Registration Dossier
Registration Dossier
Data platform availability banner - registered substances factsheets
Please be aware that this old REACH registration data factsheet is no longer maintained; it remains frozen as of 19th May 2023.
The new ECHA CHEM database has been released by ECHA, and it now contains all REACH registration data. There are more details on the transition of ECHA's published data to ECHA CHEM here.
Diss Factsheets
Use of this information is subject to copyright laws and may require the permission of the owner of the information, as described in the ECHA Legal Notice.
EC number: 232-077-3 | CAS number: 7785-26-4
- Life Cycle description
- Uses advised against
- Endpoint summary
- Appearance / physical state / colour
- Melting point / freezing point
- Boiling point
- Density
- Particle size distribution (Granulometry)
- Vapour pressure
- Partition coefficient
- Water solubility
- Solubility in organic solvents / fat solubility
- Surface tension
- Flash point
- Auto flammability
- Flammability
- Explosiveness
- Oxidising properties
- Oxidation reduction potential
- Stability in organic solvents and identity of relevant degradation products
- Storage stability and reactivity towards container material
- Stability: thermal, sunlight, metals
- pH
- Dissociation constant
- Viscosity
- Additional physico-chemical information
- Additional physico-chemical properties of nanomaterials
- Nanomaterial agglomeration / aggregation
- Nanomaterial crystalline phase
- Nanomaterial crystallite and grain size
- Nanomaterial aspect ratio / shape
- Nanomaterial specific surface area
- Nanomaterial Zeta potential
- Nanomaterial surface chemistry
- Nanomaterial dustiness
- Nanomaterial porosity
- Nanomaterial pour density
- Nanomaterial photocatalytic activity
- Nanomaterial radical formation potential
- Nanomaterial catalytic activity
- Endpoint summary
- Stability
- Biodegradation
- Bioaccumulation
- Transport and distribution
- Environmental data
- Additional information on environmental fate and behaviour
- Ecotoxicological Summary
- Aquatic toxicity
- Endpoint summary
- Short-term toxicity to fish
- Long-term toxicity to fish
- Short-term toxicity to aquatic invertebrates
- Long-term toxicity to aquatic invertebrates
- Toxicity to aquatic algae and cyanobacteria
- Toxicity to aquatic plants other than algae
- Toxicity to microorganisms
- Endocrine disrupter testing in aquatic vertebrates – in vivo
- Toxicity to other aquatic organisms
- Sediment toxicity
- Terrestrial toxicity
- Biological effects monitoring
- Biotransformation and kinetics
- Additional ecotoxological information
- Toxicological Summary
- Toxicokinetics, metabolism and distribution
- Acute Toxicity
- Irritation / corrosion
- Sensitisation
- Repeated dose toxicity
- Genetic toxicity
- Carcinogenicity
- Toxicity to reproduction
- Specific investigations
- Exposure related observations in humans
- Toxic effects on livestock and pets
- Additional toxicological data

Basic toxicokinetics
Administrative data
- Endpoint:
- basic toxicokinetics in vivo
- Type of information:
- experimental study
- Adequacy of study:
- key study
- Study period:
- no data
- Reliability:
- 2 (reliable with restrictions)
- Rationale for reliability incl. deficiencies:
- study well documented, meets generally accepted scientific principles, acceptable for assessment
Cross-referenceopen allclose all
- Reason / purpose for cross-reference:
- reference to same study
- Reason / purpose for cross-reference:
- reference to other study
Data source
Reference
- Reference Type:
- publication
- Title:
- Human metabolism od alpha-pinene and metabolite kinetics after oral administration
- Author:
- Schmidt L, Göen T
- Year:
- 2 015
- Bibliographic source:
- Schmidt, L. & Göen, T. Arch Toxicol (2015). doi:10.1007/s00204-015-1656-9
Materials and methods
- Objective of study:
- metabolism
- other: elimination kinetics
- Principles of method if other than guideline:
- Metabolism and elimination kinetics of alpha-pinene in 4 volunteers
- GLP compliance:
- no
Test material
- Reference substance name:
- (-)-pin-2(3)-ene
- EC Number:
- 232-077-3
- EC Name:
- (-)-pin-2(3)-ene
- Cas Number:
- 7785-26-4
- Molecular formula:
- C10H16
- IUPAC Name:
- (1S,5S)-2,6,6-trimethylbicyclo[3.1.1]hept-2-ene
- Test material form:
- liquid
Constituent 1
- Specific details on test material used for the study:
- (1S,5S)-(−)-α-pinene (enantiomeric reference for αPN; ≥99.0 %),
Test animals
- Species:
- other: human
- Sex:
- male/female
- Details on test animals or test system and environmental conditions:
- Four healthy human volunteers (3 men and 1 woman), mean age 33 ± 11 years, mean body weight (80 ± 8 kg)
Administration / exposure
- Route of administration:
- oral: capsule
- Details on exposure:
- Volunteers were orally exposed to 9.0 ± 0.4 mg (66 ± 3 μmol,M = 136.23 mg mmol−1) αPN via spiked gelatin capsules.
