Registration Dossier
Registration Dossier
Data platform availability banner - registered substances factsheets
Please be aware that this old REACH registration data factsheet is no longer maintained; it remains frozen as of 19th May 2023.
The new ECHA CHEM database has been released by ECHA, and it now contains all REACH registration data. There are more details on the transition of ECHA's published data to ECHA CHEM here.
Diss Factsheets
Use of this information is subject to copyright laws and may require the permission of the owner of the information, as described in the ECHA Legal Notice.
EC number: 953-178-5 | CAS number: -
- Life Cycle description
- Uses advised against
- Endpoint summary
- Appearance / physical state / colour
- Melting point / freezing point
- Boiling point
- Density
- Particle size distribution (Granulometry)
- Vapour pressure
- Partition coefficient
- Water solubility
- Solubility in organic solvents / fat solubility
- Surface tension
- Flash point
- Auto flammability
- Flammability
- Explosiveness
- Oxidising properties
- Oxidation reduction potential
- Stability in organic solvents and identity of relevant degradation products
- Storage stability and reactivity towards container material
- Stability: thermal, sunlight, metals
- pH
- Dissociation constant
- Viscosity
- Additional physico-chemical information
- Additional physico-chemical properties of nanomaterials
- Nanomaterial agglomeration / aggregation
- Nanomaterial crystalline phase
- Nanomaterial crystallite and grain size
- Nanomaterial aspect ratio / shape
- Nanomaterial specific surface area
- Nanomaterial Zeta potential
- Nanomaterial surface chemistry
- Nanomaterial dustiness
- Nanomaterial porosity
- Nanomaterial pour density
- Nanomaterial photocatalytic activity
- Nanomaterial radical formation potential
- Nanomaterial catalytic activity
- Endpoint summary
- Stability
- Biodegradation
- Bioaccumulation
- Transport and distribution
- Environmental data
- Additional information on environmental fate and behaviour
- Ecotoxicological Summary
- Aquatic toxicity
- Endpoint summary
- Short-term toxicity to fish
- Long-term toxicity to fish
- Short-term toxicity to aquatic invertebrates
- Long-term toxicity to aquatic invertebrates
- Toxicity to aquatic algae and cyanobacteria
- Toxicity to aquatic plants other than algae
- Toxicity to microorganisms
- Endocrine disrupter testing in aquatic vertebrates – in vivo
- Toxicity to other aquatic organisms
- Sediment toxicity
- Terrestrial toxicity
- Biological effects monitoring
- Biotransformation and kinetics
- Additional ecotoxological information
- Toxicological Summary
- Toxicokinetics, metabolism and distribution
- Acute Toxicity
- Irritation / corrosion
- Sensitisation
- Repeated dose toxicity
- Genetic toxicity
- Carcinogenicity
- Toxicity to reproduction
- Specific investigations
- Exposure related observations in humans
- Toxic effects on livestock and pets
- Additional toxicological data

Endpoint summary
Administrative data
Link to relevant study record(s)
Description of key information
The target substance Monoesters of C16 and C18 (branched and linear) fatty acids with decan-1-ol is expected to be hydrolysed within the gastrointestinal tract. The hydrolysis products are predicted to be readily absorbed via the oral and inhalation route. Potential for absorption via the dermal route is predicted to be low. The ester bonds will be hydrolysed in the gastrointestinal tract and mucus membranes to the methyl branched isomers of C18-fatty acids and C10-fatty alcohol, which facilitates the absorption. The fatty acid will most likely be re-esterified to triglycerides after absorption and transported via chylomicrons; while the absorbed alcohol is mainly oxidised to the corresponding fatty acid and then to a triglyceride. The major metabolic pathway for linear fatty acids is the beta-oxidation pathway for energy generation. The excretion will mainly be as CO2 in expired air; with a smaller fraction excreted as conjugated molecules in the urine. Fatty alcohol can likewise be metabolised and incorporated into physiological pathways or conjugated and excreted. No bioaccumulation will take place, as excess triglycerides are stored and used as the energy need rises.
