Registration Dossier
Registration Dossier
Data platform availability banner - registered substances factsheets
Please be aware that this old REACH registration data factsheet is no longer maintained; it remains frozen as of 19th May 2023.
The new ECHA CHEM database has been released by ECHA, and it now contains all REACH registration data. There are more details on the transition of ECHA's published data to ECHA CHEM here.
Diss Factsheets
Use of this information is subject to copyright laws and may require the permission of the owner of the information, as described in the ECHA Legal Notice.
EC number: 220-239-6 | CAS number: 2682-20-4
- Life Cycle description
- Uses advised against
- Endpoint summary
- Appearance / physical state / colour
- Melting point / freezing point
- Boiling point
- Density
- Particle size distribution (Granulometry)
- Vapour pressure
- Partition coefficient
- Water solubility
- Solubility in organic solvents / fat solubility
- Surface tension
- Flash point
- Auto flammability
- Flammability
- Explosiveness
- Oxidising properties
- Oxidation reduction potential
- Stability in organic solvents and identity of relevant degradation products
- Storage stability and reactivity towards container material
- Stability: thermal, sunlight, metals
- pH
- Dissociation constant
- Viscosity
- Additional physico-chemical information
- Additional physico-chemical properties of nanomaterials
- Nanomaterial agglomeration / aggregation
- Nanomaterial crystalline phase
- Nanomaterial crystallite and grain size
- Nanomaterial aspect ratio / shape
- Nanomaterial specific surface area
- Nanomaterial Zeta potential
- Nanomaterial surface chemistry
- Nanomaterial dustiness
- Nanomaterial porosity
- Nanomaterial pour density
- Nanomaterial photocatalytic activity
- Nanomaterial radical formation potential
- Nanomaterial catalytic activity
- Endpoint summary
- Stability
- Biodegradation
- Bioaccumulation
- Transport and distribution
- Environmental data
- Additional information on environmental fate and behaviour
- Ecotoxicological Summary
- Aquatic toxicity
- Endpoint summary
- Short-term toxicity to fish
- Long-term toxicity to fish
- Short-term toxicity to aquatic invertebrates
- Long-term toxicity to aquatic invertebrates
- Toxicity to aquatic algae and cyanobacteria
- Toxicity to aquatic plants other than algae
- Toxicity to microorganisms
- Endocrine disrupter testing in aquatic vertebrates – in vivo
- Toxicity to other aquatic organisms
- Sediment toxicity
- Terrestrial toxicity
- Biological effects monitoring
- Biotransformation and kinetics
- Additional ecotoxological information
- Toxicological Summary
- Toxicokinetics, metabolism and distribution
- Acute Toxicity
- Irritation / corrosion
- Sensitisation
- Repeated dose toxicity
- Genetic toxicity
- Carcinogenicity
- Toxicity to reproduction
- Specific investigations
- Exposure related observations in humans
- Toxic effects on livestock and pets
- Additional toxicological data

Phototransformation in air
Administrative data
Link to relevant study record(s)
- Endpoint:
- phototransformation in air
- Type of information:
- calculation (if not (Q)SAR)
- Remarks:
- Migrated phrase: estimated by calculation
- Adequacy of study:
- key study
- Study period:
- 2005-09-21 to 2005-09-25
- Reliability:
- 1 (reliable without restriction)
- Rationale for reliability incl. deficiencies:
- other: The calculation follows the SETAC Europe guideline recommendation (1995) and is in accordance with EU Directive 95/36/EEC.
- Principles of method if other than guideline:
- Guideline: Not applicable
The calculation of the substance decay rate in air was performed using the Atmospheric Oxidation Program, AOPWIN, software package v1.8 (Syracuse Research Corporation). The calculation follows the SETAC Europe guideline recommendation (1995) and is in accordance with EU Directive 95/36/EEC. - GLP compliance:
- no
- DT50:
- 14.35 h
- Validity criteria fulfilled:
- not applicable
- Remarks:
- The calculation follows the SETAC Europe guideline recommendation (1995) and is in accordance with EU Directive 95/36/EEC.
