Registration Dossier
Registration Dossier
Data platform availability banner - registered substances factsheets
Please be aware that this old REACH registration data factsheet is no longer maintained; it remains frozen as of 19th May 2023.
The new ECHA CHEM database has been released by ECHA, and it now contains all REACH registration data. There are more details on the transition of ECHA's published data to ECHA CHEM here.
Diss Factsheets
Use of this information is subject to copyright laws and may require the permission of the owner of the information, as described in the ECHA Legal Notice.
EC number: 943-098-9 | CAS number: -
- Life Cycle description
- Uses advised against
- Endpoint summary
- Appearance / physical state / colour
- Melting point / freezing point
- Boiling point
- Density
- Particle size distribution (Granulometry)
- Vapour pressure
- Partition coefficient
- Water solubility
- Solubility in organic solvents / fat solubility
- Surface tension
- Flash point
- Auto flammability
- Flammability
- Explosiveness
- Oxidising properties
- Oxidation reduction potential
- Stability in organic solvents and identity of relevant degradation products
- Storage stability and reactivity towards container material
- Stability: thermal, sunlight, metals
- pH
- Dissociation constant
- Viscosity
- Additional physico-chemical information
- Additional physico-chemical properties of nanomaterials
- Nanomaterial agglomeration / aggregation
- Nanomaterial crystalline phase
- Nanomaterial crystallite and grain size
- Nanomaterial aspect ratio / shape
- Nanomaterial specific surface area
- Nanomaterial Zeta potential
- Nanomaterial surface chemistry
- Nanomaterial dustiness
- Nanomaterial porosity
- Nanomaterial pour density
- Nanomaterial photocatalytic activity
- Nanomaterial radical formation potential
- Nanomaterial catalytic activity
- Endpoint summary
- Stability
- Biodegradation
- Bioaccumulation
- Transport and distribution
- Environmental data
- Additional information on environmental fate and behaviour
- Ecotoxicological Summary
- Aquatic toxicity
- Endpoint summary
- Short-term toxicity to fish
- Long-term toxicity to fish
- Short-term toxicity to aquatic invertebrates
- Long-term toxicity to aquatic invertebrates
- Toxicity to aquatic algae and cyanobacteria
- Toxicity to aquatic plants other than algae
- Toxicity to microorganisms
- Endocrine disrupter testing in aquatic vertebrates – in vivo
- Toxicity to other aquatic organisms
- Sediment toxicity
- Terrestrial toxicity
- Biological effects monitoring
- Biotransformation and kinetics
- Additional ecotoxological information
- Toxicological Summary
- Toxicokinetics, metabolism and distribution
- Acute Toxicity
- Irritation / corrosion
- Sensitisation
- Repeated dose toxicity
- Genetic toxicity
- Carcinogenicity
- Toxicity to reproduction
- Specific investigations
- Exposure related observations in humans
- Toxic effects on livestock and pets
- Additional toxicological data

Endpoint summary
Administrative data
Link to relevant study record(s)
Description of key information
Toxicokinetic Assessment Single Wall Carbon Nanotubes (SWCNT)
Introduction
Single walled carbon nanotubes (SWCNT) are a specific form of elemental carbon, being one-layer graphene cylinders of 1 – 2 nm in diameter and typically 1 – 10 micrometer in length. Due to the chemical structure and size, carbon nanotubes (CNT) exhibit unique physicochemical properties that make them promising nanoparticles for technical uses (re-enforcement, electrical conductivity, etc.), but also for medical use as diagnostic agents, drug and DNA plasmid transporters to target cells, and for cancer therapy.
Therefore, in recent years extensive research has been globally performed, investigating especially metabolic actions to degrade such SWCNTs in vitro and in vivo.
Substance identification Tuball™
EC number: 943-098-9
Chemical name: Single Wall Carbon Nanotubes (SWCNT)
Molecular formula: Cx
Structure: elongated tubes with a diameter of ~ 1.6 nm and a length of 1 – 10 µm
Dustiness: DIRM: 1.66 * 103 mg/kg; DIRN: 1.46 * 105 mg-1
Water solubility: absolutely insoluble
Absorption
Generally, absorption of SWCNT from the intestinal is expected to occur only to a very minor extent (assumed <1%), which is supported by the fact that no treatment specific effects were seen in a Combined Repeated Dose Toxicity Study with the Reproduction/Developmental Toxicity Screening Test of Tuball™ in Rats - Administration via Diet - according to OECD Guideline 422 (B. Hansen, 2019) up to doses of >1000 mg/kg bw/d, neither in males nor in females. However, as dark coloured faeces were observed in the high dose group as of the fourth day of dosing, the excretion of non-absorbed material was shown.