The volunteers ingested the capsules in the morning on an empty stomach After the exposure, they fasted for 1 h. During the remaining time of the experiment, they were allowed to eat and drink normally.
Doses / concentrations
- Dose / conc.:
- 9 other: mg
- Remarks:
- 9.0 ± 0.4 mg (66 ± 3 μmol,M = 136.23 mg mmol−1)
- No. of animals per sex per dose / concentration:
- 4 healthy human volunteers (3 men and 1 woman)
- Control animals:
- no
- Positive control reference chemical:
- no
- Details on study design:
- The volunteers ingested the capsules in the morning on an empty stomach
- Details on dosing and sampling:
- The volunteers ingested the capsules in the morning directly after the collection of one preexposure urine sample (n = 4 volunteers) and one blood sample (n = 2 volunteers).
- Tissues and body fluids sampled: Urine and blood
- Time and frequency of sampling: Urine samples were collected by every volunteer throughout 24 h as often as possible. For every urine sample, the sampling time and the excretion volume were recorded. Blood samples were drawn from two volunteers every hour until 5 h after exposure.
- Storage: Urine samples were stored frozen under nitrogen protective gas at −20 °C until analysis. Blood samples: after aliquotation of 2 mL for headspace analysis, the remaining volumes were stored frozen at −20 °C until analysis.
- Method type(s) for identification: Gas chromatographic–mass spectrometric analyses (HS–GC–MS and GC-PCI-MS/MS); alpha pinene in blood was analysed by HS-GC-MS. alpha pinene metabolites in blood and urine was analysed by GS-PCI-MS/MS.
- Urine samples were analysed according to the procedure of Schmidt et al. (2015). - Statistics:
- none
Results and discussion
Toxicokinetic / pharmacokinetic studies
- Details on absorption:
- Maximum blood concentrations (cmax) were reached in the samples drawn 1 and 3 h after exposure (tmax), respectively.
- Details on distribution in tissues:
- Noticeable amounts of αPN or related volatile αPN metabolites obviously seem to reach the lungs since all volunteers reported a characteristic flavour of the exhaled air.
- Details on excretion:
- Renal excretion of cVER in the pre-exposure samples was 22 ± 11 μg L−1. After oral administration renal elimination rates of cVER increased distinctly within the first 1–3 h after exposure to its maximum of 1600 ± 650 μg L−1 and declined afterwards to the base levels within the 24-h observation period. At its maximum urinary concentrations, cVER was present in conjugated forms by 28–76 %. Renal excretion kinetics of cVER showed an elimination half-life of about 1.5 ± 0.2 h. The cumulative excretion within 24 h after exposure correlated with about 5.6 ± 0.5 % of the applied dose.
Renal excretion of tVER in the pre-exposure samples was 14 ± 7.5 μg L−1 . After oral administration renal elimination rates of tVER increased distinctly within the first 1–3 h after exposure to its maximum of 1900 ± 1700 μg L−1and declined afterwards to the base levels within the 24-h observation period . At its maximum urinary concentrations, tVER was present in conjugated forms by 4–68 %. Renal excretion kinetics of tVER showed an elimination half-life of about 1.6 ± 0.2 h. The cumulative excretion within 24 h after exposure correlated with about 4.1 ± 0.4 % of the applied dose
Renal excretion of MYR in the pre-exposure samples was 7.3 ± 1.4 μg L−1. After oral administration renal elimination rates of MYR, increased distinctly within the first 1–3 h after exposure to its maximum of 690 ± 530 μg L−1 and declined afterwards to the base levels within the 24-h observation period . At its maximum urinary concentrations, MYR was present in conjugated forms by 99–100 %. Renal excretion kinetics of MYR showed an elimination half-life of about 1.6 ± 0.2 h. The cumulative excretion within 24 h after exposure correlated with about 1.5 ± 0.2 % of the applied dose
Renal excretion of MYRA in the pre-exposure samples was 16 ± 6.3 μg L−1. After oral administration renal elimination rates of MYRA increased distinctly within the first 1–3 h after exposure to its maximum of 3200 ± 2700 μg L−1and declined afterwards to the base levels within the 24-h observation period. At its maximum urinary concentrations, MYRA was present in conjugated forms by 99–100 %. Renal excretion kinetics of MYRA showed an elimination half-life of about 1.4 ± 0.1 h. The cumulative excretion within 24 h after exposure correlated with about 6.7 ± 0.3 % of the applied dose.