Key value for chemical safety assessment
- Bioaccumulation potential:
- no bioaccumulation potential
Additional information
Additional information
Basic toxicokinetics
In accordance with Annex VIII, Column 1, Item 8.8.1, of Regulation (EC) 1907/2006 and with Guidance on information requirements and chemical safety assessment Chapter R.7c: Endpoint specific guidance (ECHA, 2017), an assessment of the toxicokinetic behaviour of the target substance Monoesters of C16 and C18 (branched and linear) fatty acids with decan-1-ol is conducted to the extent that can be derived from the relevant available information. This comprises a qualitative assessment of the available substance specific data on physico-chemical and toxicological properties according to the Chapter R.7c Guidance document (ECHA, 2017) and taking into account further available information from a source substance. There are no studies available in which the toxicokinetic behaviour of the target substance was investigated.
The target substanceis an UVCB substance, obtained by esterification of isostearic acid, which is a complex mixture of branched and straight-chain fatty acids, with decyl alcohol.It is composed of isomers, therefore the molecular weight can be expressed as ca. 424 g/mol instead of a range. It is a liquid with a calculated vapour pressure < 0.0001 Pa 25 °C and a calculated log Pow of > 10. The water solubility was determined to be < 0.16 mg/L.
Absorption
Absorption is a function of the potential for a substance to move across biological membranes. The most useful parameters providing information on this potential are the molecular weight, the octanol/water partition coefficient (log Pow) value and the water solubility. The log Pow value provides information on the relative solubility of the substance in water and lipids (ECHA, 2017).
Oral
In general, molecular weights below 500 and log Pow values between -1 and 4 are favourable for absorption via the gastrointestinal (GI) tract, provided that the substance is sufficiently water soluble (> 1 mg/L). Lipophilic compounds may be taken up by micellar solubilisation by bile salts, but this mechanism may be of particular importance for highly lipophilic compounds (log Pow > 4), in particular for those that are poorly soluble in water (≤ 1 mg/L) as these would otherwise be poorly absorbed. Solids must be dissolved before absorption; the degree depends on the water solubility (Aungst and Shen, 1986; ECHA, 2017). The potential of a substance to be absorbed in the GI-tract may be influenced by several parameters, like: chemical changes taking place in GI-fluids, as a result of metabolism by GI-flora, by enzymes released into the GI-tract or by hydrolysis. These changes will alter the physico-chemical characteristics of the substance and hence predictions based on the physico-chemical characteristics of the parent substance may in some cases no longer apply or should be adjusted (ECHA, 2017).
In general, alkyl esters are readily hydrolysed in the GI-tract, blood and liver to the corresponding alcohol and fatty acid by the ubiquitous carboxylesterases. There are indications that the hydrolysis rate in the intestine catalysed by pancreatic lipase is lower for alkyl esters than for triglycerides, which are the natural substrates of this enzyme (Mattson and Volpenhein, 1969).
The target substance is expected to be enzymatically hydrolysed to methyl-branched isomers of C18-fatty acids and the C10-fatty alcohol.
The molecular weight of about 424 g/mol of the target substance, the log Pow > 10 and water solubility < 1 mg/L indicate poor absorption from the GI-tract following oral ingestion. Micellar solubilisation may have an effect on the overall absorption rate of the substance. Moreover, it is expected that the absorption rate of the hydrolysis products is expected to be high, so that absorption of the hydrolysis products is expected to be higher than absorption of the intact parent compounds.
In an acute oral toxicity study female mice were administered a single dose of 2000 mg/kg bw decyl oleate (CAS 3687-46-5). There was no mortality, no clinical signs of toxicity and no effect on the body weight (Key, 1994). Besides this, further acute oral toxicity studies with the source substances 2-octododecyl myristate (CAS 22766-83-2) in rats and with Fatty acids, C8-10, C12-18-alkyl esters (CAS 95912-86-0) in mice were taken into account as supporting information. In both studies the LD50 was > 2000 mg/kg bw (Supporting, 1989; Supporting, 1991). Moreover, the toxicological properties of the target substance have been evaluated in quantitative structure activity relationship (QSAR) modelling, which was performed with Biovia Discovery Studio (TOPKAT) 4.5 (extensible), US EPA TEST 4.2, DEREK NEXUS and OECD QSAR Toolbox 4.2. The results of models used suggest that it is unlikely that the target substance should be classified for acute oral toxicity and its toxicity can be assumed to be >2000 mg/kg bw with a worst-case value of 4,280 mg/kg bw (US EPA TEST 4.2).
