Registration Dossier
Registration Dossier
Data platform availability banner - registered substances factsheets
Please be aware that this old REACH registration data factsheet is no longer maintained; it remains frozen as of 19th May 2023.
The new ECHA CHEM database has been released by ECHA, and it now contains all REACH registration data. There are more details on the transition of ECHA's published data to ECHA CHEM here.
Diss Factsheets
Use of this information is subject to copyright laws and may require the permission of the owner of the information, as described in the ECHA Legal Notice.
EC number: 205-391-3 | CAS number: 140-01-2
- Life Cycle description
- Uses advised against
- Endpoint summary
- Appearance / physical state / colour
- Melting point / freezing point
- Boiling point
- Density
- Particle size distribution (Granulometry)
- Vapour pressure
- Partition coefficient
- Water solubility
- Solubility in organic solvents / fat solubility
- Surface tension
- Flash point
- Auto flammability
- Flammability
- Explosiveness
- Oxidising properties
- Oxidation reduction potential
- Stability in organic solvents and identity of relevant degradation products
- Storage stability and reactivity towards container material
- Stability: thermal, sunlight, metals
- pH
- Dissociation constant
- Viscosity
- Additional physico-chemical information
- Additional physico-chemical properties of nanomaterials
- Nanomaterial agglomeration / aggregation
- Nanomaterial crystalline phase
- Nanomaterial crystallite and grain size
- Nanomaterial aspect ratio / shape
- Nanomaterial specific surface area
- Nanomaterial Zeta potential
- Nanomaterial surface chemistry
- Nanomaterial dustiness
- Nanomaterial porosity
- Nanomaterial pour density
- Nanomaterial photocatalytic activity
- Nanomaterial radical formation potential
- Nanomaterial catalytic activity
- Endpoint summary
- Stability
- Biodegradation
- Bioaccumulation
- Transport and distribution
- Environmental data
- Additional information on environmental fate and behaviour
- Ecotoxicological Summary
- Aquatic toxicity
- Endpoint summary
- Short-term toxicity to fish
- Long-term toxicity to fish
- Short-term toxicity to aquatic invertebrates
- Long-term toxicity to aquatic invertebrates
- Toxicity to aquatic algae and cyanobacteria
- Toxicity to aquatic plants other than algae
- Toxicity to microorganisms
- Endocrine disrupter testing in aquatic vertebrates – in vivo
- Toxicity to other aquatic organisms
- Sediment toxicity
- Terrestrial toxicity
- Biological effects monitoring
- Biotransformation and kinetics
- Additional ecotoxological information
- Toxicological Summary
- Toxicokinetics, metabolism and distribution
- Acute Toxicity
- Irritation / corrosion
- Sensitisation
- Repeated dose toxicity
- Genetic toxicity
- Carcinogenicity
- Toxicity to reproduction
- Specific investigations
- Exposure related observations in humans
- Toxic effects on livestock and pets
- Additional toxicological data

Endpoint summary
Administrative data
Key value for chemical safety assessment
Effects on fertility
Additional information
DTPA is a chelating agent with a high affinity for metals such as Zinc, Manganese and Calcium (please refer to the section on toxicokinetics). Zinc is one of the most abundant metals in the human body (2-4g) and is present as a cofactor a large number of enzymes (between 100 and 300), covering almost all classes of enzyme. As such deficiencies in zinc can produce a wide array of symptoms including both reproductive and developmental toxicity. If a dietary multi generation reproductive toxicity study were to be performed with DTPA in the absence of any supplementation of essential minerals such as zinc, then it is highly likely that the DTPA would complex with enough of the zinc in the diet leading to an insufficient zinc intake in the animals. This would lead to evidence of male reproductive toxicity (specifically degeneration of the testicular tissue and reduced fertility), developmental toxicity such as terata of the skeletal and viscera and many othe symptoms of zinc deficiency such as alopecia, diarrhea, eye and skin lesions etc.. Such a study would therefore not provide evidence of the reproductive or developmental toxicity of DTPA but rather the toxicity associated with a deficiency in zinc.
