Registration Dossier
Registration Dossier
Data platform availability banner - registered substances factsheets
Please be aware that this old REACH registration data factsheet is no longer maintained; it remains frozen as of 19th May 2023.
The new ECHA CHEM database has been released by ECHA, and it now contains all REACH registration data. There are more details on the transition of ECHA's published data to ECHA CHEM here.
Diss Factsheets
Use of this information is subject to copyright laws and may require the permission of the owner of the information, as described in the ECHA Legal Notice.
EC number: 215-215-7 | CAS number: 1313-99-1
- Life Cycle description
- Uses advised against
- Endpoint summary
- Appearance / physical state / colour
- Melting point / freezing point
- Boiling point
- Density
- Particle size distribution (Granulometry)
- Vapour pressure
- Partition coefficient
- Water solubility
- Solubility in organic solvents / fat solubility
- Surface tension
- Flash point
- Auto flammability
- Flammability
- Explosiveness
- Oxidising properties
- Oxidation reduction potential
- Stability in organic solvents and identity of relevant degradation products
- Storage stability and reactivity towards container material
- Stability: thermal, sunlight, metals
- pH
- Dissociation constant
- Viscosity
- Additional physico-chemical information
- Additional physico-chemical properties of nanomaterials
- Nanomaterial agglomeration / aggregation
- Nanomaterial crystalline phase
- Nanomaterial crystallite and grain size
- Nanomaterial aspect ratio / shape
- Nanomaterial specific surface area
- Nanomaterial Zeta potential
- Nanomaterial surface chemistry
- Nanomaterial dustiness
- Nanomaterial porosity
- Nanomaterial pour density
- Nanomaterial photocatalytic activity
- Nanomaterial radical formation potential
- Nanomaterial catalytic activity
- Endpoint summary
- Stability
- Biodegradation
- Bioaccumulation
- Transport and distribution
- Environmental data
- Additional information on environmental fate and behaviour
- Ecotoxicological Summary
- Aquatic toxicity
- Endpoint summary
- Short-term toxicity to fish
- Long-term toxicity to fish
- Short-term toxicity to aquatic invertebrates
- Long-term toxicity to aquatic invertebrates
- Toxicity to aquatic algae and cyanobacteria
- Toxicity to aquatic plants other than algae
- Toxicity to microorganisms
- Endocrine disrupter testing in aquatic vertebrates – in vivo
- Toxicity to other aquatic organisms
- Sediment toxicity
- Terrestrial toxicity
- Biological effects monitoring
- Biotransformation and kinetics
- Additional ecotoxological information
- Toxicological Summary
- Toxicokinetics, metabolism and distribution
- Acute Toxicity
- Irritation / corrosion
- Sensitisation
- Repeated dose toxicity
- Genetic toxicity
- Carcinogenicity
- Toxicity to reproduction
- Specific investigations
- Exposure related observations in humans
- Toxic effects on livestock and pets
- Additional toxicological data

Carcinogenicity
Administrative data
Description of key information
Value used for CSA (route: oral):
NOAEL: 11 mg Ni/kg bw/day Ni sulphate hexahydrate
Value used for CSA (route: inhalation):
NOAEC: 0.5 mg Ni/m3(Dunnick et al. 1995); and0.1 mg Ni/m3inhalable as the practical threshold for lung cancer (Oller et al., 2014)
Key value for chemical safety assessment
Carcinogenicity: via inhalation route
Endpoint conclusion
- Dose descriptor:
- NOAEC
- 0.62 mg/m³
Justification for classification or non-classification
Ni oxide is classified for carcinogenicity via inhalation (Carc. 1A: H350i) according to the 1st ATP to the CLP Regulation. Supporting information can be found in the discussion section for this endpoint.
Additional information
Data on the oral carcinogenicity of Ni oxide are read-across from Ni sulphate as Ni sulfate represents a worst case scenario for oral absorption and systemic bioavailability of nickel. A 2-year carcinogenicity study with rats performed according to OECD 451 did not show any carcinogenic potential for exposure to nickel sulphate following oral (gavage) administration. The lack of systemic carcinogenicity with the most bioavailable of the Ni compounds can be read across to all other Ni compounds of equal or lesser bioavialability.A summary on this topic is provided in a background document in section 7.5.1 of IUCLID and inAppendix B1of the CSR.
NiO exists as a range of compounds from a high-temperature (green) variety to a low-temperature (jet-black) product. Thermal history is the main factor that determines the color, reactivity and stoichiometric proprtions of nickel and oxygen in the resulting oxide (Van Vlack, 1980). While animal studies have tested the toxicity of individual NiO (high and low calcining temperatures), epidemiological studies always have mixed exposures to a combination of the main chemical forms of nickel (soluble, sulphidic, metallic and oxidic).