In a combined repeated dose toxicity and reproduction/developmental toxicity screening study with 8-methylnonyl octadec-9-enoate (CAS 59231-34-4) male and female rats were administered orally doses up to 1000 mg/kg bw/day for 36 days (males) and up to 56 days (females). The NOAEL for systemic toxicity of the males was ≥ 1000 mg/kg bw/day whereas for the females, the NOAEL was set at 300 mg/kg bw/day because of the maternal toxicity observed in the high dose group (1000 mg/kg bw/day) (Key, 2013). Male and female rats were orally administered decyl oleate (CAS 3687-46-5) once daily by gavage on 5 days per week in a repeated dose 28-day oral toxicity study. No toxicologically relevant effects were noted up to and including the highest dose level of 1000 mg/kg bw/day (Supporting, 1987). All results available suggest that the test substance is of low systemic toxicity, either due to low toxicity potency or due to low absorption in combination with a low systemic toxicity.
In conclusion, the physico-chemical properties and molecular weight of the target substance suggest that some oral absorption is likely to occur. However, the substance is anticipated to undergo enzymatic hydrolysis in the GI-tract and therefore absorption of the ester hydrolysis products is also relevant. The absorption rate of the hydrolysis products is expected to be high.
Dermal
Molecular weights below 100 g/mol favour dermal uptake, while for those above 500 g/mol the molecule may be too large. Dermal uptake is anticipated to be low if the water solubility is < 1 mg/L; low to moderate if it is between 1-100 mg/L; and moderate to high if it is between 100-10000 mg/L. Log Pow values in the range of 1 to 4 (values between 2 and 3 are optimal) are favourable for dermal absorption, in particular if water solubility is high. For substances with a log Pow above 4, the rate of penetration may be limited by the rate of transfer between the stratum corneum and the epidermis, but uptake into the stratum corneum will be high. Log Pow values above 6 reduce the uptake into the stratum corneum and decrease the rate of transfer from the stratum corneum to the epidermis, thus limiting dermal absorption (ECHA, 2017).
The molecular weight of the target substance is approximately 424 g/mol does not favour dermal absorption. The low water solubility (< 1 mg/mL) and high log Pow (>10) indicate a low dermal absorption, as the uptake into the stratum corneum is predicted to be slow and the rate of transfer between the stratum corneum and the epidermis is considered to be slow as well (ECHA, 2017).
The dermal permeability coefficient (Kp) can be calculated from log Pow and molecular weight (MW) applying the following equation described in US EPA (2012), using the Epi Suite software:
log(Kp) = -2.80 + 0.66 log Pow – 0.0056 MW
Three representative constituents of the target substance were used for the prediction (please refer to the analogue justification for more details on the SMILES codes).
For the representative constituent 1 Dermwin v2.02 calculated a Kp of 529 cm/h and dermal flux rate of 1.64e-006 mg/cm²h. For the representative constituents 2 and 3 similar values were predicted: a Kp of 1.65e+003 and 1.48e+003 cm/h, respectively, and dermal flux rates of 5.96-007 and 6.16e-007 mg/cm²h, respectively. These predicted values indicate a very low dermal absorption potential of the target substance.
The indications that the target substance has low dermal absorption and/or low acute dermal toxicity are supported by the available data on source substances covering acute dermal toxicity:
An acute dermal toxicity study was performed with decyl oleate (CAS 3687-46-5), in which rats were exposed to 2000 mg/kg bw for 24 hours under occlusive conditions (Key, 2010). No mortality occurred and no toxicologically relevant systemic effects were observed. Erythema (score 1) was noted during Day 3-7 in 4/10 animals, while scales or scabs were observed in 8/10 animals for up to 9 days from Day 7 to 15. An acute dermal toxicity study was performed with 2-octyldodecyl isooctadecanoate (93803-87-3), in which rats were exposed to 2000 mg/kg bw for 24 hours under occlusive conditions (Supporting, 1998). No mortality occurred and no toxicologically relevant systemic effects were observed.