In the 2 available 28 -day studies where DTPA salts were dosed via gavage or the drinking water there were some clinical signs of a perturbation in nutrition in the high doses (diarrhea, decreased food consumption, decrease in bodyweight), and in the gavage fed animals there were deaths in the high dose group males. It is very likely that the deaths were associated with diarrhea caused by chelation of metals such as zinc and calcium in the intestinal tract leading to decreased absorption of food and water loss. In these studies there was no evidence of testicular toxicity in any of the treated groups, indicating that the dosing regime was insufficient to produce sufficient zinc deficiency to lead to testicular toxicity. It is possible that a more prolonged dosing regime could have produced a more extensive deficiency in zinc, however studies conducted with a similar chelating agent, EDTA (ethylene diamine tetraacetic acid) also failed to produce any evidence of testicular degeneration following dosing via gavage or drinking water.
DTPA is poorly absorbed both orally and via dermal application and is unlikely to be absorbed significantly via inhalation due to its high particle size (>10 microns diameter) when in powdered form and low volatility when in solution. It is not metabolised and is excreted with a very short half life (2 hours) in humans and rats. With the exception of being able to complex metal ions, chelating agents are of low chemical reactivity as evidenced by their lack of genotoxicity and skin sensitising potential. As such, it is unlikely that the chelating agent itself is a proximate toxicant, but rather that its ability to bind metal ions is responsible for observed toxicity (as indicated above). Therefore if one were to conduct a study where metal ions (for example zinc) were supplemented sufficiently it is unlikely that any systemic toxicity, including developmental or reproductive effects would be observed. This is supported by developmental toxicity studies conducted using the zinc salt of DTPA where no developmental (or systemic) toxicity was observed in mice injected intraperitoneally at doses in excess of 1000 mg/kg bw/day (Brummett et al 1977), this is compared to the calcium salt of DTPA where the same doses caused significant increases in developmental and systemic toxicity in mice. Additional support comes from studies (and treatments) conducted in humans and animals where the zinc salt of DTPA was dosed with no evidence of systemic toxicity at therapeutic doses (Kalkwarf et al 1983; Sato 1993).
In conclusion, it is plausible that a standard dietary multigeneration study conducted with DTPA would identify evidence of reproductive and developmental toxicity. However such toxicity would be due to an induced deficiency in zinc and any reproductive or developmental effects would be observed only in the presence of, and secondary to parental toxicity. Therefore it is considered innapropriate to propose any further testing on DTPA since the likely outcome of the study can be predicted without using animals unnecessarily. Reproductive toxicity effects secondary to a zinc deficiency should not be considered relevant for classification if it can be demonstrated that occupational or consumer exposure to DTPA would not result in a deficit in an individuals zinc status.
As part of a classification and labelling justification prepared for developmental toxicity it has been demonstrated that exposure to DTPA through worker and consumer uses would be insufficient to produce a deficiency in zinc in the workforce or consumer population. Since reproductive toxicity would require a zinc deficiency to be induced as a first step in the toxicity it is very unlikely that this would occur.
Short description of key information:
28-day studies on DTPA (sodium and potassium salts). Read Across to EDTA
Effects on developmental toxicity
Description of key information
OECD 414 pre-natal developmental toxicity study in rats using PentasodiumDTPA
Effect on developmental toxicity: via oral route
- Dose descriptor:
- NOAEL
- 100 mg/kg bw/day
Additional information
-
Does DTPA induce Zn deficiency in the maternal organism?
-
Is Zn deficiency per se a trigger for embryo-/fetotoxicity?
-
What is the relevance of this mechanism for the effects observed with DTPA in the teratogenicity study?
-
DTPA is capable of forming stronger complexes with the essential element zinc than other metals such as calcium, sodium and potassium.
-
DTPA administered to both animals and man is capable of increasing zinc excretion and in some cases inducing a deficiency.
-
Administration of the zinc salt of DTPA does not cause increased zinc excretion and is less toxic than other forms of DTPA.
-
Oral administration of potassium DTPA produces effects consistent with those seen with other structurally related chelating agents; i.e.in vivoDTPA appears to act in a similar manner to those chelating agents.
In a standard OECD Guideline teratogenicity study DTPA administration caused developmental toxicity (BASF 1994). In this study the dams in the high dose group exhibited reduced food consumption between GD 6-10, reduced bodyweight at GD17 and GD20, and reduced bodyweight gain during GD6-8 and GD15-17 (p< 0.05). Overall, bodyweight gain during and after cessation of treatment was lower in this group than in controls. Faeces were discoloured (dark yellow) in this group during treatment, but reverted to normal during the post-treatment phase. There were no differences between the other treatment groups and the control group.Gravid uterus weights in the dams of the high dose group were lower than control, as were the live litter sizes and bodyweights of both male and female fetuses. There was also an 11.5% reduction in adjusted bodyweight gain of the dams, although this was not statistically significant. There were no differences between the other treatment groups and the control group.There were no treatment-related findings observed following external or visceral examination of the fetuses. There were, however, significant increases in select malformations and variations of the skeleton in the high-dose group, and select retardations in both the high- and mid-dose groups, compared to control incidences.
