Registration Dossier
Registration Dossier
Data platform availability banner - registered substances factsheets
Please be aware that this old REACH registration data factsheet is no longer maintained; it remains frozen as of 19th May 2023.
The new ECHA CHEM database has been released by ECHA, and it now contains all REACH registration data. There are more details on the transition of ECHA's published data to ECHA CHEM here.
Diss Factsheets
Use of this information is subject to copyright laws and may require the permission of the owner of the information, as described in the ECHA Legal Notice.
EC number: 231-105-1 | CAS number: 7439-96-5
- Life Cycle description
- Uses advised against
- Endpoint summary
- Appearance / physical state / colour
- Melting point / freezing point
- Boiling point
- Density
- Particle size distribution (Granulometry)
- Vapour pressure
- Partition coefficient
- Water solubility
- Solubility in organic solvents / fat solubility
- Surface tension
- Flash point
- Auto flammability
- Flammability
- Explosiveness
- Oxidising properties
- Oxidation reduction potential
- Stability in organic solvents and identity of relevant degradation products
- Storage stability and reactivity towards container material
- Stability: thermal, sunlight, metals
- pH
- Dissociation constant
- Viscosity
- Additional physico-chemical information
- Additional physico-chemical properties of nanomaterials
- Nanomaterial agglomeration / aggregation
- Nanomaterial crystalline phase
- Nanomaterial crystallite and grain size
- Nanomaterial aspect ratio / shape
- Nanomaterial specific surface area
- Nanomaterial Zeta potential
- Nanomaterial surface chemistry
- Nanomaterial dustiness
- Nanomaterial porosity
- Nanomaterial pour density
- Nanomaterial photocatalytic activity
- Nanomaterial radical formation potential
- Nanomaterial catalytic activity
- Endpoint summary
- Stability
- Biodegradation
- Bioaccumulation
- Transport and distribution
- Environmental data
- Additional information on environmental fate and behaviour
- Ecotoxicological Summary
- Aquatic toxicity
- Endpoint summary
- Short-term toxicity to fish
- Long-term toxicity to fish
- Short-term toxicity to aquatic invertebrates
- Long-term toxicity to aquatic invertebrates
- Toxicity to aquatic algae and cyanobacteria
- Toxicity to aquatic plants other than algae
- Toxicity to microorganisms
- Endocrine disrupter testing in aquatic vertebrates – in vivo
- Toxicity to other aquatic organisms
- Sediment toxicity
- Terrestrial toxicity
- Biological effects monitoring
- Biotransformation and kinetics
- Additional ecotoxological information
- Toxicological Summary
- Toxicokinetics, metabolism and distribution
- Acute Toxicity
- Irritation / corrosion
- Sensitisation
- Repeated dose toxicity
- Genetic toxicity
- Carcinogenicity
- Toxicity to reproduction
- Specific investigations
- Exposure related observations in humans
- Toxic effects on livestock and pets
- Additional toxicological data

Endpoint summary
Administrative data
Link to relevant study record(s)
- Endpoint:
- basic toxicokinetics in vivo
- Type of information:
- experimental study
- Adequacy of study:
- supporting study
- Reliability:
- 2 (reliable with restrictions)
- Rationale for reliability incl. deficiencies:
- other: see 'Remark'
- Remarks:
- Restrictions - no claims that the study had been conducted and reported according to international accepted guidelines or in compliance with the principles of GLP. However, the study has been recently conducted and appears to contain a significant level of detail.
- Objective of study:
- distribution
- Qualifier:
- no guideline followed
- Principles of method if other than guideline:
- The purpose of this study is to compare the patterns of Mn distribution in various brain regions (olfactory bulb, frontal parietal cortex, globus
pallidus, striatum and cerebellum) and other tissues (lung, liver, kidney, testis) and the neurobehavioral damage following inhalation exposure of rats to three Mn species. - GLP compliance:
- not specified
- Radiolabelling:
- no
- Species:
- rat
- Strain:
- other: Sprague–Dawley CD (Crl:CD[SD] IGS BR)
- Sex:
- male
- Details on test animals or test system and environmental conditions:
- TEST ANIMALS
- Source: Charles River (St. Constant, Quebec, Canada)
- Age at study initiation: aged between 34 and 38 days
- Weight at study initiation: weighing 125–150 g
- Housing: Rats were individually housed in a polycarbonate cage with stainless steel wire lids under constant conditions of temperature, humidity
- Diet: Ad libitum
- Water: Ad libitum
- Acclimation period: Acclimatized for a week prior to initiation of the study
ENVIRONMENTAL CONDITIONS
- Photoperiod (hrs dark / hrs light): 12 hour cycle - Route of administration:
- inhalation: dust
- Vehicle:
- unchanged (no vehicle)
- Details on exposure:
- TYPE OF INHALATION EXPOSURE: whole body
GENERATION OF TEST ATMOSPHERE / CHAMPER DESCRIPTION
- Exposure apparatus: Inhalation exposure was conducted in two rectangular stainless steel chambers (131 cm long, 65 cm wide and 125 cm deep) with a total volume of 1 m3 each (Hazelton Systems Company Inc., Kalamazoo, Michigan).