If a substance shows skin irritating or corrosive properties, damage to the skin surface may enhance penetration. If the substance has been identified as a skin sensitizer then some uptake must have occurred although it may only have been a small fraction of the applied dose (ECHA, 2017).
The available in vitro skin irritation data on the target substance showed no irritating effects in the EPISKIN™ reconstructed human epidermis model according to OECD 439 and in compliance with GLP (Key, 2011). In a local lymph node assay in mice with the source substance decyl oleate (CAS 3687-46-5) only slight erythema was seen, which was fully reversible within 3 days after the last application (WoE, 2010). In a guinea pig maximisation test with the source substance 2-octyldodecyl isooctadecanoate (CAS 93803-87-3) no erythema or edema was seen after topical challenge with the undiluted test substance for 24 hours under semi-occlusive conditions (WoE, 1998). Therefore, no relevant increase in the penetration rate of the substance due to skin damage is expected for the target substance.
Overall, based on the available information and using a worst-case approach, the dermal absorption potential of the target substance is predicted to be low.
Inhalation
Substances that can be inhaled include gases, vapours, liquid aerosols (both liquid substances and solid substances in solution) and finely divided powders/dusts. The vapour pressure indicates whether a substance may be available for inhalation as a vapour. As a general guide, highly volatile substances are those with a vapour pressure greater than 25 kPa (or a boiling point below 50°C). Substances with low volatility have a vapour pressure of less than 0.5 kPa (or a boiling point above 150°C) (ECHA, 2017).
The target substance is a liquid with a boiling point of 376 °C and has a low volatility, as the vapour pressure is < 0.0001 Pa at 25 °C. Moreover the target substance is used in a non-solid and granular form. Therefore, under normal use and handling conditions, the potential for exposure via the inhalation route and thus availability for respiratory absorption of the substance in the form of gases, vapours, liquid aerosols, or powders/dusts is considered to be negligible.
However, if absorption occurs, lipophilic compounds with a log Pow > 4, that are poorly soluble in water (1 mg/L or less), can be taken up by micellar solubilisation. Esterases present in the lung lining fluid may also hydrolyse the substance, hence making the resulting alcohol and fatty acids available for respiratory absorption. Overall, if absorption occurs, the absorption rate is not expected to be higher than following oral exposure.
Distribution and Accumulation
Distribution of a compound within the body depends on the physico-chemical properties of the substance; especially the molecular weight, the lipophilic character and the water solubility. In general, the smaller the molecule, the wider is the distribution. If the molecule is lipophilic, it is likely to distribute into cells and the intracellular concentration may be higher than extracellular concentration, particularly in fatty tissues (ECHA, 2017).
As discussed under oral absorption, the target substance may undergo enzymatic hydrolysis in the GI-tract prior to absorption. The fraction of ester absorbed unchanged will undergo enzymatic hydrolysis by ubiquitous esterases, primarily in the liver (Fukami and Yokoi, 2012). The distribution and accumulation of the hydrolysis products is considered the most relevant.
After being absorbed, fatty acids are (re-)esterified along with other fatty acids into triglycerides and released in chylomicrons into the lymphatic system. This route of absorption and metabolism of a fatty acid was shown in an in vivo study performed by Sieber (1974). Twenty-four hours after intraduodenal administration of a single dose of [1-14C]-radiolabelled octadecanoic acid to rats, 52.5 ± 26% of the radiolabelled carbon was recovered in the lymph. A large majority (68 - 80%) of the recovered radioactive label was incorporated in triglycerides, 13 - 24% in phospholipids and 0.7 - 1% in cholesterol esters. No octadecanoic acid was recovered. Almost all the radioactivity recovered in the lymph was localized in the chylomicron fraction. Chylomicrons are transported in the lymph to the thoracic duct and subsequently to the venous system. On contact with the capillaries, enzymatic hydrolysis of chylomicron triacylglycerol fatty acids by lipoprotein lipase takes place. Most of the resulting fatty acids are taken up by adipose tissue and re-esterified into triglycerides for storage. Triacylglycerol fatty acids are also taken up by muscle and oxidized to derive energy or they are released into the systemic circulation and returned to the liver, where they are metabolised, stored or re-enter the circulation (IOM, 2005; Johnson, 1990; Johnson, 2001; Lehninger, 1993; NTP, 1994; Stryer, 1996; WHO, 2001). There is a continuous turnover of stored fatty acids, as they are constantly metabolised to generate energy and then excreted as CO₂. Accumulation of fatty acids takes place only if their intake exceeds the caloric requirements of the organism.