Skeletal malformations were manifested as missing thoracic and lumbar vertebrae and bipartite sternebrae. Variations noted were shortened or absent 13thrib and rudimentary cervical ribs. The only retardations found were incomplete ossification of the skull and sternebrae.Whilst these findings are a clear indication that DTPA is capable of producing terata at high oral doses, the relevance of this effect to man is questionable. It is considered probable that the developmental toxicity is occuring secondary to a maternal zinc deficiency, i.e. maternal toxicity. In order to reproduce this toxicity in a pregnant human, DTPA would have to be administered in such as way that the zinc status would be negatively impacted throughout pregnancy. This would require significant oral doses of DTPA (this is illustrated in the classification and labelling argument attached to section 13 of this IUCLID. The Support for the Hypothesis that DTPA produces a zinc deficiency by creating a zinc deficiency comes from an understanding of three key issues:
Does DTPA induce Zn deficiency in the maternal organism?
In the OECD guideline teratogenicity study on DTPA (BASF 1994) there was no assessment of zinc status in either the maternal or fetal organisms. Therefore from the results of this study alone it is not possible to conclusively state that the zinc status was affected by DTPA administration. However by considering the physical properties of DTPA and the results of other studies conducted with it or structurally similar chemicals there is enough evidence available to support the conclusion that DTPA administration is capable of altering zinc status. Therefore it is plausible that this occurred in the teratogenicity study in question.
DTPA and Zinc
DTPA is a chelating compound used both industrially and pharmacologically to bind metals. DTPA predominantly binds divalent metals including zinc, iron, manganese, calcium, sodium and potassium. Of these it binds most strongly to zinc (binding affinity Log K 18.3) compared to calcium (Log K 10.7) or potassium and sodium (Log K 0.9). Therefore in biological matrices where zinc is present, DTPA will have a strong tendency to dissociate from metals such as calcium, sodium or potassium in favour of binding zinc. Also, compared to other chelating agents such as PDTA (propylene-diamino-tetraacetic acid) and EDTA (ethylene-diamino-tetraacetic acid) DTPA has a greater affinity for zinc. Therefore one might expect any biological activity relating to the chelation of zinc to be more pronounced with DTPA than other chelating agents, although bioavailabilty will also play a significant role.
It should also be recognised that capacity of DTPA to bind zinc in the gut and in the systemic circulation will still be affected by the presence of other metals and the availability of zinc to bind. This is covered in more detail in the classification and labelling argument attached to section 13 of this IUCLID.
DTPA and Zinc excretion
Having established that DTPA has a high affinity for zinc, the effect of DTPA administration to experimental animals and humans on zinc status can be considered. There are numerous studies examining the effects of administration of the calcium salt of DTPA (CaDTPA) to animals on the excretion of zinc (urinary and fecal). (Cantilena and Klassen, 1982; Cohen and Guilmette, 1976; Domingoet al.,1988, Planas-Bohneet al.,1975 and 1976; Tandonet al.,1984). In these studies the routes of administration were either; intraperitoneal, intravenous or subcutaneous. The dosing regimes used in these studies include; single doses, multiple doses in one day, multiple daily doses, and continuous infusion. The results of all of these studies have shown that administration of CaDTPA resulted in an increased excretion of zinc relative to control regardless of the route of administration. Similar work conducted using the zinc salt of DTPA has demonstrated that administration of this compound did not cause an increase in the excretion of zinc (after taking into account the zinc that is part of the test substance). This work has also identified that the calcium form of DTPA is far more toxic than the zinc form. The investigators commenting that the chelation of endogenous metals such as zinc and perhaps manganese and subsequent increased excretion is responsible for the toxicity (Planas-Bone et al1976).