- Conclusions:
- The model substance 2-methyl-2H-isothiazol-3-one (MIT) is predicted to be rather efficiently removed from air by OH radical reactions during daylight situations, whereas at night the decay will predominantly be determined by reactions with ozone, however, at a much lower rate.
- Endpoint:
- phototransformation in air
- Type of information:
- (Q)SAR
- Adequacy of study:
- key study
- Study period:
- 30 Oct 2002 - 15 may 2003
- Reliability:
- 2 (reliable with restrictions)
- Rationale for reliability incl. deficiencies:
- other: The study was conducted according to standard SAR method and GLP was not necessary. The report contains sufficient data for interpretation of study results
- Qualifier:
- no guideline followed
- Principles of method if other than guideline:
- Structure Activity Relationship was used to estimate photodegradation
- GLP compliance:
- no
- Remarks:
- Not applicable
- Specific details on test material used for the study:
- Details on properties of test surrogate or analogue material (migrated information):
Not applicable - Estimation method (if used):
- Structure-Activity Relationships (SAR) Method
OH radical reaction (Atkinson, 1987)
SAR utilizes the fact that a number of separate radical reaction processes occur and that they can be dealt with individually. These processes involve: a) hydrogen atom abstraction from C-H bonds in alkanes, carbonyls, and other saturated organics; b) radical addition of >C=C< and -C=C-(triple bond) unsaturated bonds; c) radical addition to aromatic rings and d) radical interaction with -NH2, >NH, >N-, -SH, and -S- groups, etc.
The total radical reaction rate constant is then given by:
k(total) = k (hydrogen atom abstraction from C-H bonds) + k(radical addition to >C=C< and -C=C- (triple bonds) bonds) + k(radical addition to aromatic rings) + k(radical interaction with -NH2, >NH, >N-, -SH, and -S- groups)....................................................................................................eq. (1)
NO3 radical reaction (Sabljic and Gusten, 1990)
Unlike OH radical reaction, little is known about the reaction mechanism of NO3 radicals with organic compounds. Since no database with NO3 radical reactions is available, the rate constants for reactions of organic compounds with NO3 radicals are mostly estimated by correlations between kNO3 and kOH. Sabljic and Gusten conducted a survey of the literature which yielded 58 gas phase reaction rate constants for the reaction of the NO3 radical with organic compounds. From the good linear correlation, they concluded that both radicals react in a very analogous manner with organic compounds and the rate constants can be described by a correlation:
-log kNO3 = -18.86 + 3.05 x (-log kOH).................................................................................................................................................................................eq. (2)
Global average concentration of OH and NO3 radicals (Crutzen, 1982; Finlayson-Pitts and Pitts, 1982)
In the troposphere, the important direct sources of OH radicals are from the reaction of O atoms, formed from the photodissociation of O3, with water (Crutzen):
O3 + hv (lambda =319 nm) ->O(1D) +O2
O(1D) + H2O ->2OH
and from the photodissociation of HONO:
HONO + hv(lambda = 400 nm) ->OH +NO
On the other hand, the important sources of NO3 radicals are from the following reactions (Finlayson-Pitts and Pitts):
HNO3 + hv(lambda = 266 nm) -> O + HNO2 -> H + NO3, and
N2O5<-->NO3 + NO2
Phototransformation half-lives (Sabljic and Gusten, 1990)
By assuming a pseudo first-order behavior of radical reaction in the troposphere, the tropospheric half-file of a chemical compound can be calculated as:
t1/2 = ln 2/(k[C])............................................................................................................................................................................................................................eq. (3)
Where [C] is the concentration of the radicals in the troposphere such as OH, NO3, etc.
References:
Atkinson, R. A structure-activity relationship for the estimation of rate constants for the gas-phase reactions of OH radicals with organic compounds. International Journal of Chemical Kinetics, vol 19, 799-828, 1987
Crutzen, P.J. The global distribution of hydroxyl. Atmospheric Chemistry, ed., E.D. Goldberg, pp. 313-328, 1982
Finlayson-Pitts, B.J. and Pitts, Jr., J.N. Atmospheric Chemistry: fundamentals and experimental techniques, John Willy & Sons, 1982.