Elimination of the 3 ununknown αPN metabolites (αPN-M1, αPN-M2, and αPN-M3) . accounted for about 1 % (αPN-M1), 10 % (αPN-M2), and 2 % (αPN-M3) of the orally applied dose.
The cumulative excretion graphs suggest completeness ofelimination about 10 h after exposure.
Toxicokinetic parametersopen allclose all
- Toxicokinetic parameters:
- half-life 1st: 1.5 ± 0.2 h
- Remarks:
- mean renal elimination half-live of cVER
- Toxicokinetic parameters:
- half-life 1st: 1.6 ± 0.2 h
- Remarks:
- mean renal elimination half-live of tVER
- Toxicokinetic parameters:
- half-life 1st: 1.6 ± 0.2 h
- Remarks:
- mean renal elimination half-live of MYR
- Toxicokinetic parameters:
- half-life 1st: 1.4 ± 0.1 h
- Remarks:
- mean renal elimination half-live of MYRA
Metabolite characterisation studies
- Metabolites identified:
- yes
- Details on metabolites:
- In blood: detection of cVER (cis- verbenol) , tVER (trans-verbenol) , and MYR (myrtenol) over the entire blood sampling period of 1–5 h.
In urine: detction of: cVER, tVER, MYRA (myrtenic acid), and MYR and three peaks of unknown αPN metabolites (αPN-M1, αPN-M2, and αPN-M3) . cVER account for 5.6 ± 0.5 % of the applied oral αPN dose, tVER for 4.1 ± 0.4 %, MYR for 1.5 ± 0.2 % and MYRA for 6.7 ± 0.3 %. It was estimated that the newly identified metabolites accounted for about 1 % (αPN-M1), 10 % (αPN-M2), and 2 % (αPN-M3) of the orally applied dose. The quantified metabolites in urine only accounted for about 22 % of the orally applied dose
It was supposed that αPN-M1 is MYRA-4-OH and αPN-M2 is identical with MYRA. αPN-M3 may be an isomer of saturated MYRA, i.e. DHMYRA.
Any other information on results incl. tables
None of the volunteers reported any adverse health effects due to the supplementation with 10 mg of αPN. Though, all volunteers mentioned a characteristic aromatic smell of the exhaled breath which occurred about 1 h after oral exposure and subsequently vanished within 2–3 h after exposure. It was assume that respiratory elimination of unmetabolized αPN was a substantial elimination pathway, as well, which may have contributed to a large extend to the 78 % of the oral dose which was not recovered in form of renal metabolites. This is in accordance with the volunteers’ reports on olfactory perception during the exposure experiments. However, the amount of αPN exhaled was not quantified.
The synchronous and steep metabolite time courses observed in blood and urine may be explained by simultaneous and competing reactions during first-pass metabolism. This first-pass effect, which rapidly yields in polar phase I and II metabolites, is presumably responsible for the low blood concentrations as well as the short elimination half-lives in the first elimination phases. The second, slower elimination phases observed for all metabolites are most probably caused by release of low amounts of αPN or its metabolites from tissue compartments. However, the cumulative elimination indicate that the second phase only plays a minor role to the cumulatively eliminated amount. At the elimination peak, MYR and MYRA are almost entirely conjugated to glucuronic acid (or sulphate), whereas cVER and tVER are only conjugated up to 76 %. This effect may be due to steric hindrance of the secondary hydroxyl groups caused by the bicyclic backbone or due to instability of the conjugates.