Further evidence of the potential of DTPA to cause an increase in zinc excretion comes from work in mice and rats to identify an effective antidote for acute zinc intoxication (Llobet et al 1988; Domingo et al 1988). This work compared a number of chelating agents including DTPA and EDTA. In both studies mice (or rats) were administered lethal doses of zinc via intraperitoneal injection and then administered doses of chelating agents either immediately or 10 minutes later via intraperitoneal injection. The antidotal effectiveness was assessed by comparing the degree of zinc induced mortality. Llobet et al (1988) also assessed the excretion of zinc in the feces and urine. In both studies the calcium salt of DTPA was most protective against acute zinc toxicity. It also caused an increase in zinc excretion in the urine and feces.
DTPA and Zinc deficiency
Data in humans show that intravenous administration of DTPA (the calcium salt) is capable of causing an increase in the excretion of zinc, in some cases leading to a deficiency. In one case study, (Proksch and Koumllmel, 1985), a patient treated for manganese poisoning with CaEDTA and CaDTPA exhibited zinc deficiency syndrome with acrodermatitis enteropathica-like skin changes. This cleared up following oral administration of zinc aspartate. In a second study (Kalkwarf et al.,1983) levels of trace metals were assessed in the urine samples of a worker contaminated in the 1976 Americanum incident. This worker had been treated over the course of 3 years with different forms of DTPA (CaNaDTPA or ZnNaDTPA) in an effort to reduce the radio nuclide contamination. Analysis of the workers urine identified that of all the trace metals assessed, zinc was the only one excreted at much higher levels than ‘normal’ and the peaks in zinc excretion appeared to correspond to treatments with CaNaDTPA. The treatment schedule also included intermittent zinc supplementation. It was noted that this supplementation or administration of ZnNaDTPA compensated for the loss of zinc caused by CaNaDTPA.
With the exception of the more recent toxicity studies conducted with sodium or potassium DTPA, all previous experimental investigations of DTPA (calcium or zinc salts) have been conducted using non-oral routes of administration. Therefore there is very little information on the effect of oral administration of DTPA (gavage or dietary) on the general health (including zinc status) of animals and man. However in a 28 day study in rats (Elliotet al.,1987), gavage administration of the potassium salt of DTPA at doses up to 1330 mg/kg bw/day resulted in clear signs of toxicity including an increase in mortality (at the highest does). In the mid and low dose animals (83 and 333 mg/kg) there were no mortalities and with the exception of a reduced feed consumption in some mid dose males, there were no clinical signs observed. In the highest dose group, there were several mortalities in both sexes (4/5 male, 1/5 female). Prior to death, the male animals displayed hunched posture, abnormal gait, diarrhea, piloerection, yellow-brown staining of fur and decreased respiration rate. High dose animals also consumed less food and watery contents in the caecum were noted in 3/4 high dose females at necropsy. These effects are consistent with those observed following a similar treatment regime with EDTA and other structurally similar chelating compounds. Owing to the low oral absorption of DTPA, it is probably exerting its effects, such as binding dietary zinc, in the gut, thus causing an absorption-mediated deficiency of zinc, rather than a deficiency due to increased urinary elimination. Whilst these data do not show that oral dosing of DTPA is capable of causing a zinc deficiency,it does demonstrate the consistency in toxicity with other chelating compounds such as EDTA which are generally believed to cause a zinc deficiency (Swenerton and Hurley 1971; EDTA RAR 2004) leading to developmental toxicity, albeit at much higher doses (>1000mg/kg).
In summary, the available data demonstrate that
Is Zn deficiency per se a trigger for embryo-/fetotoxicity?
The following text is an extract from Rogers et al. (1985) that gives a good summary of the spectrum of effects of zinc deficiency on the developing fetus.
“The production of congenital malformations in a mammal by maternal Zn deficiency was first reported in 1966 by Hurley and Swenerton in the Sprague-Dawley rat (Hurley and Swenerton, '66). Since this initial report, there has been considerable work on the teratogenic effects of Zn deficiency and the mechanisms involved. Virtually every developing organ system has been shown to be adversely affected by maternal Zn deficiency; the types of defects produced by Zn deficiency are many, and they occur with high frequencies. Fetuses of Zn-deficient rats have been reported to have brain defects, eye defects, cleft palates, and skeletal defects, as well as gross malformations of the cardiovascular, respiratory, and urogenital systems (Hurley and Swenerton, '66; Hurley, '69; Mills et al., '69; Hurley and Shrader, '72; Warkany and Petering, '72; Apgar, '72). In addition to these structural defects, the biochemical development of the lung (Vojnick and Hurley, '77) and pancreas (Robinson and Hurley, '81a, b) is also adversely affected by gestational Zn deficiency in the rat.”