- Method of holding animals in test chamber: Rats were housed individually in 15 cm (long) x 15 cm (wide) x 20 cm (deep) stainless steel wire mesh cages.
- Source and rate of air: Mn aerosol particles were supplied to the inhalation chamber using a Fluidized Bed Aerosol Generator (Model 3400 TSI Inc., St.-Paul, MN).
- Method of conditioning air: Air entering the chamber was filtered through a high efficiency particulate air (HEPA) filter. Before entering the chamber, Mn aerosol particles were delivered through a stainless steel AS ME pressure tank (Model 73, Mc Master-Carr, NJ) that allowed settling of larger Mn aerosol particles
- Method of particle size determination: Once every 2 weeks, air samples were collected using a six-stage Marple Personal cascade Impactor in order to establish the particle size. - Duration and frequency of treatment / exposure:
- Rats were placed in the inhalation chamber 6 h per day, 5 days per week during 13 consecutive weeks.
- Remarks:
- Doses / Concentrations:
A group was exposed to a target level of approximately 4000 mg/m3 of metallic Mn. A control group was used. Rodent pellet chow and tap water were available ad libitum except when the animals were in the exposure chambers. Manganese concentration in this diet was approximately 95 ppm while Mn concentration in drinking water was approximately 0.001 ppm. - No. of animals per sex per dose / concentration:
- Animals were randomly divided into four groups of 15
- Control animals:
- yes, concurrent no treatment
- Details on dosing and sampling:
- PHARMACOKINETIC STUDY
- Tissues and body fluids sampled : All brains were removed and were hemisected in the sagittal plane. The right brain hemisphere, to be used for Mn analysis, was dissected into five regions: olfactory bulb, frontal parietal cortex, cerebellum, globus pallidus and striatum. During striatum and globus pallidus dissection, care was taken to avoid the white matter fibers of the internal capsule being blended into the sample. Also the lung, liver, testis, and kidney were also dissected for Mn analysis.
Body weights measured weekly. - Statistics:
- All statistical analyses were performed using SPSS Statistical Software (Version 10.0.5). Various statistical tests, such as one-way ANOVA post hoc multiple comparison (Tukey; Bonferroni, Dunnett T3-Test and Tamhane T2) intra and inter categories, were used to compare data for locomotor activity and Mn concentration in all the organs and tissues. Homogeneity of variances for the exposed groups was tested with Levene’s test, and all statistical analyses were performed on untransformed data. A probability value of less than 0.05 (i.e. P < 0.05) was used as the critical level of significance within each statistical test.
- Metabolites identified:
- not measured
- Conclusions:
- Interpretation of results: Bioaccumulation potential cannot be judged based on study results
The results show that Mn metal can be distributed in the brain and other tissues in rat, as a result of inhalation of respirable particles. - Endpoint:
- basic toxicokinetics in vivo
- Adequacy of study:
- supporting study
- Reliability:
- other: 5
- Rationale for reliability incl. deficiencies:
- other: see 'Remark'
- Remarks:
- This appears to be the same study data as used in the Normandin 2004 publication (manganese metal data). There are a few minor differences that appear to be due to the number of animals per group. As such this publication was not considered to uniquely and unequivocally contain data that could inform the toxicokinetic assessment of manganese.
- Qualifier:
- no guideline followed
- Principles of method if other than guideline:
- 3.75 mg/m3 (as respirable Mn) exposure 6h/day 5 days/wk for 13 weeks. Blood chemistry + organ weights measured
- GLP compliance:
- not specified
- Species:
- rat
- Strain:
- Sprague-Dawley
- Sex:
- male/female
- Route of administration:
- inhalation: aerosol
- Conclusions:
- Interpretation of results: Bioaccumulation potential cannot be judged based on study results.