The route of exposure of most relevance to humans is inhalation, and thus the most robust and applicable study for characterizing carcinogencity associated with chronic exposure to NiO was that reported by Dunnick and colleagues (1995), in which the findings of a 2-year bioassay conducted by the National Toxicology Program were reported (NTP, 1996a). These authors reported that inhalation of a high-calcining temperature (green) NiO caused lung neoplasms in F344 rats and B6C3F1 mice exposed chronically (two years) for 6 hr/day (3 dose groups tested in both species). In rats, adenomas and carcinomas were observed in the lungs of both male and female rats at concentrations ≥ 1.25 mg/m3(a statistically significant increase in tumors was not observed at 0.62 mg/m3), and pheochromocytomas at the highest dose of 2.5 mg/m3NiO. In B6C3F1 mice, adenomas and carcinomas were observed only in females at 2.5 mg/m3but not at 5.0 mg/m3(a statistically significant increase in tumors was not observed at 1.25 mg/m3), thus demonstrating a lack of dose-response relationship in mice. No other exposure-related neoplasms were found in rats or mice. Based on these collective findings, the authors characterized the evidence for lung tumor formation and adrenal medullary tumors in rats as “some” as opposed to “no” or “clear.”The induction of lung tumors in rats may have been at least in part do to a “particle overload” effect, since impaired lung clearance was noted at the highest exposure levels (Oller et al., 1997). In mice, the authors characterized the evidence for NiO carcinogenicity in the lung as “equivocal.” In contrast to these findings, Haratake et al. (1992) did not find any tumorous lesions in rats exposed to 1 mg NiO/m3(black or green) for six months. Following an additional 12-month observation period in which no additional treatment-related tumors were found, the authors concluded that neither green nor black NiO showed any apparent inducing or promoting effects on tumorigenesis
Although not relevant routes of exposure, studies in rodents on intramuscular and intraperitoneal routes of exposure exist. It was demonstrated that NiO (unspecified color) was carcinogenic following a single i. m. injection of 5 mg in mice and 20 mg in Wistar rats (Gilman, 1962). The majority of these tumors were sarcomas that showed propensity to metastasize to the lungs in rats but less so in mice. Similar results were reported by Sunderman (1984), where i. m. injection of green-grey NiO (14 mg Ni/rat) induced sarcomas in 14/15 F344 rats (52% of these tumors were rhabdomyosarcomas). In a later study, Sunderman et al. (1990) examined the carcinogenicity of three forms of nickel oxide calcined at different temperatures (black, grey-black, and green) following a single i. m. administration of NiO (dose based on concentration of nickel, 20 mg Ni/rat). Only rats exposed to black (low calcining temperature) NiO exhibited sarcomas at the injection site. Similar dose levels were shown to induce sarcomas in rats following a single intrapleural injection of 10 mg NiO (unspecified color/temperature) (Skaug et al., 1985). Pott et al. (1987) reported that i. p. injection of a maximum tolerable dose of 1000 mg NiO (split between two weekly administrations) of unspecified color resulted in tumor formation in 98% of 47 Wistar rats within an average latency period of 31 weeks. This group of researchers also evaluated carcinogenicity in rats following intratracheal administration (10 mg NiO, unspecified color) in Wistar rats once weekly for 5 or 15 consecutive weeks. Approximately 30% of the animals in each dose group (total dose of 50 or 150 mg NiO) exhibited tumors in the lung and trachea.
Taken together, available carcinogenicity data indicate that nickel oxide compounds have the potential to induce tumors at the point of contact following long-term exposure. Physiochemical characteristics greatly influence carcinogenic potential, as demonstrated by findings from injection studies indicating that green nickel oxide has less carcinogenic potency than black nickel oxide. Data were sufficient to characterize inhalation carcinogenicity of green nickel oxide in laboratory animals; however, additional data may be needed to fully characterize the carcinogenic potency of black nickel oxides following long term exposure via environmentally relevant routes. At least with regards to lung toxicity, the black and green forms of nickel oxide behaved similarly in recent acute inhalation studies (EPSL, 2009, 2010), demonstrating similar low acute toxicity.
The epidemiological evaluation of the carcinogenic risk for different chemical forms of nickel(soluble, sulphidic, metallic and oxidic) has some limitations. There are no available cohorts exclusively exposed to a single nickel species. Furthermore, assessments of the relative contribution of the different nickel species far back in time depend largely on 1) more recent exposure measurements that are back extrapolated to earlier times or 2) exposure estimates based on job histories. Both of these approaches introduce uncertainty, and comparatively minor differences in estimated exposures may have a high impact on dose-response relationships. While the animal studies described above have been conducted with various forms of nickel monoxide, the exposures of human cohorts are generally described as being to “oxidic nickel.” Oxidic nickel includes low and high calcining temperature NiO but can also include nickel hydroxides, nickel carbonates, nickel silicates and complex compounds containing other metals (e.g., nickel-copper oxides).
