Registration Dossier
Registration Dossier
Data platform availability banner - registered substances factsheets
Please be aware that this old REACH registration data factsheet is no longer maintained; it remains frozen as of 19th May 2023.
The new ECHA CHEM database has been released by ECHA, and it now contains all REACH registration data. There are more details on the transition of ECHA's published data to ECHA CHEM here.
Diss Factsheets
Use of this information is subject to copyright laws and may require the permission of the owner of the information, as described in the ECHA Legal Notice.
EC number: 250-264-8 | CAS number: 30618-84-9
- Life Cycle description
- Uses advised against
- Endpoint summary
- Appearance / physical state / colour
- Melting point / freezing point
- Boiling point
- Density
- Particle size distribution (Granulometry)
- Vapour pressure
- Partition coefficient
- Water solubility
- Solubility in organic solvents / fat solubility
- Surface tension
- Flash point
- Auto flammability
- Flammability
- Explosiveness
- Oxidising properties
- Oxidation reduction potential
- Stability in organic solvents and identity of relevant degradation products
- Storage stability and reactivity towards container material
- Stability: thermal, sunlight, metals
- pH
- Dissociation constant
- Viscosity
- Additional physico-chemical information
- Additional physico-chemical properties of nanomaterials
- Nanomaterial agglomeration / aggregation
- Nanomaterial crystalline phase
- Nanomaterial crystallite and grain size
- Nanomaterial aspect ratio / shape
- Nanomaterial specific surface area
- Nanomaterial Zeta potential
- Nanomaterial surface chemistry
- Nanomaterial dustiness
- Nanomaterial porosity
- Nanomaterial pour density
- Nanomaterial photocatalytic activity
- Nanomaterial radical formation potential
- Nanomaterial catalytic activity
- Endpoint summary
- Stability
- Biodegradation
- Bioaccumulation
- Transport and distribution
- Environmental data
- Additional information on environmental fate and behaviour
- Ecotoxicological Summary
- Aquatic toxicity
- Endpoint summary
- Short-term toxicity to fish
- Long-term toxicity to fish
- Short-term toxicity to aquatic invertebrates
- Long-term toxicity to aquatic invertebrates
- Toxicity to aquatic algae and cyanobacteria
- Toxicity to aquatic plants other than algae
- Toxicity to microorganisms
- Endocrine disrupter testing in aquatic vertebrates – in vivo
- Toxicity to other aquatic organisms
- Sediment toxicity
- Terrestrial toxicity
- Biological effects monitoring
- Biotransformation and kinetics
- Additional ecotoxological information
- Toxicological Summary
- Toxicokinetics, metabolism and distribution
- Acute Toxicity
- Irritation / corrosion
- Sensitisation
- Repeated dose toxicity
- Genetic toxicity
- Carcinogenicity
- Toxicity to reproduction
- Specific investigations
- Exposure related observations in humans
- Toxic effects on livestock and pets
- Additional toxicological data

Specific investigations: other studies
Administrative data
Link to relevant study record(s)
Description of key information
The mortality and the signs of systemic toxic observed in the oral acute or repeated dose toxicity studies of GMT fit well with the signs observed after oral acute or repeated administration of its toxicological relevant metabolite thioglycolate (mercaptoacetate). They seem primarily linked to the inhibition of the β-oxidation of fatty acids. This inhibition induced secondary effects like a decrease of blood glucose, liver glycogen content, blood and hepatic ketone bodies and liver acetyl-CoA and an increase of plasma free fatty acids and liver triglycerides and acyl-CoA and an enhancement of hepatic pyruvate. The fatty liver induced by mercaptoacetate was mainly due to an inhibition of acyl-CoA dehydrogenase activity and consequently to a marked depression of the β-oxidation pathway. Fasted animals appeared to be more sensitive to the toxic effects than non-fasted animals.
Additional information
Thioglycolic acid (mercaptoacetic acid) is the toxicological relevant metabolite resulting from GMT after hydrolysis of the ester bonds. In the various acute or repeated dose toxicity studies (OECD # 408, 414, 416) performed by oral route with mercaptoacetic acid and/or its salts, signs of systemic toxic (including mortality), increase of food consumption and/or perturbation of some biochemical parameters related to the fatty acids oxidation were observed. It is suggested that the most probable mechanism of toxicity was linked to the inhibition of the β-oxidation of fatty acids as described below.