Absorbed alcohols are mainly oxidised to the corresponding fatty acid and then follow the same metabolism as described above for fatty acids, to form triglycerides. The absorption and metabolism of a fatty alcohol was assessed in an in vivo study performed by Sieber (1974). Twenty-four hours after intraduodenal administration of a single dose of [1-14C]-radiolabelled octadecanol to rats, 56.6 ± 14% of the radiolabelled carbon was recovered in the lymph. More than half (52-73%) of the recovered radioactive label was incorporated in triglycerides, 6-13% in phospholipids, 2-3% in cholesterol esters and 4-10% in un-metabolised octadecanol. Almost all the radioactivity recovered in the lymph was localized in the chylomicron fraction. The results of administration of hexanol resulted in a recovery of 8.5% in the lymph (Sieber, 1974), indicating that alcohols with shorter-length carbon chains are hydrolysed to the corresponding fatty acid and transported directly to the liver via the portal vein as the free acid bound to albumin. The conversion into the corresponding fatty acids by oxidation and distribution in the form of triglycerides, means that the metabolites of fatty alcohols are also used as an energy source or stored in adipose tissue.
Metabolism
The metabolism of the target substance initially occurs via enzymatic hydrolysis of the ester resulting in the methyl-branched isomers of C18-fatty acids and C10-fatty alcohol. The esterases catalysing the reaction are present in most tissues and organs, with particularly high concentrations in the GI-tract and in the liver (Fukami and Yokoi, 2012). Depending on the route of exposure, esterase-catalysed hydrolysis takes place at different places in the body. After oral ingestion, esters of alcohols and fatty acids can undergo enzymatic hydrolysis in the GI-tract. In contrast, substances which are absorbed through the pulmonary alveolar membrane or through the skin may enter the systemic circulation directly before entering the liver where hydrolysis will generally take place.
The C10-fatty alcohol of the target substance, as well as the fatty alcohols of the source substances, will mainly be metabolised to the corresponding carboxylic acid via the aldehyde as a transient intermediate (Lehninger, 1993). The stepwise process starts with the oxidation of the alcohol by alcohol dehydrogenase to the corresponding aldehyde, where the rate of oxidation increases with increased chain-length. Subsequently, the aldehyde is oxidised to carboxylic acid, catalysed by aldehyde dehydrogenase. Both the alcohol and the aldehyde may also be conjugated with e.g. glutathione and excreted directly, bypassing additional metabolism steps (WHO, 1999).
The fatty acids can be further metabolised directly following absorption, following oxidation from an alcohol or following de-esterification of triglycerides. A major metabolic pathway for linear and branched fatty acids is the beta-oxidation for energy generation. In this multi-step process, the fatty acids are at first esterified into acyl-CoA derivatives and subsequently transported into cells and mitochondria by specific transport systems. In the next step, the acyl-CoA derivatives are broken down into acetyl-CoA molecules by sequential removal of 2-carbon units from the aliphatic acyl-CoA molecule. Fatty acids having an odd number are oxidized in the same way as fatty acids having an even number, except that propionyl CoA and acetyl CoA, rather than two molecules of acetyl CoA, are produced in the final round of degradation. The activated three-carbon unit in propionyl CoA enters the citric acid cycle after it has been converted into succinyl CoA (Berg, 2002).
Further oxidation via the citric acid cycle leads to the formation of H₂O and CO₂ (Lehninger, 1993). Alternative pathways for long-chain fatty acids include the omega-oxidation at high dose levels (WHO, 1999). The fatty acid can also be conjugated (by e.g. glucuronides, sulphates) to more polar products that are excreted in the urine.
The potential metabolites following enzymatic metabolism of the substance were predicted using the OECD QSAR toolbox version 4.2 (OECD, 2018). The QSAR tool was used to predict metabolites using skin metabolism simulator, rat liver S9 metabolism simulator and microbial metabolism simulator (Table1). For the target substance SMILES codes of three representative constituents were used for the prediction (please refer to the analogue justification for more details on the SMILES codes).