Table1: Characteristics of the blood kinetics ofαPN metabolites after oral exposure to 9.0±0.4 mg (66±2.8 µmol)αPN (mean val- ues±range;n=2 volunteers)
Metabolite |
cmax(µgL−1) |
cmax(nM) |
tmax(h) |
t1/2(h) |
cVER |
1.4±0.7 |
9.3±4.8 |
1–3 |
0.8a |
tVER |
4.0±2.1 |
26±14 |
1–3 |
1.0a |
MYR |
1.7±0.4 |
11±2.4 |
1–3 |
1.7a |
a Elimination not completed within 5 h observation period
Table 2: Characteristics of the renal αPN metabolite elimination kinetics after oral exposure to 9.0±0.4 mg (66±2.8 µmol)αPN (mean values±SD;n=4 volunteers)
Metabolites |
RE,max(µg h−1) |
tmax(h) |
t1/2(h) |
kel(h−1) |
AUC0 tf(µmol) |
Share of oral dose(%) |
cVER
|
170±97 |
1.6±0.9 |
1.5±0.2 |
0.461 |
3.7±0.3 |
5.6± 0.5 |
T VER |
120±64 |
1.6±0.9 |
1.6±0.2 |
0.436 |
2.7±0.3 |
4.1±0.4 |
MYR |
48±30 |
1.6±0.9 |
1.6±0.2 |
0.440 |
1.0±0.1 |
1.5±0.2 |
MYRA |
230±130 |
1.6±0.9 |
1.4±0.1 |
0.483 |
4.4±0.1 |
6.7±0.3 |
RE,maxmaximum renal excretion;tmaxtime to reach maximum renal excretion;t1/2elimination half-life;AUC0→tfarea under the renal excretion vs. time curve (from time 0 to final sampling timetf);Vtotalsummarized excreted urine volume
Applicant's summary and conclusion
- Conclusions:
- The present study demonstrates in details the human αPN metabolism after oral uptake and the elimination kinetics of four relevant metabolites (cVER, tVER, MYRA, and MYR ). Two unknown human metabolites, whose predicted structures [4-hydroxymyrtenic acid (MYRA-4-OH) and dihydromyrtenic acid (DHMYRA)] were identified. It is demonstrated that human metabolism of αPN proceeds fast and the body is almost entirely cleared from the metabolites 10 h after exposure.
- Executive summary:
In a metabolism study, four healthy human volunteers were orally exposed to a single dose of 9 mg of (1S,5S)-(−)-α- pinene (αPN) via spiked gelatin capsules. Each volunteer gave one urine sample before administration and subsequently collected each urine sample within 24 h after administration. Blood samples were collected directly after administration of the capsule and every hour until 5 hours exposure for 2 volunteers. The concentration of the αPN metabolites were determined using a very specific and sensitive GC-PCI-MS/MS procedure. Concentration of αPN was analysed in blood by HS-GC-MS procedure.
αPN metabolites cVER (cis- verbenol) , tVER (trans-verbenol) , and MYR (myrtenol) were detected in blood samples over the entire blood sampling period of 1–5 h, unmetabolised αPN were below the limit of detection.
The metabolite concentrations showed synchronous time courses even though the levels were low and varied between the two volunteers. Metabolite blood levels were low. The non-detection of αPN in blood after low oral doses in contrast to the detectable metabolite levels indicates a fast and approximately entire pre-systemic metabolism such as hepatic or intestinal firstpass metabolism.
αPN metabolites were detected in urine in considerably higher amounts in contrast to blood levels. The low blood concentrations compared to the high urinary levels, thus, indicate a fast transfer from blood to urine and a rapid renal elimination.
In addition to the known and established αPN metabolites cVER and tVER, the relevance of MYR and MYRA as products of the human in vivo metabolism of αPN was confirmed. Two unknown human metabolites were identified and these structures could be predicted as 4-hydroxymyrtenic acid (MYRA-4-OH) and dihydromyrtenic acid (DHMYRA).
Human in vivo metabolism of αPN is similarly dominated by extensive oxidation reactions on the methyl side-chains yielding in carboxylic acid structures. Nonetheless, only 22% of the applied dose was quantified as metabolite. Thus, further metabolites and the share of αPN eliminated unchanged via lungs remain unclear.
Human metabolism of αPN proceeds fast and the body is almost entirely cleared from the metabolites 10 h after exposure
Information on Registered Substances comes from registration dossiers which have been assigned a registration number. The assignment of a registration number does however not guarantee that the information in the dossier is correct or that the dossier is compliant with Regulation (EC) No 1907/2006 (the REACH Regulation). This information has not been reviewed or verified by the Agency or any other authority. The content is subject to change without prior notice.
Reproduction or further distribution of this information may be subject to copyright protection. Use of the information without obtaining the permission from the owner(s) of the respective information might violate the rights of the owner.
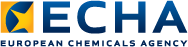