Registration Dossier
Registration Dossier
Data platform availability banner - registered substances factsheets
Please be aware that this old REACH registration data factsheet is no longer maintained; it remains frozen as of 19th May 2023.
The new ECHA CHEM database has been released by ECHA, and it now contains all REACH registration data. There are more details on the transition of ECHA's published data to ECHA CHEM here.
Diss Factsheets
Use of this information is subject to copyright laws and may require the permission of the owner of the information, as described in the ECHA Legal Notice.
EC number: 201-167-4 | CAS number: 79-01-6
- Life Cycle description
- Uses advised against
- Endpoint summary
- Appearance / physical state / colour
- Melting point / freezing point
- Boiling point
- Density
- Particle size distribution (Granulometry)
- Vapour pressure
- Partition coefficient
- Water solubility
- Solubility in organic solvents / fat solubility
- Surface tension
- Flash point
- Auto flammability
- Flammability
- Explosiveness
- Oxidising properties
- Oxidation reduction potential
- Stability in organic solvents and identity of relevant degradation products
- Storage stability and reactivity towards container material
- Stability: thermal, sunlight, metals
- pH
- Dissociation constant
- Viscosity
- Additional physico-chemical information
- Additional physico-chemical properties of nanomaterials
- Nanomaterial agglomeration / aggregation
- Nanomaterial crystalline phase
- Nanomaterial crystallite and grain size
- Nanomaterial aspect ratio / shape
- Nanomaterial specific surface area
- Nanomaterial Zeta potential
- Nanomaterial surface chemistry
- Nanomaterial dustiness
- Nanomaterial porosity
- Nanomaterial pour density
- Nanomaterial photocatalytic activity
- Nanomaterial radical formation potential
- Nanomaterial catalytic activity
- Endpoint summary
- Stability
- Biodegradation
- Bioaccumulation
- Transport and distribution
- Environmental data
- Additional information on environmental fate and behaviour
- Ecotoxicological Summary
- Aquatic toxicity
- Endpoint summary
- Short-term toxicity to fish
- Long-term toxicity to fish
- Short-term toxicity to aquatic invertebrates
- Long-term toxicity to aquatic invertebrates
- Toxicity to aquatic algae and cyanobacteria
- Toxicity to aquatic plants other than algae
- Toxicity to microorganisms
- Endocrine disrupter testing in aquatic vertebrates – in vivo
- Toxicity to other aquatic organisms
- Sediment toxicity
- Terrestrial toxicity
- Biological effects monitoring
- Biotransformation and kinetics
- Additional ecotoxological information
- Toxicological Summary
- Toxicokinetics, metabolism and distribution
- Acute Toxicity
- Irritation / corrosion
- Sensitisation
- Repeated dose toxicity
- Genetic toxicity
- Carcinogenicity
- Toxicity to reproduction
- Specific investigations
- Exposure related observations in humans
- Toxic effects on livestock and pets
- Additional toxicological data

Toxicological Summary
- Administrative data
- Workers - Hazard via inhalation route
- Workers - Hazard via dermal route
- Workers - Hazard for the eyes
- Additional information - workers
- General Population - Hazard via inhalation route
- General Population - Hazard via dermal route
- General Population - Hazard via oral route
- General Population - Hazard for the eyes
- Additional information - General Population
Administrative data
Workers - Hazard via inhalation route
Systemic effects
Long term exposure
- Hazard assessment conclusion:
- DNEL (Derived No Effect Level)
- Value:
- 54.7 mg/m³
- Most sensitive endpoint:
- repeated dose toxicity
- Route of original study:
- By inhalation
DNEL related information
- DNEL derivation method:
- other: SCOEL OEL
Acute/short term exposure
- Hazard assessment conclusion:
- DNEL (Derived No Effect Level)
- Value:
- 164.1 mg/m³
- Most sensitive endpoint:
- repeated dose toxicity
- Route of original study:
- By inhalation
DNEL related information
- DNEL derivation method:
- other: SCOEL STEL
Local effects
Long term exposure
- Hazard assessment conclusion:
- low hazard (no threshold derived)
Acute/short term exposure
- Hazard assessment conclusion:
- low hazard (no threshold derived)
DNEL related information