Several epidemiological studies evaluating the association between occupational exposure to oxidic nickel and disease (primarily lung and nasal cancer) were identified. High risk cohorts with significant oxidic nickel exposures are comprised of workers employed in the processing and refining of sulfidic nickel ores in Norway, Wales, and Canada (Doll et al., 1990; Roberts et al., 1989; Andersen et al., 1996; Grimsrud et al., 2003). Low risk cohorts exposed to oxidic nickel are found among lateritic ores refining workers in New Caledonia (Doll et al., 1990; Goldberg et al., 1994) and alloy workers in the USA (Enterline and Marsh, 1982; Redmond et al., 1984; Arena et al., 1998). Exposures in all these workers were always mixed, with confounding from other nickel species as well as other metals, acid mists, arsenic and smoking. This was a factor acknowledged by the authors in each study; however, each of these studies specifically identified nickel oxide as a primary compound to which some workers were exposed.
The Clydach cohort consisted of 2521 men which were employed in various processes in the refining of nickel from grinding and roasting of nickel containing sulfidic nickel and copper, extraction of copper by sulfuric acid, reduction of oxidic nickel by water gas, extraction of nickel as gaseous nickel carbonyl and decomposition of the carbonyl by heat (Doll et al., 1990). Workers were predominantly exposed to sulfidic, oxidic (mainly Ni-Cu oxides and impure NiO) and metallic nickel dusts and, to a lower extent, to soluble nickel salts in a hydrometallurgical plant. Reliable quantitative data about airborne exposures do not exist, except the fact that exposures had been very high in milling/grinding, roasting and plant cleaning occupations. Average levels of 20 mg/m3of oxidic nickel in the calcining area and of 13 mg/m3in thecopper plant were estimated. There were high incidences of lung and nasal cancers in workers hired prior to 1930 (Doll et al., 1990). This sub-cohort exhibited 172 lung cancer cases with a SMR 393 (95% CI 336-456) and 74 nasal cancers with a SMR 21119 (95% CI 16583-26514).Workers hired after 1940 showed little increased lung or nasal cancer risk (Peto et al., 1984; Doll et al., 1990; Sorahan and Williams, 2005). This could be due to the introduction of respiratory protection in some areas of the refinery, reduction in overall dust due to the introduction of improved equipment and changes in the feed material and sulfuric acid. These changes resulted in the elimination of high concentrations of arsenic, copper, and sulfur. In the Grimsrud and Peto (2006) analyses of the Clydach data, increased nasal and lung cancer risk (30%) for workers hired after 1930 are reported. Men that worked 15 years or longer in the Clydach nickel plant had increased SMRs for lung cancer. As oxidic nickel was the predominant species this suggests that it played a role in carcinogenicity of the nickel plant workers. Post 1990 analyses of the data from the Clydach refinery show hints of possible correlations between excess cancer risk and nickel metal and soluble nickel exposure (Easton et al., 1992). However, these associations were either not reproduced or lost statistical significance after accounting for confounding exposures. The authors indicated that their model may have overestimated the risks for metallic (and possibly soluble) nickel and underestimated those for sulfides and/or oxides.
In workers from the refinery in Norway, evaluation was conducted for four groups of nickel species. Oxidic nickel could be found mainly in the roasting, smelting and calcining department. Average levels of oxidic nickel (including complex Ni-Cu oxides and NiO) were estimated at 10 mg/m3before 1956 and 5 mg/m3thereafter, with lesser amounts present in certain areas of the refinery. Workers employed in roasting, smelting and calcining department showed a positive relationship between excess cancer risk and duration of exposure, suggesting an association between carcinogenicity and oxidic nickel exposure (Doll et al., 1990). In an update of this cohort, there continued to be some evidence that long term exposure to oxidic nickel was related to an excess lung cancer risk. Importantly this study showed that cigarette smoking in combination with exposure to nickel appears to produce synergistic effects (Andersen et al.,1996). The authors specifically noted that a dose-response relationship was observed for exposure to nickel oxide and nasal cancer, though the lack of direct evaluation of exposure limits the interpretation of this finding (Andersen et al., 1996). In the Grimsrud et al., (2003) analysis of this cohort, increased relative risks of lung cancer were observed with increased cumulative exposure to oxidic nickel after age adjustments, with or without adjustment for smoking.