As in an “In vitro EPIDERM™ Skin Corrosion Test according to OECD 431” (N. Warren, 2016a), a “Determination of Skin Irritation Potential using the EPISKIN™ Reconstructed Human Epidermis Model according to OECD 439” (N. Warren, 2016b) and in an in vivo “Assessment of Sensitising Properties on Albino Guinea Pigs by Repeated Applications; Buehler Test with 3 Applications according to OECD 406 study” (F. Richeux, 2016), all performed with Tuball™ SWCNT, no observation were made that would indicate any interaction with reconstructed human epidermis or with guinea pig skin, it can be assumed that penetration of skin and subsequent dermal exposure is not relevant (assumed being < 1%).
Absorption by exposure via the inhalation route is also assumed low (<1%) as the substance is extremely difficult to get airborne as an aerosol. When trying to perform a repeated dose sub-chronic toxicity study via inhalation, all attempts to create a respirable and stable aerosol/dust atmosphere failed, as the SWCNT clocked up each and every equipment that was tried (six different setups were investigated for suitability) (L. Ma-Hock, 2019). Additionally, the dustiness of Tuball™ was measured and found being more than 1 – 2 orders of magnitude lower than other carbon nanotubes measured and reported in public literature. This confirms that dustiness and ability to form aerosols of Tuball™ is rather limited and thus, inhalation exposure may be assumed to be significantly lower than for other carbon nanomaterials.
Distribution
If marginal amounts of SWCNT are absorbed or entering body fluids, distribution is assumed to take place mainly via blood. As SWCNT are investigated in medical application as carrier for drug (as drug delivery system) the biodistribution of single-walled carbon nanotubes in rats was investigated (Z. Lin et al., 2014) by using the iodogen oxidative method to synthesize iodinated single-walled carbon nanotubes and applying 125I-labelled SWCNT (a single dose of 100 μl) by intratracheal instillation. Animals were sacrificed and investigated 2, 4, 8, 24 and 72 hours after dosing. More than 99% of radioactivity remained in the trachea and based on total radioactivity concentration decreasing concentrations were found in the other organs/fluids as follows: trachea >> urine > stomach > small intestine > serum > bladder > blood vessel > kidney > liver > lung > adrenal > femoral head > spleen > testis > thymus > thyroid > heart > fat > muscle > brain. The total administered radioactivity was located mainly in the trachea (>99%, supporting low absorption, see above). Compared to the 2 h group, the radioactivity distribution for the 24 h group was significantly increased, and this was not significantly reduced after 72 h. The radioactivity distribution in serum and blood vessels for the 2 h group increased quickly within 8 h without an obvious decrease, but levels were distinctly depressed after 24 h. The low radioactivity detected in the thyroid gland, the major target organ of iodine, indicates that the tracer is stable and only a very small amount of 125I is lost from the SWCNTs. The biodistribution data of 125I-SWCNTs is also good evidence showing that the 125I-labelling method is reliable for tracing SWCNTs in vivo. The total recovery of the experiment was about 80%. The data showed that clearing mechanisms, resulting in highest amounts found in urine, stomach and small intestine, functioned efficiently for iodine marked SWCNT and thus the substance as such does not bioaccumulate in tissue. Although, functionalisation of SWCNTs by the iodogen oxidative method also alters the surface and composition of SWCNT, there are no alternative methods available currently, to investigate SWCNT in vivo by any other means such as radiolabelled carbon methods, and the investigation of iodine labelled SWCNTs remains the best method so far, showing that SWCNTs are non-bioaccumulative in rodents.
Metabolism
In light of potential use of SWCNTs as potential carriers for drugs in medicinal applications for the future, the biodegradation in tissue has been extensively investigated, mainly during the last ten years and describing all publications and state of knowledge is almost impossible, due to the vast number of publications. However, a focus in research has been on the oxidative degradation of SWCNT in vitro and in biota, clarifying and identifying several modes of action for tissue clearance from SWCNTs. Thus, only the main mechanisms for clearance / metabolism published so far shall be addressed here.