Workers - Hazard via dermal route
Systemic effects
Long term exposure
- Hazard assessment conclusion:
- DNEL (Derived No Effect Level)
- Value:
- 7.8 mg/kg bw/day
- Most sensitive endpoint:
- repeated dose toxicity
- Route of original study:
- By inhalation
DNEL related information
- DNEL derivation method:
- other: SCOEL OEL
- Explanation for the modification of the dose descriptor starting point:
- Inhalation DNEL converted to Dermal DNEL - see discussion below
Acute/short term exposure
- Hazard assessment conclusion:
- no hazard identified
DNEL related information
Local effects
Long term exposure
- Hazard assessment conclusion:
- no hazard identified
Acute/short term exposure
- Hazard assessment conclusion:
- no hazard identified
Workers - Hazard for the eyes
Local effects
- Hazard assessment conclusion:
- medium hazard (no threshold derived)
Additional information - workers
DNELs for local effects:
Eye irritation (due to classification with R36)
The substance is classified as an eye irritant. The available human and animal data do not allow a quantitative approach. According to the REACH guidance on information requirements and chemical safety assessment, Part E: Risk Characterisation, a qualitative risk characterisation should be performed for this endpoint. In order to guarantee ‘adequately control of risks’, it is necessary to stipulate risk management measures that prevent eye irritation, i.e. goggles are considered to be adequate risk management measures for worker protection.
Skin irritation (due to classification with R38)
Trichloroethylene is classified as a skin irritating substance (R38).However, it is not possible to derive a DNEL based on the available data. According to the REACH guidance on information requirements and chemical safety assessment, Part E: Risk Characterisation, a qualitative risk characterisation should be performed for this endpoint.In order to guarantee ‘adequately control of risks’, it is necessary to stipulate risk management measures that prevent dermal exposure that will cause skin irritation.
Skin sensitisation (proposed classification with R43)
Trichloroethylene is proposed to be classified as a skin sensitisation substance (R43).However, it is not possible to derive a DN(M)EL based on the available data. According to the REACH guidance on information requirements and chemical safety assessment, Part E: Risk Characterisation, a qualitative risk characterisation should be performed for this endpoint. In order to guarantee ‘adequately control of risks’, it is necessary to stipulate risk management measures that prevent dermal exposure that will cause skin sensitisation.
DNELS for systemic effects
Starting point for DNEL derivation
There is a significant toxicological database for trichloroethylene and several toxicological effects have been identified, including CNS effects, hepatotoxicity, nephrotoxicity, immunological effects and reproductive/developmental effects. With respect to the latter two findings, there is significant uncertainty surrounding both the nature and relevance of the findings. Notably, the recent EPA IRIS review considered that immunotoxicity and developmental cardiac effects were key effects for the derivation of a reference concentration. However their assessment and evaluation of the data for these endpoints has been widely criticised. An analysis of their assessment is provided here to demonstrate the issues with it and provide support for not considering these effects as key effects for the derivation of a DNEL.
Non-cancer RfC/RfD value based on rat cardiac malformation data from Dawson et al. (1993) and Johnson et al. (2003).
The studies from the University of Arizona investigators used unconventional and inappropriate and unusual testing methods to evaluate rat cardiac malformations, and both studies have been highly criticized in the peer reviewed literature (Hardin et al., 2004, 2005; Watson et al., 2006), in various comments submitted on behalf of industry to EPA (Kimmel et al, 2010, Appendix A), and also in recent EPA-sponsored peer review body analyses of these studies (Willhite, 2013, Appendix B). The significant concerns associated with the Johnson et al. studies are extensively detailed in these critiques (see Appendices).