Table1Predicted metabolites using the OECD QSAR toolbox (OECD, 2018)
Representative Constituents of the target substance |
Predicted hepatic metabolites |
Predicted dermal metabolites |
Predicted microbiological metabolites |
1 |
72 |
2 |
82 |
2 |
72 |
2 |
90 |
3 |
57 |
2 |
102 |
Primarily, the ester bond is broken both specifically in the liver and in general in vivo, and the hydrolysis products may be further metabolised. The predicted liver metabolites of the three constituents are the product of alpha-, beta- or omega-oxidation (= addition of hydroxyl group), in total 72, 72 and 57 were predicted for the three representative constituents 1, 2 and 3, respectively. In the case of omega-oxidation, it is followed by further oxidation to the aldehyde, which is then oxidised to the corresponding carboxylic acid. In general, the hydroxyl groups make the substances more water-soluble and susceptible to metabolism by phase II-enzymes. Two skin metabolites each were predicted for the three representative constituents of the target substance, the C10-fatty alcohol and the C18iso-, C18- and C16-fatty acid, respectively. The metabolites formed in the skin are expected to enter the blood circulation and eventually meet the same fate as the hepatic metabolites. More than 82 metabolites were predicted to result from all kinds of microbiological metabolism of the ester in the GI-tract, including hydrolysis of the ester bond, aldehyde formation and fatty acid chain degradation of the molecule. The results of the OECD Toolbox simulation support the information retrieved in the literature on metabolism.
There is no indication that the target substance is activated to reactive intermediates under the relevant test conditions. The experimental studies performed with the target and source substances on genotoxicity (Ames test, gene mutation in mammalian cells in vitro, and chromosome aberration assay in mammalian cells in vitro) were negative, with and without metabolic activation.
Excretion
The C10-fatty acid derived from the oxidation of the corresponding alcohol as well as the methyl branched isomers of C18-fatty acids resulting from hydrolysis of the ester will be metabolised for energy generation or stored as lipid in adipose tissue or used for further physiological functions, like incorporation into cell membranes (Lehninger, 1993). Therefore, the fatty acid metabolites are not expected to be excreted to a significant degree via the urine or faeces but to be excreted via exhaled air as CO₂ or stored as described above. Experimental data with ethyl oleate (CAS 111-62-6, ethyl ester of oleic acid) support this principle. The absorption, distribution, and excretion of 14C-labelled ethyl oleate was studied in Sprague Dawley rats after a single, oral dose of 1.7 or 3.4 g/kg bw (Bookstaff et al., 2003). At sacrifice (72 hours post-dose), mesenteric fat was the tissue with the highest concentration of radioactivity. The other organs and tissues had very low concentrations of test material-derived radioactivity. The main route of excretion of radioactivity in the groups was via the expired air as CO₂. 12 hours after dosing, 40-70% of the administered dose was excreted in expired air (consistent with beta-oxidation of fatty acids). 7-20% of the radioactivity was eliminated via the faeces, and approximately 2% via the urine.
The alcohol component may also be conjugated with e.g. glutathione to form a more water-soluble molecule and excreted via the urine, bypassing further metabolism steps (WHO, 1999). The fraction of octadecyl stearate that is not absorbed in the GI-tract will be excreted via the faeces.
References
Aungst B. and Shen D.D. (1986). Gastrointestinal absorption of toxic agents. In Rozman K.K. and Hanninen O. Gastrointestinal Toxicology. Elsevier, New York, US.
Berg JM, Tymoczko JL, Stryer L. Biochemistry. 5th edition. New York: W H Freeman; (2002). Section 22.3, Certain Fatty Acids Require Additional Steps for Degradation.
Bookstaff et al. (2003). The safety of the use of ethyl oleate in food is supported by metabolism data in rats and clinical safety data in humans. Regul Toxicol Pharm 37: 133-148.
ECHA (2017). Guidance on information requirements and chemical safety assessment, Chapter R.7c: Endpoint specific guidance. Version 3.0, June 2017.