V.E. Kagan et al., 2008, already had reported about the biodegradation of single-walled carbon nanotubes through natural, enzymatic catalysis. They could show increased degradation of nanotube structure by incubating nanotubes with a natural horseradish peroxidase (HRP) and low concentrations of H2O2(∼40 µM) at 4 °C over 12 weeks under static conditions. By observing distinct SWCNT characteristic Raman band over weeks in their enzymatic system investigated, they could show that these Raman bands fully disappeared within 16 weeks.
In an article by V.E. Kagan et al., 2010 it was reported that hypo-chlorite and reactive radical intermediates of the human neutrophil enzyme myeloperoxidase (MPO) catalyse the biodegradation of single-walled carbon nanotubes in vitro in neutrophils and to a lesser degree in macrophages. Molecular modelling suggests that interactions of basic amino acids of the enzyme with the carboxyls on the carbon nanotubes position the nanotubes near the catalytic site. Importantly, the biodegraded nanotubes do not generate an inflammatory response when aspirated into the lungs of mice.
The comparison of the ability of various peroxidases to degrade SWNTs in vitro revealed that both myeloperoxidase due to its ability to produce hypochlorite, and lactoperoxidase due to its ability to produce hypobromite, are extremely efficient in the degrading of carbon nanotubes. The biodegradation of SWCNTs in the model system can also be induced by free radicals generated as a result of heme degradation and, to a lesser extent, by active oxoferryl intermediates of peroxidases. Experiments reported by I.I. Vlasova et al., 2011 showed that in the presence of blood plasma, peroxidase intermediates or free radical products of heme degradation were unable to initiate biodegradation of carbon nanotubes, but only the generation of hypochlorite by MPO can cause the biodegradation of carbon nanotubes in vivo. At high concentrations, hypochlorite caused a decrease in optical absorbance of plasma-containing SWCNTs suspension, which is indicative of the nanotube degradation. The results suggested that hypochlorite can serve as a main oxidizing agent to modify and degrade nanotubes at the sites of inflammation and in phagosomes.
Biodegradation of SWCNT was also proven by a range of methods including transmission electron microscopy, UV-visible-NIR spectroscopy, Raman spectroscopy, and confocal Raman imaging by V.E. Kagan et al., 2013, investigating that carbon nanotubes were degraded over time by adding human eosinophil peroxidase (hEPO) and H2O2 to SWCNT suspensions, and the SWCNTs suspension turned translucent after 96 h. Neither hEPO alone nor H2O2 alone caused degradation of SWCNTs. This was also supported by C. Farrera et al., 2014.
V.E. Kagan et al., 2014 used chemical, biochemical methods, as well as cell-based assays and animal models and demonstrated oxidative biodegradation of oxidatively functionalized single-walled carbon nanotubes via super-oxide/NO● àperoxynitrite-driven oxidative pathways of activated macrophages facilitating clearance of nanoparticles from the lung. As macrophage NADPH oxidase is the major generator of superoxide radicals required for production of peroxynitrite involved in the SWCNT biodegradation comparative measurements on days 7 and 28 were performed. It was shown that carbonaceous nanoparticles may be taken up by macrophages and “oxidatively digested” by peroxynitrite generated in activated macrophages via NADPH-oxidase-driven production of superoxide anion radicals and NO●. It was also considered likely that clearance of SWCNTs from the lung might be due to the enhanced macrophage transport. This work was also supported by Y. Ding et al., 2017, who investigated more the dependence on activation by NADPH oxidase, supporting this process.
M Yang, et al., 2019 investigated macrophage cell line RAW 264.7 and primary rat Kupffer cells for uptake of SWCNTs as well as their quantity changes up to 7 days later by measuring optical absorbance in the near infrared region and Raman spectra of SWCNTs in the cell lysates. SWCNTs were degraded by approximately 25 – 30% within first 4 days after uptake; however, no additional degradation occurred for the remainder of the 7-day test period. Generation of reactive oxygen species (ROS) by macrophages decreased as CNT degradation occurred, returning to control levels by Day 7. In the meantime, the glutathione level gradually recovered over time. There were no changes in cell viability or caspase 3/7 activation during SWCNT degradation. These results confirm degradation of SWCNTs by macrophages, associated with reactive oxygen species generation and also suggest that CNT cytotoxicity decreases in line with degradation.