Sabljic, A. and Gusten, H. Predicting the night-time NO3 radical reactivity in the troposphere. Atmospheric Environment, vol. 24A, No.1, pp 73-78, 1990
- DT50:
- ca. 13 h
- Results with reference substance:
- Not applicable
- Validity criteria fulfilled:
- yes
- Conclusions:
- RH-573 has a short half-life of about 13 hours and is expected to be quickly photodegraded in the troposphere. Potential metabolites are also very reactive towards photodegradation with half-lives ranging from 19 to 25 hours.
- Executive summary:
Phototransformation rate constants and half-lives of RH-573 was calculated using a SAR (Structure-Activity Relationships) method. The calculation shows that it has a short half-life of about 13 hours and is expected to be quickly photodegraded in the troposphere. Potential metabolites are also very reactive towards photodegradation with half-lives ranging from 19 to 25 hours. Due to very small annual production volume of isothiazolone products, their effect on global warming is negligible.
Referenceopen allclose all
The reaction with hydroxyl radicals dominates the decay of MIT during daylight while at night the much slower decay due to ozone may dominate:
The half-life and rate constant for the photochemical oxidative degradation of MIT in air via the hydroxyl reaction was estimated to be 4.78 hours and 26.84 x 10E–12 cm3 molecule-1 s-1, respectively, based on 1.5 x 10E6 OH radicals per cm3 which is considered to be a representative value for daylight hours (12 hours per day). The MIT half-life of 4.78 hours is equivalent to 0.399 daylight periods (12 h day). During night-time, OH radical concentrations will be much lower and hence the decay rate will decrease correspondingly.
Similarly, the half life and rate constant for the photochemical oxidative degradation of MIT in air via the ozone reaction was estimated to be 157 hours and 0.175 x 10E17cm3 molecule-1 s-1, respectively, based on 7 x 10E11 mol ozone/cm3 (=55 µg ozone/m3) which is considered to be a representative value for ozone over a 24 hours period. The MIT half-life of 157 hours is equivalent to 6.549 days (24 h day).
The half-life of MIT for the reaction with OH-radicals taking into account the concentration of OH-radicals in atmosphere of 5 x 10E5 molecules cm-3 as recommended in the TGD (2203) is equivalent to 14.35 hours or 1.196 days (12-h day)
RH-573
Due to relatively low vapor pressure and high water solubility, the concentration of isothiazolone compounds in the trophosphere is expected to be low. This ensures that the photodegradation of radicals with these compounds obeys first-order kinetics.
Phototransformation rate constants related to individual bonds of RH-573 are listed in Table 1 for OH radical reaction (Atkinson, 1987). The largest values belong to those that are related to nitrogen and sulfur type of bonds.
According to Atkinson(1986), the gas phase reactions of OH radical with the thiols (RSH), sulfides (RSR'), and amines (RNH2, RR'NH, and RR'R"N) proceed via initial OH radical addition to the S- and N- atoms. For the thiols and sulfides, the OH radical reaction proceeds via OH radical addition to the S-atom. For the amines, the majority of the initial OH radical reaction proceeds via OH radical addition to the N-atom, followed by a number of decomposition reaction of the adduct leading to products.
Table 1 OH rate constant of different type of reactions (10 -13 cm3/molecule/sec
Bond Type | kOH | Number of Bonds, RH-573 |
C-H | 0.14 | 5 |
>C=C< | 11.0 | 1 |
>C=O | 0.31 | 2 |
>N- | 60.2 | 3 |
-S- | 20.0 | 2 |
Phototransformation rate constants of OH and NO3 radical reactions of isothiazolone compounds are calculated using equation (1) and equation (2), as shown in Table 2. kOH is about 1000 fold of kNO3.
Table 2. Phototransformation rate constants of OH and NO3 radicals (10 -13 cm3/molecule/sec)
Rate constant | RH-573 |
kOH | 232.6 |
kNO3 | 0.268 |
Table 3 shows the global average OH and NO3 radical concentrations in daylight and night hours. NO3 radical concentration is about 400 times of that of OH radical.