Effects of mercaptoacetate on glycemia
Mercaptoacetate has been proved to have an action on blood glucose regulation (Freemanet al., 1956). Respectively, six hours after i. v. (175 mg/kg bw) or i. p. (150 mg/kg bw) treatments with sodium mercaptoacetate, rabbits and rats presented a significantly reduced blood sugar concentration (> 50%), sometimes leading to death. Since the LD50greatly increases in glucose-treated rats (3.4 to 4.4 fold), it is very likely that animals died from hypoglycaemia. Five hours after i. p. treatment (0.04 ml/g of 5% glucose solution ± 630 mg/kg bw sodium mercaptoacetate), the average liver glycogen found in treated mice was significantly reduced (>70%) indicating glycogenolysis due to mercaptoacetate. There was no significant difference in muscle glycogen of treated and untreated animals (3 mL of 50% glucose solutionper os± 300 mg/kg bw sodium mercaptoacetate i. p.). In diabetic rats treated with mercaptoacetate, the water intake, the urine volume and the urinary sugar decreased, suggesting that mercaptoacetate was not acting by increasing insulin secretion. There appears to be a rise then a fall in blood sugar in diabetic rabbits after treatment with mercaptoacetate (300 mg/kg bw i. v.).
In a recent study (Davies, 2010c), not yet finalized at the time of registration, it appeared that fasted animal are more sensitive to the toxic and hypoglycemic effects of mercaptoacetate than non-fasted animals. In fasted animals, an initial rise of the glycemia is followed by a severe hypoglycemia until the re-feeding of the animals. In the non-fasted animals, no initial rise was observed and the hypoglycemia was moderate compared to fasted animals. The initial glucose rise in the fasted animals suggest that mercaptoacetate stimulate the glycogenolysis, then when the glycogene is consumed, the glycemia decreased. The administration of glucose to fasted animals prevents the blood glucose decrease. It could explain why significant effects on blood glucose, hydroxybutyrate and/or acetoacetate was observed in the 90-day oral study (Rousseau, 2010) whereas no effect was observed in the 2-generation study (Davies 2010a). In the 90-day study, the animal were fasted overnight before the blood sampling, 5-6 hours after the treatment, in the 2-generation, the animals were not fasted.
Effects of mercaptoacetate on the fatty acid oxidation and liver enzyme activity
On the basis of the published results (Nordmann and Nordmann, 1971; Sabouraultet al.,1976; Sabouraultet al.,1979; Bauchéet al.,1981; Bauchéet al.,1982; Bauchéet al.,1983.;Sabbaghet al.,1985; Schulz, 1987), there is no doubt that mercaptoacetate inhibited the hepaticβ-oxidation of fatty acids resulting in a greater conversion into triglycerides in the liver. As a result, ketogenesis was inhibited.
Intraperitoneal administrations (31 mg/kg bw and 15 mg/kg bw, 3 hours later) of 2-mercaptoethanol (which is oxidized to mercaptoacetate) causes a fatty liver, as shown by the highly significant (p<0.01) increase of liver triglycerides (2.2x), accompanied by a considerable increase of plasma free fatty acids (2.4x) and by remarkable decreases in blood ketone bodies (acetoacetate –73% and β-hydroxybutyrate –90%), 3 hours after the 2ndinjection. A very significant (p<0.01) decrease of blood glucose (-35%) and liver glycogen (-70%) content occurs at the same time(Nordmann and Nordmann, 1971; reliability 2).
It appears that mercaptoacetate administered to female Wistar rats (45 mg/kg bw, i. p.) induced an increase of hepatic triacylglycerol (2.7x at 3h and 13.7x at 24h) and blood free fatty acids (3.9x at 3h and no effects at 24h), a decrease of blood triacylglycerol (-30% at 3h and –48% at 24h) and phospholipids (-43% at 3 h and –18% at 24h) as well as a reduction in the hepatic ketone body level (acetoacetate –52% at 3h and β-hydroxybutyrate –75% at 3h and –42% at 24h). The large and early increase of blood free fatty acids reflects most probably an enhanced peripheral fat mobilization, which is an important factor in the pathogenesis of fatty liver (Sabouraultet al.,1976; reliability 2).
After mercaptoacetate administration (45 mg/kgbwi. p.) the hepatic levels of free CoA-SH and acetyl-CoA were markedly decreased, falling to c. a. 20% of the control values. At the same time, on the contrary, the hepatic acyl-CoA level was increased (+120%), an effect which did not completely balance, however, the reduction in free CoA-SH and acetyl-CoA concentrations. Moreover, 2-mercaptoacetate treatments induced both a dramatic increase (> 15x) of the hepatic pyruvate level and a significant reduction (-40%) of the blood glucose level (Sabouraultet al., 1979; reliability 2). The increase in hepatic pyruvate level could result from a direct/indirect inhibition of the mitochondrial utilization of pyruvate by mercaptoacetate.