M. Zhang et al., 2019 (see also M. Zhang et al., 2018) have investigated and compared the biodegradation by 1.1% sodium hypochlorite pentahydrate (NaClO·5H2O) at 37 °C of different carbon nanotubes (single wall SWCNT, carbon nanohorns CNH, multi wall MWCNT and thicker multi wall tMWCNT) to CO2 ultimately, and could show that half-lifes increased in the order SWCNT < CNH < MWCNT < tMWNT, whereas SWCNT were completely degraded within 24 hours (half-lifes of 0.22 – 1.75 h; four different SWCNTs with diameters of 0.7 – 5 nm investigated). The theory behind this is that the biodegradation of CNTs by neutrophils or macrophages is mediated by the oxidation of the CNTs by hypochlorite (ClO−), which is generated as a by-product of various enzymatic reactions and thus this investigation simulated the biodegradation in vitro.
In a recent study (R. El-Sayed et al., 2019) the biodegradation of single-walled carbon nanotubes (SWCNTs) by Cytochrome P450 enzymes (CYP3A4) by using Raman spectroscopy was shown. CYP3A4 is a known isozyme accountable for metabolizing various endogenous and exogenous xenobiotics. CYP3A4 is expressed dominantly in the liver and other organs including the lungs. The results suggest that CYP3A4 has a higher affinity for SWCNTs compared to chemically oxidized SWCNTs (c-SWCNT). HEK293 cellular viability was not compromised when incubated with SWCNT. However, a CYP3A4 transfected HEK293 cell line showed no digestion of c-SWCNTs after incubation for 96 h. Cellular uptake of c-SWCNTs was observed by electron microscopy and localization of c-SWNTs was confirmed in endosomal vesicles and in the cytoplasm. This work was supported by molecular dynamic simulations suggesting that CYP3A4 degrades SWCNTs more efficiently than c-SWCNTs as SWCNTs are more energetically favorable to bind to the active site of the CYP3A4. Thus, CYP3A4 may play an important role in the clearance of SWCNT materials in living organisms (compare also M. Chen et al., 2017).
In summary, different enzymatic catalytic pathways for degradation of CNTs were discovered and characterized in recent work using heme peroxidases such as horseradish peroxidase (HRP), myeloperoxidase (MPO) and eosinophil peroxidase (EPO) and meanwhile several oxidative mechanisms have been identified showing that SWCNTs can be biodegraded by MPO/H2O2, MPO/H2O2/Cl−, OCl− and ONOO−, as well as others. Thus, enzyme catalysed oxidative pathways have been proven in vitro and in vivo, to be capable to biodegrade SWCNT ultimately to CO2 and it can be assumed that such mechanisms not only take place in rodents but also in biota such as the aquatic environment.
Excretion
As shown by Z. Lin et al. (2014) the highest amount of labelled material that was absorbed via inhalation exposure (more than 99% remained unabsorbed in the trachea) was found in urine and thus clearance via urine appears an efficient excretion route for absorbed SWCNTs.
The main route for clearance from the body following oral uptake is via faeces, as was seen in a Combined Repeated Dose Toxicity Study with the Reproduction/Developmental Toxicity Screening Test of Tuball™ in Rats - Administration via Diet - according to OECD Guideline 422 (B. Hansen, 2019), in which no changes in the consistency of the faeces were noted for the male and female animals of the control group and the treatment groups (100, 300 or 1000 mg Tuball™/kg bw/day). However, dark discoloured faeces were noted continuously from test day 18 (4 days after the start of dosing) until the end of the study for all male and female animals of the high dose group. This was due to the high concentration of the administered test item (black powder) and showed that the main elimination route from the body by oral (via feed) administration is via faeces. To a minor extent, also exhalation of CO2, finally resulting from biodegradation may play a role, but so far there is no evidence reported.