Subsequently it is very well understood that a nutritional deficiency in zinc in the maternal organism can produce very severe consequences in the developing fetus. However an important consideration is that the extent, duration and timing of zinc deficiency during pregnancy will affect the severity and localization of the effects on the developing fetus (Hurley et al., 1971; Record et al., 1985; Hickory et al., 1979). This is important because less extensive effects are associated with a mild or fluctuating zinc deficiency during pregnancy, and the timing during pregnancy will determine the organ systems/skeletal structures affected (Hurley et al., 1971; Record et al., 1985; Hickory et al., 1979). The effects of short term/transitory zinc deficiency highlight the fact that in rats the maternal organism does not seem to be capable of compensating for a sudden drop in zinc intake by mobilizing zinc stores (Hurley et al., 1971); in fact it seems that during periods of zinc deficiency the maternal liver sequesters zinc via induction of the zinc-binding protein, matallothionein, thus restricting the supply of zinc to the embryo/fetus (Rogers et al., 1985). King (2000) notes that in humans and animals, transfer of sufficient zinc to the fetus is dependent on maintenance of normal maternal serum zinc concentrations, therefore interfering with the maternal zinc status is the first step in producing a zinc deficiency in the developing fetus. Importantly, teratogenic effects have resulted from a decrease in the transfer of zinc to the embryo, even in the absence of detectable decreases in absolute zinc levels (Daston et al., 1991; Keen et al., 2003; Leazer et al., 1992; Tauberneck et al., 1994). Thus it appears possible to induce teratogenicity in the absence of overt visible maternal toxicity.
In the studies mentioned above examining zinc deficient diets and developmental toxicity it is often reported that maternal feed consumption and bodyweight gain were reduced compared to control animals. However where a pair fed control group was also included, the restriction in food intake was shown to cause some decrease in maternal bodyweight gain but it did not result in any developmental toxicity (Rogers et al., 1985). These findings implicate zinc deficiency, not reduced feed consumption as the causative agent responsible for developmental toxicity.
In summary, there is a significant body of literature on the effects on the developing fetus of deficiencies in nutrients such as zinc. From this database it is very clear that zinc plays such an important role in so many of the processes involved in the growth and development of the fetus that a deficiency in this nutrient has serious consequences. Subsequently any substance capable of negatively affecting the zinc status of the maternal organism is likely to have adverse effects on the developing fetus resulting in varying degrees of malformations. These malformations will also depend on the duration and severity of the deficiency.
What is the relevance of this mechanism (zinc deficiency) for the effects observed with DTPA in the teratogenicity study?
DTPA and evidence of zinc deficiency mediated teratogenicity
Fisher et al (1975) administered ZnDTPA (6 mice at 360 micromol/kg bw and 6 mice at 2900 micromol/kg bw), CaDTPA (6 mice at 360 micromol/kg bw and 12 mice at 2900 micromol/kg bw) or saline solution (12 mice) to female mice (strain C57BL/Do) via daily subcutaneous injections. These doses are equivalent to 199 or 1600mg ZnDTPA /kg bw and 179 or 1441 mg CaDTPA/kg bw. The dosing period started 4 days after the mating period began and continued throughout pregnancy until the pups reached an age of 13 days. In the group of mice dosed with 2900 micromol/kg bw CaDTPA there were no viable offspring observed. Only one stillborn pup was observed but it appeared grossly normal. In the 360 micromol/kg bw CaDTPA group there were no adverse effects on reproduction or developmental parameters. Both dose levels of ZnDTPA were reported to be ‘completely harmless’ to the mothers and the pups.