The study demonstrates absorption and distribution of Mn into various tissues including brain tissues following inhalation of respirable Mn metal dust.
Referenceopen allclose all
Mn Concentrations in the Inhalation Chamber
The Mn concentrations (mean ±S:D) in the air of the inhalation chamber for metallic Mn and the control group were 3750±846 and 0.30±0.02 mg/m3respectively. Ninety percent of the metallic Mn particles in the inhalation chamber were smaller than 1.55mm.
Mn Tissue Concentrations
Exposure led to an increase in brain Mn concentrations (mean±S:D). With the exception of the frontal parietal cortex and the cerebellum of rats in metallic Mn exposure group, brain Mn concentrations were significantly higher compared to controls. Increased lung Mn concentrations were observed following inhalation exposure to metallic Mn. No increase in liver Mn concentrations were observed. The concentrations in the five brain regions were higher in the exposed rats.
In the table below the percentage increase of Mn in brain regions and tissues is detailed relative to the control group following 13 weeks of exposure
|
Mn concentrations in lung, putamen, and cerebellum were significantly higher in E than in C (0.30 vs. 0.17, 0.89 vs. 0.44, 0.63 vs. 0.48 ppm; p <0.01), as well as in the kidney, frontal cortex, and globus pallidus (1.15 vs. 0.96, 0.84 vs. 0.47, 1.28 vs. 0.55 ppm; p <0.05). Potassium concentration was significantly lower in E than in C (5.11 vs. 5.79 mmol/L; p <0.05), as was alkaline phosphatase (106.9 vs. 129.6 U/L; p <0.01). Locomotor activity indicated higher distance covered in the
first 12-h period for E (45 383 vs. 36 098 cm; p <0.05) and lower resting time in the last 12-h period for E (36 326 vs. 37 393 s; p <0.05).
Description of key information
An assessment of the substance's toxicokinetics has been undertaken based on the findings of the data available as detailled throughout the registration dossier.
Key value for chemical safety assessment
- Bioaccumulation potential:
- no bioaccumulation potential
- Absorption rate - oral (%):
- 5
- Absorption rate - dermal (%):
- 0
Additional information
TOXICOKINETIC ASSESSMENT
Manganese (Mn); (EC Number 231-105-1, CAS Number 7439-96-5)
The substance, manganese metal, can occur as the free element in nature (often in combination with iron) and in many minerals. It is a silvery gray-white metallic solid and can compose of large flakes to a fine powder. Particle size analysis of a typical sample after removal of the largest flakes has shown that only 1.5% of the particles were smaller than 100 µm diameter. However, finest grade material can have 10-fold more particles smaller than 100 µm diameter.
Absorption
The test material, manganese metal, has a very low water solubility of 7.0 x 10-4g/L of manganese in solution at 20.0°C(Butler and White 2009). The test material also has a low solubility (0.020%) in artificial alveolar based upon the extractable manganese(Anderson 2009). Since the finest grade test material can have up to 15% of particles smaller than 100 µm diameter, the test material was subjected to an acute inhalation toxicity (nose only) study in the rat(Griffiths 2010). In order to facilitate aerosolisation and reduce particle size, the test material was ground using a centrifugal ball mill prior to use. A group of 10 rats were exposed to a mean atmosphere concentration of 5.14 mg/L test material with a mean mass median aerodynamic diameter of 5.55 µm and a prediction of 40% of particles being less than 4 µm. The results concluded that the acute inhalation median lethal concentration (4 hr LC50) of manganese metal was greater than 5.14 mg/L in the rat. As such, although manganese metal has the potential to be inhaled due to its particle size distribution in finest grade materials; it doesn’t exhibit inhalation toxicity at a high dose in the rat. Since the test material has a low solubility (0.020%) in artificial alveolar it is likely that most of the inhaled test material was not absorbed but instead was cleared from the lungs by the mucocilliary elevator into the gastrointestinal (GI) tract. However, in chronic inhalation exposure, such poorly soluble particles have been shown to produce effects such as decreasing particle clearance from the lungs as well as pulmonary inflammation (Lee et al., 1985). This phenomenon was described by ECETOX, 2013 as “lung overload’. In the same technical document, this phenomenon was also reported to be rat specific. In a repeated dose toxicity study conducted over a 90 day period in accordance with OECD Guideline 413, specifically milled samples of manganese metal were prepared and used to generate the aerosols used in the study. These samples were of much smaller MMAD than typical manganese powders and are considered to represent a worst-case scenario. No test-item related findings were noted during performance of the functional observation battery. There were no test-item related ophthalmoscopic findings. There was an increase in manganese concentration measured in whole blood samples taken during treatment and a dose dependent increase in manganese concentration in erythrocytes and platelets. Dose dependent increase in manganese levels was detected in brain tissue during the study but values returned to normal after the recovery period. This indicates that there is some measurable absorption of Mn. Microgranuloma, alveolitis and inflammatory lesions were observed and are considered indicative for an adverse particle overload reaction rather than systemic toxicity as chronic inhalation exposure to high particle concentrations* of low soluble particles of low toxicity hare eliciting comparable localised pulmonary toxicity via processes that are pro-inflammatory in nature, causing oxidative stress and persistent pulmonary inflammatory response (reported by ECETOX, 2013) mainly driven by their physical state.