Literature:
- M. Chen et al., 2017 Understanding enzymatic degradation of single-walled carbon nanotubes triggered by functionalization using molecular dynamics simulation; M. Chen et al., 2017, Environ. Sci.: Nano, 2017, 4, 720
- Y. Ding et al., 2017 NADPH oxidase-dependent degradation of single-walled carbon nanotubes in macrophages; Yun Ding et al.; J Mater Sci: Mater Med (2017) 28:7
- C. Farrera et al., 2014 Extracellular entrapment and degradation of single-walled carbon nanotubes; Consol Farrera et al.; Nanoscale, 2014, 6, 6974
- B. Hansen, 2019 Combined Repeated Dose Toxicity Study with the Reproduction/Developmental Toxicity Screening Test of Tuball™ in Rats - Administration via Diet - according to OECD Guideline 422
- V.E. Kagan et al., 2008 Biodegradation of Single-Walled Carbon Nanotubes through Enzymatic Catalysis; V.E. Kagan et al.; NANO LETTERS 2008 Vol. 8, No. 11, 3899-3903
- V.E. Kagan et al., 2010 Carbon nanotubes degraded by neutrophil myeloperoxidase induce less pulmonary inflammation; Valerian E. Kagan et al.; Nature nanotechnology, online published 2010
- V.E. Kagan et al., 2013 Biodegradation of Single-Walled Carbon Nanotubes by Eosinophil Peroxidase; Valerian E. Kagan et al.; small 2013, 9, No. 16, 2721–2729
- V.E. Kagan et al., 2014 Lung Macrophages “Digest” Carbon Nanotubes Using a Superoxide/Peroxy-nitrite Oxidative Pathway; ACSNano, Vol. 8, No. 6, 5610 – 5621, 2014
- Z. Lin et al., 2014 Biodistribution of single-walled carbon nanotubes in rats; Z. Lin et al.; Toxicol. Res., 2014, 3, 497
- L. Ma-Hock, 2019 TUBALL™ Technical trial to generate dust atmosphere appropriate for subsequent repeated-dose inhalation studies
- F. Richeux, 2016 Tuball™Assessment of Sensitising Properties on Albino Guinea Pigs by Repeated Applications Buehler Test with 3 Applications according to OECD 406
- R. El-Sayed et al., 2019 Degradation of pristine and oxidized single wall carbon nanotubes by CYP3A4; Biochemical and Biophysical Research Communications 515 (2019) 487 – 492
- I.I. Vlasova et al., 2011 Myeloperoxidase-Induced Biodegradation of Single-Walled Carbon Nanotubes Is Mediated by Hypochlorite; I.I. Vlasova et al.; Russian Journal of Bioorganic Chemistry, 2011, Vol. 37, No. 4, pp. 453–463.
- N. Warren, 2016a Tuball™: In vitro EPIDERM™ Skin Corrosion Test according to OECD 431
- N. Warren, 2016b Tuball™: Determination of Skin Irritation Potential using the EPISKIN™ Reconstructed Human Epidermis Model according to OECD 439
- M. Yang et al., 2019 Time-dependent degradation of carbon nanotubes correlates with decreased reactive oxygen species generation in macrophages; Mei Yang et al., 2019, International Journal of Nanomedicine 2019:14 2797–2807
- M. Zhang et al., 2018 Size-dependent cell uptake of carbon nanotubes by macrophages: A comparative and quantitative study; Minfang Zhang et al.; Carbon 127 (2018) 93 – 101 and Quantification of Carbon Nanotubes Taken up by Macrophage Cells Using Optical Absorption Method; Minfang Zhang et al.; e-J. Surf. Sci. Nanotech. Vol. 16 (2018) 93 - 96
- M. Zhang et al., 2019 Diameter-Dependent Degradation of 11 Types of Carbon Nanotubes: Safety Implications; M. Zhang et al., 2019, ACS Appl. Nano Mater. 2019
Key value for chemical safety assessment
- Bioaccumulation potential:
- low bioaccumulation potential
- Absorption rate - oral (%):
- 1
- Absorption rate - dermal (%):
- 1
- Absorption rate - inhalation (%):
- 1
Additional information
Information on Registered Substances comes from registration dossiers which have been assigned a registration number. The assignment of a registration number does however not guarantee that the information in the dossier is correct or that the dossier is compliant with Regulation (EC) No 1907/2006 (the REACH Regulation). This information has not been reviewed or verified by the Agency or any other authority. The content is subject to change without prior notice.
Reproduction or further distribution of this information may be subject to copyright protection. Use of the information without obtaining the permission from the owner(s) of the respective information might violate the rights of the owner.
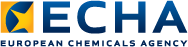