Mixed culture |
glucose glutamate |
0.12 |
Logan et al (1998) |
Mixed culture |
Phenol |
0.04 |
Logan et al (1998) |
Azospira oryzae strain GR-1 (DSM 11199) isolated from activated sludge was the first bacterium studied in more detail (Rikken et al, 1996; Wolterink et al, 2005). When strain GR-1 was grown on acetate, the release of chloride was proportional to the disappearance of chlorate, showing that this compound was completely reduced. The oxidation of acetate is coupled to the reduction of chlorate, whereas chlorite reduction is not affected by the addition of acetate. Azospira oryzae strain GR-1 disproportionates chlorite into molecular oxygen and chloride. For chlorate reduction by Azospira oryzae strain GR-1 the following biodegradation pathway was formulated: ClO3- → ClO2-→ Cl-+ O2. The rapid dismutation of chlorite into chloride and molecular oxygen is the key reaction in the reduction of chlorate. All (per)chlorate-reducing bacteria isolated to date have the ability to dismutate chlorite (Coates and Achenbach, 2004). Complete reduction of chlorate into chloride and molecular oxygen is catalysed by two enzymes. Chlorate is reduced to chlorite by (per)chlorate reductase (EC 1.97.1.1) (Kengen et al, 1999). Chlorate respiration at high rates is made possible by the action of the second enzyme which reduces the toxic chlorite to chloride while producing molecular oxygen. This is mediated by chlorite dismutase (EC 1.13.11.49) (van Ginkel et al 1996; Stenklo et al 2001). Chlorite has never been found to accumulate in solution during bacterial respiration of (per)chlorate.
Soils
The high water solubility of chlorate may facilitate its transport in soils from aerobic to anaerobic environments. Microbial chlorate reduction by organisms capabable of growing therefore constitutes a significant sink for chlorate in soils, especially in view of the importance of denitrification and sulphate reduction. Disappearance of chlorate from soils has been known for more than a century (Aslander 1928; Karki and Kaiser, 1979).
In an attempt to simulate the fate of sodium chlorate used as a herbicide, biodegradation was determined in four soils under aerobic conditions over a period of 120 days using OECD TG 307 (van Ginkel and van der Togt, 2004). Anaerobic degradation was examined in one soil which was kept under aerobic conditions for 28 days and then the soil was water-logged for a period of 120 days. Significant biodegradation of chlorate was noted during the first four weeks under aerobic conditions. This reduction is assumed to take place in anaerobic niches of the soil comparable to nitrate reduction observed in aerobic soils. After four weeks the chlorate reduction leveled off. Nitrate build up detected with a qualitative analysis occurred in the aerobic test systems due nitrification of ammonium in the aerobic niches of the soils. Nitrate is a known inhibitor of chlorate reduction and considered responsible for the leveling off of the chlorate degradation. One soil was water-logged from day 28 to the end of the test. Under these conditions chlorate reduction did not resume immediately but recommenced after the nitrate concentration was significantly reduced. In water-logged loam in the absence of nitrate, remainder of the chlorate was reduced within approximately 6 weeks. The time for 50, 75 and 90% dissipation under aerobic conditions cannot be estimated because after approximately four weeks the reduction of chlorate in all soils decreased significantly due to an artifact of the set-up of the study (increased nitrate concentrations due to nitrification). Nonetheless, kinetic data of chlorate in soils may be determined using the depletion curve from day 0 to 28 of the test period. During this period, the biodegradation of chlorate appears to follow first order kinetics and the half-lives calculated range from 39 to 58 days. These results indicate that chlorate is easily reduced in soils.
Table Half-lives determined in four soils at "environmentally realistic" nitrate concentrations measured in the soils upon arrival.
Soil |
Aerobic/anaerobic |
DT50 (days) |
Loamy sand |
Aerobic |
55 |
Loam |
Aerobic |
39 |
Sandyloam |
Aerobic |
58 |
Clay |
Aerobic |
47 |
Loam |
Anaerobic |
7.5 |
The half-life of chlorate in loam under anaerobic conditions (water-logged soil) was only 7.5 days. This rate of biodegradation is approximately 5 to 8 times higher than rates found in aerobic soils. The rates are probably very conservative because of the high concentrations used are not representative for other uses of sodium chlorate. The establishment of the route of degradation was assessed in loam (van Ginkel and van der Togt, 2004). The stoichiometric release of chloride from chlorate confirms chlorate is entirely reduced without production of other chlorine containing compounds. Chlorite was not detected in the soil samples during the test period. The stoichiometric release of chloride from chlorate confirms findings with chlorate respiring microorganisms (see above).
Information on Registered Substances comes from registration dossiers which have been assigned a registration number. The assignment of a registration number does however not guarantee that the information in the dossier is correct or that the dossier is compliant with Regulation (EC) No 1907/2006 (the REACH Regulation). This information has not been reviewed or verified by the Agency or any other authority. The content is subject to change without prior notice.
Reproduction or further distribution of this information may be subject to copyright protection. Use of the information without obtaining the permission from the owner(s) of the respective information might violate the rights of the owner.
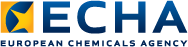