*amount of poorly soluble material deposited daily in the lungs
Manganese metal has considerable solubility (59±2.9%) in artificial gastric juice(Anderson 2009). Although 59% of the available Mn dissolves in simulated gastric fluid, only 5% of any Mn is actually absorbed via the alimentary canal. So, if a small amount is swallowed it would be (wt in g) x 59% x 5% i.e. still relatively low amounts absorbed. The acute oral median lethal dose (LD50) of the test material in the female Wistar strain rat was estimated to be greater than 2000 mg/kg bodyweight (Pooles 2009). As such the test material has a low potential for toxicity by oral absorption. As the test material has a very low solubility in water coupled with its physical inorganic nature (metallic solid) means that it is very unlikely to be absorbed through the skin.
In conclusion, the test material has a low potential for any absorption by oral ingestion, inhalation or dermal absorption.
Metabolism, Distribution and Excretion
Since the test material has a low potential for absorption by any route it means that the test material will not be readily bioavailable. The majority of any test material that is ingested orally is likely to pass through the GI tract unchanged and be excreted in the faeces. Any small amount of manganese that is absorbed by the gut will enter the essential manganese pool along with that which is absorbed from the daily nutritional requirement of manganese. The circulating amount of manganese will be controlled by the normal homeostatic mechanism provided by the liver that controls the manganese balance. Any test material that is inhaled is likely to be cleared from the lungs by the mucocilliary elevator into the GI tract and again excreted unchanged in the faeces. Increases in measured manganese levels following exposure to manganese dust via inhalation have been shown to return to normal over time upon removal from exposure – recovery period. It can be inferred that quantities of manganese adsorbed through the respiratory tract would also be excreted under control of the body’s normal homeostatic mechanism.
References
Anderson, K. A. (2009). Bioaccessibility of manganese from manganese Materials in Gastric and Lung (Alveolar) Biofluids, Oregon State University.
Broich, K. (2016). Mn Metal Powder: 13-Week Inhalation Toxicity Study in the Wistar Rat with a 4-Week Recovery Period. Project Number D32135, Envigo CRS (Switzerland) Limited.
Butler, R. E. and D. F. White (2009). Mn Metal: (The Manganese Metal Company/BHP): Determination of Water Solubility and Particle Size Distribution. Project number 2702/0001, Harlan Laboratories Ltd.
European centre for ecotoxicology and toxicology of chemicals, Technical report No. 122, Poorly soluble particles/Lung overload – December 2013 ( ISSN-2079-1526-122 ( online)
Griffiths, D. R. (2010). Mn metal: Acute Inhalation Toxicity (Nose Only) Study in the Rat. Project Number 2702/0108, Harlan Laboratories Ltd.
Lee KP, Trochimowicz HJ, Reinhardt CF. Pulmonary response of rats exposed to titanium dioxide (TiO2) by inhlation for two years. Toxicol Appl Pharmacology 79: 179-182
Pooles, A. (2009). Mn metal: Acute Oral Toxicity in the Rat - Fixed Dose Method. Project Number 2702/0083, Harlan Laboratories Ltd.
Information on Registered Substances comes from registration dossiers which have been assigned a registration number. The assignment of a registration number does however not guarantee that the information in the dossier is correct or that the dossier is compliant with Regulation (EC) No 1907/2006 (the REACH Regulation). This information has not been reviewed or verified by the Agency or any other authority. The content is subject to change without prior notice.
Reproduction or further distribution of this information may be subject to copyright protection. Use of the information without obtaining the permission from the owner(s) of the respective information might violate the rights of the owner.
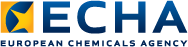