The Dawson (1993) and Johnson (2003) studies, although originally described as two independent studies, in fact represented a pooling of control data from at least two studies separated by an unknown period of time potentially spread over several years of laboratory investigations. Such data pooling of the control groups (55 litters versus 9-13 litters for treated groups) is highly inappropriate for toxicity studies prone to potential changes in a variety of experimental variables including: different lots of TCE test material; change(s) in personnel, qualifications and level of expertise of investigators over the lengthy investigation time; modifications to procedures for examination fetal hearts and associated classification of lesions over time; and changes in sources of animal supply/husbandry that might influence chemical responses. Both the Dawson et al. (1993) and Johnson et al. (2003) papers did not provide adequate experimental detail to allow an assessment of the potential impact of these important variables on study outcome.
The fundamental methods for assessment of developmental toxicity responses of both papers were insufficiently detailed and severely flawed. For example, cardiac malformation incidence was calculated on a “per fetus” basis rather than the accepted “per litter” norm for such studies, and represents a critical analysis/reporting deficiency. Importantly, the lack of a detailed description of pup assignments to individual litters does not allow for an external reanalysis incorporating this standard development toxicity evaluation practice. Additionally, no information was provided on other ancillary health endpoints such as treatment effects in the mothers or non-cardiac effects in the fetuses, both of which are data necessary for meaningful and toxicologically defensible interpretation of developmental toxicity studies.
The Dawson/Johnson studies reported increased cardiac malformations over an exceptionally and unusually wide range of doses, and, importantly, the reported incidence of cardiac malformations over that range lacked evidence of a dose response relationship. There was a 440,000-fold difference between lowest tested, 2.5 ppb, and the top dose tested of 1,100,000 ppb (1100 ppm). Although the two lowest doses presented in Johnson et al. (2003), 2.5 and 250 ppb, were apparently unique to that study, both the 1.5 ppm (1500 ppb) and 1100 ppm (1,100,000 ppb) dose groups were apparently repeated from the earlier Dawson et al. study (1993) albeit with a significantly larger comparator control group due the methodologically inappropriate/questionable pooling of control data from multiple experiments conducted over an unknown period of time. In addition, the study authors later admitted refinements to the classification of cardiac malformations over the course of the investigations (Johnson et al., 2004). Despite the extreme differences in the range of test doses, fetal malformation rates differed only marginally (2.2, 0.0, 4.5, 5.0 and 10.5% for the 0, 2.5, 250, 1500 and 1,100,000 ppb treatment groups, respectively) and lacked a dose response (the 1,500 ppb was not statistically different from controls; also, the control cardiac malformation rate was reported as 3.0% in Dawson et al. vs 2.2% reported in Johnson et al. In addition, when TCE was administered only during pre-pregnancy (a test period not targeting fetal cardiac development), cardiac malformation incidence was reported as 6.6 and 6.5% for the 1,500 ppb and 1,100,000 ppb pre-pregnancy only treatments, respectively (Dawson et al., 1993), suggesting that inter-experimental variation in control cardiac lesion incidence maybe higher than indicated in Johnson et al. (2003). The extremely unusual dose-response, i.e., only a slightly varied response incidence separated by very large treatment dose differences, raises significant concerns whether the claimed TCE-induced increases in cardiac malformation incidence was truly treatment-related, and strongly suggests the response was more likely an artifact of the novel methodology used in the assessment of cardiac malformations.
Very important to weighting the relevance of the conclusions presented by Dawson and Johnson (1993, 2003) as a driver of a potential human health risk concern, however, is that the findings were not replicated in two high quality GLP developmental toxicity studies which included a specific focus on identifying potential TCE-related cardiac malformations. Using an oral gavage dosing protocol, Fisher et al., (2001) failed to find any evidence of TCE-induced cardiac malformations in rats despite using a top test dose that was approximately 4-fold higher than that used in the Johnson et al. study (500 mg/kg/day versus 129 mg/kg/day). And perhaps even more indicative of the likelihood of a methodological problem associated with cardiac lesion evaluations conducted in Dawson/Johnson, the lead investigator of these studies, Johnson, participated in conduct of fetal examinations in the Fisher et al. study (but, importantly, blinded to treatment).