Fisher et al. (1976) administered a range of doses of CaDTPA to pregnant mice (strain - C57BL/Do) via daily subcutaneous injections for different 4 day periods during pregnancy. The mice were separated into 3 groups and were dosed on either days 2-6, 7-11 or 12-16 during pregnancy. The mice received injections of 0, 720, or 1440 micromoles/kg bw (days 2-6 or 7-11) or doses of 0, 720, 1440, or 2880 micromoles/kg bw (days 12-16). These doses are equivalent to 0, 357, 715 and 1430 mg CaDTPA/kg bw. The dams were sacrificed on day 18 of gestation and the fetuses examined for morphologic alterations. CaDTPA (357 or 715 mg/kg bw) dosed either from day 2 to 6 or from day 7 to 11 of gestation resulted in an increase in resorptions compared to control. Neither of these doses caused an increase in resorptions (relative to control) when dosed from day 12 to 16 whereas 1430 mg/kg bw did. Dosing with 715 mg/kg bw produced malformations in fetuses in all dosing period groups. The types of malformation and number of fetuses affected varied however with the dosing schedule. The malformations observed were typical of those associated with zinc deficiency (exencephaly with ablepharia, exencephaly, spina bifida aperta, cleft palate). Polydactyly was also observed when 715 and 1430 mg/kg bw were administered from days 12 to 16. The authors noted that this may not be a true effect of CaDTPA as the strain of mouse used has a background incidence of 1.5% for this malformation. However this type of malformation is still consistent with zinc deficiency and so should not be ruled out all together. Dosing with 357 mg/kg bw only produced malformations when dosed from days 2 to 6 and these malformations were consistent with those observed with 715 mg/kg bw dosed for the same period.
Brummett and Mays (1977) investigated the teratogenicity of the zinc salt of DTPA in the mouse using a similar protocol to that used by Fisher et al., (1976). Pregnant mice were subcutaneously dosed with ZnDTPA daily either from days 2-6 or 7-11 during gestation. The strain of mice (C57BL/Do) was the same as that used by Fisher et al (1975 and 1976). The doses of ZnDTPA used were either 0, 5720 or 11520 micromoles/kg bw; these doses are equivalent to 0, 3163 and 6371 mg/kg bw. Due to the hypertonic nature of the test material an additional group of mice were treated with a solution of sodium chloride (1380 micromole NaCl/ml) at the same ion concentration, osmolality, pH and volume as the high dose ZnDTPA treatment. A CaDTPA dose group (1440 micromole/kg bw = 715 mg/kg bw) dosed daily on days 7-11 was also included in this study. The pregnant mice were euthanized on day 18 of gestation and the fetuses removed and examined for gross malformations, visceral malformations and skeletal malformations. Dosing with ZnDTPA in this study did not result in any malformations of the fetuses although 6371 mg/kg (days 2-6 and 7-11) and 3163 mg/kg (days 7-11) caused an increase in embryo toxicity relative to controls (aborted litters or resorptions). However administration of the hypertonic saline solution also caused an increase in aborted litters and resorbed fetuses relative to control. The only malformed fetus observed was in the CaDTPA group which had exencephaly. Considering the previous studies on CaDTPA it seems likely that had it been dosed in this study from days 2-6 then the malformations observed would have been far more extensive. This seems to suggest that it could take a few days for DTPA to induce a zinc deficiency and that it might last for a few days after dosing has ceased since organogenesis peaks in the mouse between days 7 and 11 of gestation, thus the sensitivity to zinc induced malformations should be greatest during this time period.
In a follow up study to that reported by Brummett and Mays (1977), Calder et al. (1978) dosed mice daily via subcutaneous injection 4 days after mating began until birth or until 29 injections had been administered. Two forms of ZnDTPA were used in this study, one commercial batch with no NaCl present and another made in the lab containing NaCl. No gross malformations were reported in this study although it appears only an external exam was performed. The authors report that 6371 mg/kg bw ZnDTPA (commercial grade) was not toxic to either dams or pups although it did produce a statistically significant drop in pup weight. Doses of 1560 or 3163 mg/kg bw ZnDTPA (commercial or lab grade) did not significantly alter any of the parameters examined (pup weight, pups/litter, abortion rate) relative to control. The study also followed the progress of the dams and the pups for their remaining lifespan to understand whether there were delayed effects on fertility or viability. At the time of this study report there was no evidence of any impairment in the fertility of the mature pups or the viability of their offspring however no additional details are given.
The above studies appear to demonstrate that CaDTPA is capable of causing fetotoxicity and malformations consistent with zinc deficiency and that the frequency and type of these malformations is dependant on the dosage and dosing period during pregnancy. Conversely ZnDTPA dosed at significantly higher dose levels for equivalent dosing periods does not appear to cause malformations. It does however result in increased fetotoxicity albeit at extremely high dose levels. The explanation given by the investigators as to why there is a difference in teratogenicity between the calcium and zinc salts of DTPA is that the toxicity is due to the chelation of essential metals such as zinc and manganese (consider the data on increased excretion of zinc following DTPA administration) and that the zinc salt of DTPA cannot chelate any additional zinc. CaDTPA on the other hand will release the calcium and bind zinc in the body increasing its excretion and producing a zinc deficient state.