Indeed, it has been shown, using rat hepatic mitochondria, that mercaptoacetate could be a substrate for acetyl-CoA synthase, following an ATP-dependent activation, and that the resulting compound, 2-mercaptoacetyl-CoA could inhibitnon-specifically thefatty acyl-CoA dehydrogenases (long-chaingeneralandshort-chainacyl-CoAdehydrogenases) as well as the branched-chain acyl-CoA dehydrogenase, namely isovaleryl-CoA (Bauchéet al., 1982 and Bauchéet al., 1983; Sabbaghet al., 1985).
Mercaptoacetate injected parenterally or administered by gavage, significantly depressed hepatic succinoxidase (SO) in rats, mice and rabbits, the male displaying a higher sensitivity than the adult female.In vitro, mercaptoacetate was without affect on cytochrome oxidase and diaphorase activities but depressed NADH cytochrome-c-reductase activity.In vivo, mercaptoacetatewas without action on the SO activity in spleen and brain but was inhibited in liver and kidneys of male rats. The activities of the hepatic xanthine oxidase, D-amino acid oxidase, malic oxidase, LDH and alcohol deshydrogenase as well as the activities of the renal LDH and alcohol deshydrogenase were not affected. The feeding of diets supplemented with excessive amounts of amino acids prior to the injection of mercaptoacetate, protected against the action of the thiol(Bakshy and Gershbein, 1971 and Bakshy and Gershbein, 1973).
Effects of mercaptoacetate on food consumption
The effect of mercaptoacetate on food consumption seems to depend on the age, the strain, the nutritional status and on the nature of the dietary fat.
Several experiments have shown that mercaptoacetate increased the food intake in medium- or high-fat fed animals but not in low-fat fed animals (Scharrer and Langhans, 1986). This increase was essentially due to the reduced interval between meals rather than the increase of the meals size or duration(Langhans and Scharrer, 1987). The inhibition exerted by mercaptoacetate on the β-oxidation of the fatty acids was considered to be the earliest metabolic signal modifying independent ingestion in rats (increase of the plasma free fatty acids, decrease of plasma β-hydroxybutyrate confirmed the inhibition). A long-term feeding-inhibitory effect could also be observed after mercaptoacetate administrations, probably mediated by a different mechanism than its feeding-stimulatory effect (Brandtet al., 2006).
Several hypothesis were issued to explain the control of the food intake by the β-oxidation:
· The decrease in reduced cofactors (Nordmann and Nordmann, 1971; Scharrer and Langhans, 1986) increases the food intake.
· The inhibition of the β-oxidation decreased the membrane potential in liver cell, which modulate the afferent vagal activity, stimulating the food intake (Boutellieret al., 1999). Mercaptoacetate-induced enhanced feeding in rats given fat-enriched diet does not depend on a stronger hepatic and/or celiac vagal afferent response than rats given a low-fat diet (Randichet al., 2002). In addition, the release of ketone bodies could independently act on the afferent vagal activity.
· The NMDA receptors and the neurotransmitter glutamate may be involved in processing these mecanoreceptive signals (Duvaet al., 2005).
· Some results suggest that mercaptoacetate elicits a feeding inhibitory effect in fasted rats, which could be due to an increased β-adrenergic activity. Hypothermia, increased plasma free fatty acids levels and eventually disturbances in glucose metabolism may have contributed (Brandtet al., 2006).
· Glucose deprivation does not contribute to feeding elicited by mercaptoacetate-induced inhibition of fatty acid oxidation in rats fed a carbohydrate-free, high-fat diet (Del Preteet al., 2001).
· Experiments performed on food intake in developing rats (Switherset al., 2000, Switherset al., 2001), in adequation with effects observed in adult rats, show that the action of mercaptoacetate, by blockade of fatty acid oxidation, stimulates independent ingestion and not suckling or water intake. This stimulation does not work on younger animals, 9-weeks aged old or lower. This is probably due to a compensatory system present at this period of development. In addition, mercaptoacetate has a short-term action (shorter than to the duration of β-oxidation inhibition) probably antagonized later by the disturbances of metabolite homeostasis resulting from the impairment of fatty acid oxidation. At high dose-levels of mercaptoacetate, inhibition of the food intake and gastric emptying were observed.
Information on Registered Substances comes from registration dossiers which have been assigned a registration number. The assignment of a registration number does however not guarantee that the information in the dossier is correct or that the dossier is compliant with Regulation (EC) No 1907/2006 (the REACH Regulation). This information has not been reviewed or verified by the Agency or any other authority. The content is subject to change without prior notice.
Reproduction or further distribution of this information may be subject to copyright protection. Use of the information without obtaining the permission from the owner(s) of the respective information might violate the rights of the owner.
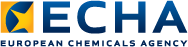