The Dawson and Johnson findings also were not replicated in high dose rat developmental toxicity study conducted by inhalation and using a top exposure level far above the EPA IRIS RfC value (600 ppm, 6 hr/day during embryo-fetal developmental period, and which produced significant maternal toxicity; Carney et al., 2006). Thus, Carney et al. (2006) provides further high quality confirmation of an absence of cardiac malformations in rats by a second key route of exposure concern for TCE, inhalation.
It is also useful to note that the conclusion that TCE is not a developmental toxicant is consistent with a series of studies which also failed to find evidence of TCE-induced malformations (reviewed in Watson et al., 2006).
The significant deficiencies of the Dawson and Johnson studies make them both highly unreliable for use in development of health risk standards, and particularly so given the failure of higher quality and higher dose studies conducted by both the oral and inhalation routes to replicate the Dawson and Johnson findings. The far higher quality studies of Fisher et al (2001) and Carney et al. (2006) should be the primary animal data for evaluating human developmental toxicity, and both of these studies indicate TCE is not a developmental toxicant, and does not increase the incidence of cardiac malformations.
Non-cancer RfC/RFD based on developmental immunotoxicity in mice (Keil et al, 2009; Peden-Adams et al, 2006)
In addition to the Dawson and Johnson studies, EPA also relied on two other reports from another research laboratory as principal or supporting studies for estimation of the RfC/RfD. Those studies described immunotoxicity responses in either adult (Keil et al., 2009) or developing (Peden-Adams et al., 2006) mice treated with 1.4 and 14 ppm TCE in drinking water. These responses included a standard indicators of immune suppression, alteration splenic antibody response (PFC) to sheep red blood cells (Peden-Adams et al., 2006), and alteration of thymus weights (Keil et al., 2009). Thus, even absent the findings of Dawson/Johnson, EPA determined that these immunoxicity findings translated to calculated RfC/RfD values in close quantitative proximity to values based on cardiac malformations. However, as with the Johnson et al. study, the reliability of either of these findings to establish an RfD/RfC also is highly questionable for the reasons summarized below.
The decrease in adult thymus weights following treatment of B6C3F1 mice with either 1.4 or 14 ppm TCE for 27 to 30 weeks (Keil et al., 2009) was not replicated in an earlier developmental immunotoxicity study conducted by the same laboratory using the same strain of mice and a similar dosing regimen of 1.4 and 14 ppm TCE in drinking water administered continuously to dams and to the B6C3F1 mice offspring up to adulthood at 8 weeks of age (Peden-Adams et al., 2006). In addition, Keil et al. (2009) reported that TCE treatmentdecreasedthymic cellularity at the 14 ppm dose level while Peden-Adams et al. (2006) described an unexpectedly opposite effect ofincreasedthymic cellularity at the same dose level at 8 weeks of age. Although Peden-Adams (2006) reported other evidence of developmental immune suppression, a variety of experimental descriptors key to interpreting the risk relevance of these findings (parental toxicity, coincident toxicity, control of litter effects, positive control data) were not described (critiqued in Boverhof et al., 2013).
Interestingly, in a separate study examining the immunotoxicity of another environment contaminant, PFOS, in B6C3F1 mice, the Keil/Peden-Adams research team finding of decreased PFC response also was not replicated in a similar immunotoxicity evaluation using even higher PFOS dose levels (Qazi et al., 2010). The lack of replication of the PFOS findings from the Keil/Peden-Adams investigators further calls into the question the ability of the Keil/Peden-Adams group to reliably implement methods routinely used to detect chemically-induced immunotoxicity.
In addition to findings in mice, a recent inhalation immunotoxicity in adult rats (0, 100, 300, and 1000 ppm, 6 hour/day, 5 days/week, 4 weeks) found no treatment related effects on thymus weights, and identified a NOEL for TCE immunotoxicity of 300 ppm based on a reduced splenic PFC response seen at 1000 ppm (Boverhof et al., 2013). Even despite the differences in mode of administration and use of a different species, the dramatic difference in dose required to elicit immunotoxicity in adult rats compared to the very low dose inducing similar immunotoxicity in mice (Keil et al., 2009) further questions the potential health significance of those findings.