Ontario sintering workers at the Copper Cliff plant (Canada) and the leaching, calcining and sintering department within the Port Colborne nickel refinery were exposed to both oxidic and sulfidic nickel. These workers experienced increased SMRs for lung and nasal cancers (Doll et al., 1990; Roberts et al., 1989).
Workers in New Caledonia smelting and refining lateritic ores showed no association between exposures to nickel (mostly in the forms of silicate oxides or complex oxides devoid of copper) and excess nasal or lung cancer risks (Doll et al., 1990; Goldberg et al., 1994). Highest estimated nickel exposure levels were less than 2 mg/m3.
A large study of high nickel alloy workers in the USA included about 31,000 workers followed until 1988 (Enterline and Marsh, 1982; Redmond et al., 1983; 1984; Arena et al., 1998). Workers were exposed mainly to metallic and oxidic nickel mostly present as complex oxides of iron, nickel, chromium, generally free of copper. Average nickel exposures of these workers were relatively low (0.7 mg/m3) (Sivulka and Seilkop, 2009). A small increase in lung cancer risk was found when the cohort was compared to the US population (RR=1.13; 95%CI: 1.05-1.17), but no excess lung cancer risks were found when compared to the local reference populations (RR=1.01; 95%CI: 0.95-1.09).
Exposure to nickel oxide was characterized by Polednak et al (1981) in an evaluation of welders from nuclear facilities in Tennessee. This study did not find compelling associations between exposure and mortality (primarily due to a large amount of variability around standard mortality rates, SMRs), though the authors concluded that high risks for respiratory cancer associated with nickel oxide exposure could not be excluded. It should be noted that this study applies only to exposures during welding. Welding involves exposure to very fine fumes of various metals (mixed oxides and spinels), asbestos, etc., and thus the exposures in this study are not directly comparable to exposures to nickel oxides outside of welding.
Collectively, these studies generally relied on estimates of exposure based on historical industrial hygiene data and/or job exposure matrices. Because it was not possible to determine components of dust or identify the nickel species, it is difficult to state with certainty which specific nickel compounds were associated with the suggested increases in respiratory cancer risk observed in workers with some exposure to nickel oxide. It appears that high risk cohorts had higher levels of exposure to oxidic nickel containing nickel-copper oxides, while low risk cohorts had lower levels of exposure to oxidic nickel devoid of copper. Nickel-copper oxides have been shown in animal injection studies to have a potency similar to that of nickel subsulphide and much higher potency than NiO, to induce tumors (Sunderman et al., 1990). The available epidemiological data characterizing associations between disease and exposure to nickel monoxide do not provide compelling evidence of a causal relationship, though such a relationship can also not be excluded. Stronger evidence is present for exposure to oxidic nickel compounds that include complex Ni-Cu oxides. Taken together the epidemiological and animal data suggest that at least some forms of nickel oxide can be carcinogenic to the respiratory tract of humans after inhalation.
The following information is taken into account for any hazard / risk assessment:
ORAL: Data are read-across from Ni sulphate (highest systemic bioavailability). A well-conducted OECD 451 study in rats did not show any carcinogenic potential of nickel sulphate following oral administration.A summary document that discusses this topic can be found in Appendix B1 of the CSR (and IUCLID Section 7.7).
INHALATION: The most robust and environmentally relevant carcinogenicity study for NiO was conducted as part of a National Toxicology Program study on the toxicity and carcinogenicity of NiSO4, Ni3S2and NiO (Dunnick et al. 1995). Following inhalation of NiO for up to two years (6 hr/d, 5 d/wk, two exposure levels), the authors characterized the evidence for lung tumor formation in rats as “some” as opposed to “no” or “clear. ” In mice, the authors characterized the evidence for NiO carcinogenicity in the lung as “equivocal.” Taken together the epidemiological and animal data suggest that at least some forms of nickel oxide can be carcinogenic to the respiratory tract of humans after inhalation.
DERMAL: Dermal absorption to Ni oxides is very low (<1%). As oral exposure to nickel sulphate resulting in highest systemic bioavailability does not show any carcinogenic potential, there are good reasons to assume that cancer is not a relevant end-point with respect to dermal exposure either.
Information on Registered Substances comes from registration dossiers which have been assigned a registration number. The assignment of a registration number does however not guarantee that the information in the dossier is correct or that the dossier is compliant with Regulation (EC) No 1907/2006 (the REACH Regulation). This information has not been reviewed or verified by the Agency or any other authority. The content is subject to change without prior notice.
Reproduction or further distribution of this information may be subject to copyright protection. Use of the information without obtaining the permission from the owner(s) of the respective information might violate the rights of the owner.
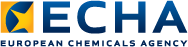