Table 3. Global average concentration (molecule/cm3)
Day light (Hewitt and Harrison) | Night hours (Sabljic and Gursten) | |
OH radical | 6.5 x 105 | |
NO3 radical | 2.4 x 108 |
The calculation of phototransformation half-lives (both OH and NO3 radical reactions) is performed by using equations (3) and the results are listed in Table 4. OH radical reactions are dominant photodegradation process with shorter half-lives than NO3 radical reactiosn (13 hrs vs 29 hrs). Comparing to photodegradation in water, the half lives of RH-573 in troposphere (12.7) is much shorter than those in water (11 days, See Section 5.1.3).
Table 4. Photodegradation half-life of RH-573
Hours | RH-573 |
t1/2(OH) | 12.7 |
t1/2(NO3) | 29.9 |
Metabolites
SAR calculations are conducted for all potential metabolites using Equations 1 and 3 (Table 5). Since the metabolites do not have the unstable isothiazoline ring, they will be more stable than the parent compounds. However, since all potential metabolites have either S and/or N type bonding, they are still very reactive towards tropospheric photodegradation with half-lives ranging 19.4 - 24.4 hours.
Table 5. Potential RH-573 metabolites
Potential RH-573 Metabolites | kOH (10 -13 cm3/molecule/s | t1/2 (hour) |
CH3NHC(O)CH=CHSO3H |
152.72 | 19.4 |
CH3NHC(O)CHHC(O)SO2H | 142.03 | 20.9 |
CH3NHC(O)CHHCOOH | 122.03 | 24.3 |
CH3NHC(O)COOH | 121.44 | 24.4 |
CH3NHC(O)CH3 | 121.86 | 24.3 |
CH3NHCOOH | 121.13 | 24.4 |
Description of key information
The photochemical oxidative degradation half-life of MIT in air was estimated using the Atmospheric Oxidation Program AOPWIN. The reaction with hydroxyl radicals dominates the decay of MIT during daylight while at night the much slower decay due to ozone may dominate. The half-life and rate constant for the photochemical oxidative degradation of MIT in air via the hydroxyl
reaction was estimated to be 4.78 hours and 26.84 x 10E–12 cm3 molecule-1 s-1, respectively, based on 1.5 x 10E6 OH radicals per cm3 which is considered to be a representative value for daylight hours (12 hours per day). The MIT half-life of 4.78 hours is equivalent to 0.399 daylight periods (12 h day). During night-time, OH radical concentrations will be much lower and hence the decay rate will decrease correspondingly. Similarly, the half life and rate constant for the photochemical oxidative degradation of MIT in air via the ozone reaction was estimated to be 157 hours and 0.175 x 10E17cm3 molecule-1 s-1, respectively based on 7 x 10E11 mol ozone/cm3 (=55 μg ozone/m3) which is considered to be a representative value for ozone over a 24 hours period. The MIT half-life of 157 hours is equivalent to 6.549 days (24 h day). The half-life of MIT for the reaction with OH-radicals taking into account the concentration of OH-radicals in atmosphere of 5 x 10E5 molecules cm-3 as recommended in the TGD (2203) is equivalent to 14.35 hours or 1.196 days (12-h day).
Key value for chemical safety assessment
- Half-life in air:
- 14.35 h
- Degradation rate constant with OH radicals:
- 26 840 000 000 000 000 000 cm³ molecule-1 s-1
Additional information
Information on Registered Substances comes from registration dossiers which have been assigned a registration number. The assignment of a registration number does however not guarantee that the information in the dossier is correct or that the dossier is compliant with Regulation (EC) No 1907/2006 (the REACH Regulation). This information has not been reviewed or verified by the Agency or any other authority. The content is subject to change without prior notice.
Reproduction or further distribution of this information may be subject to copyright protection. Use of the information without obtaining the permission from the owner(s) of the respective information might violate the rights of the owner.
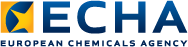