The support for the mechanism of maternal zinc deficiency being responsible for teratogenicity of chemicals capable of depleting zinc comes from work where a zinc depleting agent was dosed in conjunction with a zinc supplemented diet. Doses that previously caused teratogenicity were found to be non teratogenic in the presence of sufficient zinc (Swenerton and Hurley (1971). Considering this work, it is plausible that if the diet used in the BASF study using sodium DTPA (BASF 1994) had been supplemented with zinc as done by Swenerton and Hurley (1971) then the developmental toxicity would have been prevented. This would also be consistent with the difference in the toxicity of calcium DTPA compared with zinc DTPA.
Whilst the data above demonstrate that DTPA is capable of producing malformations when not dosed as the zinc salt, there has been some question about the types of malformations observed in the developmental toxicity study conducted on sodium DTPA (BASF 1994). In that study sodium DTPA was dosed daily to pregnant rats via gavage at doses of 100, 400 and 1000 mg/kg bw, gestation days 6 to 15. The effects observed in the fetuses in this study were not as severe as those observed with subcutaneous dosing of CaDTPA, or a complete zinc deficiency. It has also been noted that missing vertebrae is not a malformation commonly associated with zinc deficiency and therefore perhaps there is another mechanism of action. However missing vertebrae resulting from a zinc deficiency has been reported in the past (Swenerton and Hurley 1966) as have delays in ossification, missing ossification centers, bipartite sternebrae and many other skeletal deformities (Rogers et al., 1985; Stevens et al., 1962; Hurley et al., 1971; Jankowski et al., 1995; Record et al., 1985, 1986; Ferreira et al., 1989). As mentioned previously, zinc deficiency potentially can affect a broad range of developmental processes, with the specific malformations manifested being mainly a function of exposure timing, duration and dose. Assuming that a functional zinc deficiency requires at least few days of dosing, the zinc deficiency likely occurred during a stage of embryogenesis when axial skeleton patterning (i.e., the vertebrae and its derivatives) is being established. Thus, a causal association between DTPA-induced zinc deficiency and missing vertebrae is entirely plausible.
Perhaps when considering the less severe effects observed in the BASF study it should be acknowledged that the route of administration can affect the degree and frequency of malformations observed. Chelating agents dosed in the diet rather than via gavage will have a larger window of opportunity to interact with essential metals in the gut and subsequently preventing their absorption by forming a complex that is less able to be absorbed in the gut and increasing excretion. Therefore dietary dosing will probably be more effective at producing a zinc deficiency than gavage dosing. Thus perhaps the use of gavage dosing in the BASF study was in part responsible for the less severe malformations observed.
In summary,
• The zinc salt of DTPA is significantly less teratogenic/fetotoxic than the calcium salt. This is most likely due to the induction of a maternal zinc deficiency by CaDTPA resulting from an increase in zinc excretion.
• It is apparent therefore that the salt form of DTPA which is dosed to pregnant animals is directly related to the teratogenicity potential and this in turn is related to the potential for chelation of zinc. Thus salt forms of DTPA that can chelate zinc will be potentially teratogenic i.e. those salts where the DTPA-metal complex has a lower dissociation constant than ZnDTPA.
• The concept of a mode of action involving zinc deficiency is further supported by the data on other chemicals that can deplete zinc, where zinc supplementation negated the teratogenicity of a dose known to be teratogenic.
• The dosing route plays a very important role in the elicitation of a teratogenic response, dietary dosing being more effective than gavage dosing and subcutaneous/intravenous or intraperitoneal dosing being more effective than oral dosing.
• The duration of dosing and timing during pregnancy are also important factors influencing the type, severity and frequency of malformations.
Conclusions
The difference in the teratogenicity of the calcium and zinc salts of DTPA indicates that DTPA itself is unlikely to be having a direct teratogenic effect on the developing fetus. If DTPA were directly teratogenic then the salt form should not significantly influence the teratogenicity particularly following intravenous or subcutaneous dosing.