Fiume MM, Bergfeld WF, Belsito DV, Hill RA, Klaassen CD, Liebler D, Marks JG Jr, Shank RC, Slaga TJ, Snyder PW, Andersen FA. Safety assessment of stearyl heptanoate and related stearyl alkanoates as used in cosmetics. Int J Toxicol. 2012 Sep-Oct;31(5 Suppl):141S-6S.
Fukami, T. and Yokoi, T. (2012). The Emerging Role of Human Esterases. Drug Metab Pharmacokinet 27(5): 466-477
IOM (2005). Dietary Reference Intakes for Energy, Carbohydrate, Fiber, Fat, Fatty Acids, Cholesterol, Protein, and Amino Acids (Macronutrients). Institute of the National Academies. The National Academies Press. http://www.nap.edu/openbook.php?record_id=10490&page=R1
Johnson, R.C. et al. (1990). Medium-chain-triglyceride lipid emulsion: metabolism and tissue distribution. Am J Clin Nutr 52(3):502-8.
Johnson W. Jr; Cosmetic Ingredient Review Expert Panel. (2001). Final report on the safety assessment of trilaurin, triarachidin, tribehenin, tricaprin, tricaprylin, trierucin, triheptanoin, triheptylundecanoin, triisononanoin, triisopalmitin, triisostearin, trilinolein, trimyristin, trioctanoin, triolein, tripalmitin, tripalmitolein, triricinolein, tristearin, triundecanoin, glyceryl triacetyl hydroxystearate, glyceryl triacetyl ricinoleate, and glyceryl stearate diacetate. Int J Toxicol. 2001;20 Suppl 4:61-94.
Lehninger, A.L., Nelson, D.L. and Cox, M.M. (1993). Principles of Biochemistry. Second Edition. Worth Publishers, Inc., New York, USA. ISBN 0-87901-500-4.
Mattson, F.H. and Volpenhein, R.A. (1969). Relative rates of hydrolysis by rat pancreatic lipase of esters of C2 - C18 fatty acids with C1 – C18 primary n-alcohols. J Lipid Res Vol(10): 271-276.
National Toxicology Program (NTP) (1994) Comparative toxicology studies of Corn Oil, Safflower Oil, and Tricaprylin (CAS Nos. 8001-30-7, 8001-23-8, and 538-23-8) in Male F344/N Rats as vehicles for gavage. http://ntp.niehs.nih.gov/ntp/htdocs/LT_rpts/tr426.pdf (2011-12-19). Report No.: C62215. Owner company: U.S. Department of Health and Human Services, Public Health Services, National Institutes of Health.
OECD (2018). (Q)SAR Toolbox v4.2 Developed by Laboratory of Mathematical Chemistry, Bulgaria for the Organisation for Economic Co-operation and Development (OECD). Calculation performed 18 Oct 2018. http://toolbox.oasis-lmc.org/?section=overview
Sieber, S.M., Cohn, V.H., and Wynn, W.T. (1974). The entry of foreign compounds into the thoracic duct lymph of the rat.Xenobiotica 4(5), 265.
Stryer, L. (1996). Biochemie. 4. Auflage. Heidelberg Berlin Oxford: Spektrum Akademischer Verlag.
US EPA (2012). Estimation Programs Interface Suite™ for Microsoft® Windows, v 4.11. United States Environmental Protection Agency, Washington, DC, USA. Downloaded from: http://www.epa.gov/oppt/exposure/pubs/episuite.htm
WHO (1999). Evaluation of certain food additives and contaminants. Forty-ninth report of the joint FAO/WHO Expert Committee on Food Additives. WHO Technical Report Series 884. ISBN 92 4 120884 8.
WHO (2001). Safety Evaluation of Certain Food Additives and Contaminants: Aliphatic Acyclic Diols, Triols, and Related Substances. WHO Food Additives Series No. 48.
Information on Registered Substances comes from registration dossiers which have been assigned a registration number. The assignment of a registration number does however not guarantee that the information in the dossier is correct or that the dossier is compliant with Regulation (EC) No 1907/2006 (the REACH Regulation). This information has not been reviewed or verified by the Agency or any other authority. The content is subject to change without prior notice.
Reproduction or further distribution of this information may be subject to copyright protection. Use of the information without obtaining the permission from the owner(s) of the respective information might violate the rights of the owner.
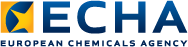