References for this analysis:
Boverhof DR, Krieger SM, Hotchkiss JA, Stebbins KE, Thomas J, Woolhiser MR. Assessment of the immunotoxicity potential of trichloroethylene and perchloroethylene in rats following inhalation exposure. J. Immunotoxicol. 10: 311-320, 2013.
Carney, E.W., B.A. Thorsrud, P.H. Dugard, and C.L. Zablotny. Developmental toxicity studies in Crl:CD (SD) rats following inhalation exposure to trichloroethylene and perchloroethylene. Birth Defects Research, Part B: Developmental and Reproductive Toxicology 77:405-412 (2006).
Dawson BV, Johnson PD, Goldberg SJ, Ulreich JB. Cardiac teratogenesis of halogenated hydrocarbon-contaminated drinking water. J Am Coll Cardiol. 21:1466-72 (1993).
Fisher JW, Channel SR, Eggers JS, Johnson PD, MacMahon KL, Goodyear CD, Sudberry GL, Warren DA, Latendresse JR, Graeter LJ. Trichloroethylene, trichloroacetic acid, and dichloroacetic acid: Do they affect fetal rat heart development? Int J Toxicol. 20:257-67 (2001).
Hardin BD, Kelman BJ, Brent RL. Trichloroethylene and cardiac malformations, a correspondence in Environ Health Perspect. 112:A607-8 (2004).
Hardin BD, Kelman BJ, Brent RL. Trichloroethylene and dichloroethylene: A critical review of teratogenicity. Birth Def Res (Pt A) 73: 931-955 (2005).
Halogenated Solvent Industry Alliance, Comments submitted by Paul Dugard, Toxicological Review of Trichloroethylene in support of the IRIS Database, 2009.
Johnson PD, Goldberg SJ, Mays MZ, Dawson BV. Threshold of trichloroethylene contamination in maternal drinking waters affecting fetal heart development in the rat. Environ Health Perspect. 111:289-92 (2003).
Keil DE, Peden-Adams MM, Wallace S, Ruiz P, Gilkeson GS. Assessment of trichloroethylene (TCE) exposure in murine strains genetically prone and non-prone to develop autoimmune disease. J Environ Sci Hlth.44: 443-453 (2009).
Kimmel CA, Kimmel GL, DeSesso JM. Comments on the public review draft of EPA’s toxicological review for TCE developmental effect. Submitted January 29, 2010.
Peden-Adams MM, Eudaly JG, LM, Smythe J, Miller J, Gilkeson GS, Keil DE. Development immunotoxicity of trichloroethylene (TCE): Studies in B6C3F1 mice. J Env Sci Hlth Part A: Toxic/hazardous substances and environmental engineering 41: 249-271 (2006).
Qazi MR, Nelson BD, DePierre JW, Abedi-Valvgerdi M. 28-Day dietary exposures of mice to a low dose (7 mg/kg) of perfluorooctane sulfonate (PFOS) alters neither the cellular compositions of the thymus and spleen nor humoral immune responses: Does the route of administration play a pivotal role in PFOS-induced immunotoxicity? Toxicol. 267: 132-139 (2010).
National Toxicology Program (NTP). Toxicology and carcinogenesis studies of trichloroethylene in four strains of rats (ACI, August, Marshall, Osborne-Mendel). Technical Report Series No. 273 (1988).
Watson RE, Jacobson CF, Williams, AL, Howard, WB, DeSesso JM. Trichloroethylene-contaminated drinking water and congenital heart defects. A critical analysis of the literature. Reprod Toxicol 21: 117-141 (2006).