DTPA has a strong affinity for zinc when compared to other metals such as sodium, potassium and calcium. It is also not absorbed well from the intestinal tract following oral dosing in humans and thus absorption in rats is also likely to be poor. Therefore it is plausible that oral dosing of pregnant rats with DTPA will lead to zinc being bound in the gut and excreted in the faeces. Absorbed DTPA will either be bound already to zinc or will bind endogenous zinc (or other metals) in the body before being excreted.
The potential increase in zinc excretion associated with oral dosing with DTPA may then cause a reduction in the zinc available to the mother and the developing fetuses. Considering that pregnant rats receiving sub optimal levels of zinc do not appear to be able to mobilize tissue stores of zinc to ensure adequate supply to the fetus, the decrease in zinc intake/increased zinc excretion will result in an insufficient supply of zinc to the fetus. An insufficient zinc supply on the developing fetus produces malformations and increased fetotoxicity.
The timing of this drop in zinc availability during pregnancy will determine the degree of teratogenicity. Due to the relatively limited spectrum of effects observed in the BASF study it is probable that the gavage dosing of DTPA did not cause a complete deficiency in zinc throughout pregnancy but instead zinc levels fluctuated, deficient levels coinciding with certain stages in fetal development such as skeletal development and bone ossification.
In conclusion there are adequate data to support the hypothesis that the teratogenicity resulting from gavage dosing of rats with DTPA is a result of an induced deficiency of zinc in the mother which subsequently impacts the fetus.
Justification for classification or non-classification
Reproductive toxicity classification:
There are no data available on DTPA relating to multi generation toxicity. As indicated previously, if a multi generation study were to be conducted it is plausible that effects on fertility (specifically male fertility) could be observed, however such effects would be secondary to a zinc deficiency and would only occur in conjunction with clear systemic toxicity consistent with such a deficiency. Taking into account the fact that worker or consumer exposure would not lead to sufficient DTPA exposure to cause a deficit in an individuals zinc status (see below and document attached at section 13) it is not considered appropriate to classify for reproductive toxicity.
Developmental toxicity classification:
The chelating agent DTPA appears to be developmentally toxic following high oral doses in rats. DTPA complexes with essential metals such as zinc in the gut, preventing these from becoming bio-available. At high doses this deprivation of essential nutrients leads to toxic effects such as developmental toxicity due to the importance of zinc in the healthy development of a growing fetus. As a result of this it is considered appropriate to classify DTPA for developmental toxicity. In determining the appropriate classification an assessment of the relevance of the hazard to humans has been made. This assessment takes two parts; 1) estimation of exposures to the workforce (the group with the highest exposure levels) and 2) an assessment of how these exposures may affect the zinc status of the workforce, specifically a pregnant worker.
Taking conservative assumptions into account, the estimated worker exposures to DTPA are low. Due to the much lower levels of DTPA in formulated products that may be available to the consumer, consumer exposure levels would be significantly lower than worker exposure levels. The effect of estimated worker exposures to DTPA under normal working conditions or following an accidental acute exposure would not be considered to be detrimental to a workers (including pregnant women) zinc status. Thus it is highly unlikely that a consumer’s zinc status would also be negatively affected, considering the significantly lower level of exposure likely in the general population. Thus exposure to DTPA would not result in the development of a zinc deficiency. According to the text of the Classification, Labelling and Packaging directive, where data from animals provide clear evidence that a substance is capable of producing reproductive or developmental toxicity, this substance can be classified as category 1B. However, within the framework of a weight of evidence approach it is demonstrated in a comprehensive exposure assessment that the occurrence of developmental toxicity in humans would require unrealistic exposure situations and thus is extremely unlikely, therefore Classification of DTPA in Category 2 according to the CLP directive is considered to be appropriate. (Equivalent to Category 3 Developmental toxin according to the Dangerous substances directive).
The basis for this assessment is provided in the Classification and Labelling justification document attached to this IUCLID at section 13.
Additional information
Information on Registered Substances comes from registration dossiers which have been assigned a registration number. The assignment of a registration number does however not guarantee that the information in the dossier is correct or that the dossier is compliant with Regulation (EC) No 1907/2006 (the REACH Regulation). This information has not been reviewed or verified by the Agency or any other authority. The content is subject to change without prior notice.
Reproduction or further distribution of this information may be subject to copyright protection. Use of the information without obtaining the permission from the owner(s) of the respective information might violate the rights of the owner.
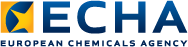