Considering the issues surrounding the use of Immunotoxicity or developmental cardiac effects as key starting points for the derivation of a DNEL, the more reliable and robust endpoint of kidney toxicity has been selected as the starting point for DNEL derivation. This was the approach taken by SCOEL in 2009 when they derived an OEL for trichloroethylene. In deriving the OEL SCOEL concluded that the key endpoint of concern was kidney toxicity and kidney cancer.Although the exact mechanism of action remains to be clarified, it is considered that kidney cancer in humans is most likely secondary to kidney toxicity and that if there is a genotoxic element involved it is not the critical component to the mechanism. This is discussed in more detail in the review of the mode of action and also in the SCOEL assessment attached to the IUCLID dossier. This view is consistent with observations in experimental systems, as well as in occupationally exposed and diseased persons, where the risk of human renal cell cancer is minimised if exposure to nephrotoxic concentrations is avoided, including concentrations leading to sub-clinical renal changes that can be monitored by urinary excretion of suitable marker proteins. In their assessment SCOEL therefore concluded that although genotoxicity could potentially play a role in the carcinogenesis of trichloroethylene, there is still a practical threshold possible due to the need for concurrent kidney toxicity. As such, a threshold approach was adopted when deriving the OEL for trichloroethylene.
Since the SCOEL assessment there have not been any new data generated that would challenge this approach and as such, a threshold approach to setting an occupational exposure level is still considered to be applicable today.
The basis for the SCOEL OEL was the occupational studies performed by Green et al. (2004) and Selden et al. (1993). In the occupational field study by Greenet al(2004) which included 70 workers, the mean trichloroethylene exposure was 32 ppm (range 0.5-252 ppm). In this cohort some minor sub-clinical alterations in renal functional parameters were observed. This is corroborated by data of Seldenet al(1993), who found no increase in urinary excretion of the NAG marker protein in workers exposed to a range of 6 to 10 ppm trichloroethylene. The SCOEL OEL was therefore set to 10 ppm as a level that was protective of kidney toxicity and this was adopted as the 8hr TWA OEL. This is supported by the more recent study by Vermeulen et al (2012) who identified only minimal effects on urinary excretion of a more sensitive biomarker for kidney toxicity, KIM-1, in workers exposed to approximately 12 ppm TCE during their shift.
Worker Systemic, Long term Inhalation DNEL
In Appendix R.8-13 of the Guidance on information requirements and chemical safety assessment, Chapter R.8: Characterisation of dose [concentration]-response for human health (May 2008, ECHA) it is noted that:
‘When an EU IOEL exists the registrant may, under conditions as described below, use the IOEL in place of developing a DNEL. A registrant is allowed to use an IOEL as a DNEL for the same exposure route and duration, unless new scientific information that he has obtained in fulfilling his obligations under REACH does not support the use of the IOEL for this purpose. This could be because the information obtained is more recent than the information that was used to support setting the IOEL at EU level and because it leads to another value being derived which requires different risk management measures (RMMs) and operational conditions (OCs)’.
The SCOEL OEL of 10 ppm is therefore adopted as the DNEL; equivalent to 54.7 mg/m3
DNEL for acute exposure
SCOEL also derived a short term exposure limit (STEL) based on the average exposure level of 32ppm associated with evidence of kidney toxicity in the Green et al (2004) study. The STEL was set as 30 ppm. This STEL derived by the SCOEL will be used as DNEL for acute inhalation exposure: 30 ppm (164.1 mg/m3) for an exposure duration of 15 minutes.
DNEL for long-term exposure
Dermal exposure (route-to-route extrapolation):
To arrive at a long-term value for the dermal route for workers, route to route extrapolation will be applied starting with the inhalation long-term value of the SCOEL:
54.7 mg/m3* [100/100] (a) * 10 m3(b) / 70 kg (c) = 7.8 mg/kg bw/day
(a) correction for absorption, worst case of 100% via both routes;
(b) 8-hour respiratory volume for workers
(c) body weight for workers
No additional assessment factors are applied.
General Population - Hazard via inhalation route
Systemic effects
Long term exposure
- Hazard assessment conclusion:
- DNEL (Derived No Effect Level)
- Value:
- 13.7 mg/m³
Acute/short term exposure
- Hazard assessment conclusion:
- DNEL (Derived No Effect Level)
- Value:
- 82 mg/m³
DNEL related information
Local effects
Long term exposure
- Hazard assessment conclusion:
- low hazard (no threshold derived)
Acute/short term exposure
- Hazard assessment conclusion:
- DNEL (Derived No Effect Level)
- Value:
- 82 mg/m³
DNEL related information
General Population - Hazard via dermal route
Systemic effects
Long term exposure
- Hazard assessment conclusion:
- DNEL (Derived No Effect Level)
- Value:
- 4.6 mg/kg bw/day
Acute/short term exposure
- Hazard assessment conclusion:
- no hazard identified
DNEL related information
Local effects
Long term exposure
- Hazard assessment conclusion:
- no hazard identified
Acute/short term exposure
- Hazard assessment conclusion:
- no hazard identified
General Population - Hazard via oral route
Systemic effects
Long term exposure
- Hazard assessment conclusion:
- DNEL (Derived No Effect Level)
- Value:
- 0.9 mg/kg bw/day
Acute/short term exposure
- Hazard assessment conclusion:
- no hazard identified
DNEL related information
General Population - Hazard for the eyes
Local effects
- Hazard assessment conclusion:
- medium hazard (no threshold derived)
Additional information - General Population
There are no consumer uses for trichloroethylene and as such DNELs for the General population are not required. In addition, under the conditions of the supported uses it is not expected that there would be general population exposure through releases of the trichloroethylene to the environment.
However, irrespective of whether they are required or not, DNELs for the general population can be derived using the occupatioanl exposure guideline derived by SCOEL (see worker DNELs). This has been illustrated below.
General population-DNEL short-term for the inhalation route:
To arrive at a short-term value for the general population, a factor for intra-species differences will be applied to the 15 min STEL of the SCOEL (‘recovery’ to ‘no recovery’ is assumed):
164.1 mg/m3* 1/2 (a) = 82 mg/m3
(a) correction for intra-species differences: workers default factor: 5, general population default factor: 10
General population-DNEL long-term for inhalation route:
To arrive at a long-term value for the general population, a factor for intra-species differences and exposure modification will be applied to the 8-h TWA for workers (covering from ‘recovery’ to ‘no recovery’ is assumed):
54.7 mg/m3* 10/20 (a) * 1/2 (b) = 13.7 mg/m3
(a) modification based on differences in exposure and activity (10 m3in 8 h for workers, 20 m3in 24 h for the general population)
(b) correction for intra-species differences: workers default factor: 5, general population default factor: 10
General population-DNEL long-term for dermal route:
To arrive at a long-term value for the dermal route for the general population, route to route extrapolation will be applied starting with the inhalation long-term value calculated for the general population:
13.7 mg/m3* [100/100] (a) * 20 m3(b) / 60 kg (c) = 4.6 mg/kg bw/day
(a) correction for absorption;
(b) 24 hour respiratory volume for the general population (to calculate the internal dose corresponding to 24 hour exposure)
(c) body weight for the general population
General population-DNEL long-term for oral route:
To arrive at a long-term value for the oral route for the general population, the chronic oral study in rats was used, which has an NOAEL of 50 mg/kg bw/day:
50 mg/kg bw/day / [5/7] (a) / 4 b / 10 (d) / 60 kg (e) = 0.9 mg/kg bw/day
(a) correction for duration (from 5 to 7 days)
(b) correction for allometric factor (rat)
(c) correction for intra-species differences
(d) body weight for the general population
Information on Registered Substances comes from registration dossiers which have been assigned a registration number. The assignment of a registration number does however not guarantee that the information in the dossier is correct or that the dossier is compliant with Regulation (EC) No 1907/2006 (the REACH Regulation). This information has not been reviewed or verified by the Agency or any other authority. The content is subject to change without prior notice.
Reproduction or further distribution of this information may be subject to copyright protection. Use of the information without obtaining the permission from the owner(s) of the respective information might violate the rights of the owner.
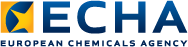