Registration Dossier
Registration Dossier
Data platform availability banner - registered substances factsheets
Please be aware that this old REACH registration data factsheet is no longer maintained; it remains frozen as of 19th May 2023.
The new ECHA CHEM database has been released by ECHA, and it now contains all REACH registration data. There are more details on the transition of ECHA's published data to ECHA CHEM here.
Diss Factsheets
Use of this information is subject to copyright laws and may require the permission of the owner of the information, as described in the ECHA Legal Notice.
EC number: 944-341-1 | CAS number: -
- Life Cycle description
- Uses advised against
- Endpoint summary
- Appearance / physical state / colour
- Melting point / freezing point
- Boiling point
- Density
- Particle size distribution (Granulometry)
- Vapour pressure
- Partition coefficient
- Water solubility
- Solubility in organic solvents / fat solubility
- Surface tension
- Flash point
- Auto flammability
- Flammability
- Explosiveness
- Oxidising properties
- Oxidation reduction potential
- Stability in organic solvents and identity of relevant degradation products
- Storage stability and reactivity towards container material
- Stability: thermal, sunlight, metals
- pH
- Dissociation constant
- Viscosity
- Additional physico-chemical information
- Additional physico-chemical properties of nanomaterials
- Nanomaterial agglomeration / aggregation
- Nanomaterial crystalline phase
- Nanomaterial crystallite and grain size
- Nanomaterial aspect ratio / shape
- Nanomaterial specific surface area
- Nanomaterial Zeta potential
- Nanomaterial surface chemistry
- Nanomaterial dustiness
- Nanomaterial porosity
- Nanomaterial pour density
- Nanomaterial photocatalytic activity
- Nanomaterial radical formation potential
- Nanomaterial catalytic activity
- Endpoint summary
- Stability
- Biodegradation
- Bioaccumulation
- Transport and distribution
- Environmental data
- Additional information on environmental fate and behaviour
- Ecotoxicological Summary
- Aquatic toxicity
- Endpoint summary
- Short-term toxicity to fish
- Long-term toxicity to fish
- Short-term toxicity to aquatic invertebrates
- Long-term toxicity to aquatic invertebrates
- Toxicity to aquatic algae and cyanobacteria
- Toxicity to aquatic plants other than algae
- Toxicity to microorganisms
- Endocrine disrupter testing in aquatic vertebrates – in vivo
- Toxicity to other aquatic organisms
- Sediment toxicity
- Terrestrial toxicity
- Biological effects monitoring
- Biotransformation and kinetics
- Additional ecotoxological information
- Toxicological Summary
- Toxicokinetics, metabolism and distribution
- Acute Toxicity
- Irritation / corrosion
- Sensitisation
- Repeated dose toxicity
- Genetic toxicity
- Carcinogenicity
- Toxicity to reproduction
- Specific investigations
- Exposure related observations in humans
- Toxic effects on livestock and pets
- Additional toxicological data

Endpoint summary
Administrative data
Link to relevant study record(s)
- Endpoint:
- basic toxicokinetics
- Type of information:
- read-across from supporting substance (structural analogue or surrogate)
- Adequacy of study:
- key study
- Study period:
- 2006-10-16 to 2006-12-07
- Reliability:
- 2 (reliable with restrictions)
- Rationale for reliability incl. deficiencies:
- other: GLP guideline study
- Objective of study:
- absorption
- distribution
- excretion
- metabolism
- Qualifier:
- according to guideline
- Guideline:
- OECD Guideline 417 (Toxicokinetics)
- Deviations:
- no
- Qualifier:
- according to guideline
- Guideline:
- EPA OPPTS 870.7485 (Metabolism and Pharmacokinetics)
- Deviations:
- no
- Qualifier:
- according to guideline
- Guideline:
- other: EU Council Directive 91/414/EEC amended by the Commission Directive 94/79/EC
- Deviations:
- no
- GLP compliance:
- yes (incl. QA statement)
- Remarks:
- Ministerium für Umwelt, Raumordnung und Landwirtschaft des Landes Nordrhein-Westfalen (Aktenzeichen: VI-3-31.11.62.04)
- Radiolabelling:
- yes
- Remarks:
- [14C]label positions: 2,3-14C1, 2'3'-14C1; [13C]label positions: 2,3-13C1, 2'3'-13C1
- Species:
- rat
- Strain:
- Wistar
- Sex:
- male
- Details on test animals or test system and environmental conditions:
- TEST ANIMALS
- Strain: Wistar Hsd/Cpb: WU
- Source: Harlan & Winkelmann GmbH, Gartenstraße 27, 33178 Borchen, Germany
- Age at study initiation: 7 weeks at the time of delivery
- Weight at study initiation: a mean weight of about 200 g: 194 - 205 g at the time of administration; 206 - 215 g at the time of sacrifice
- Fasting period before study: yes, last feeding ca. 16 hours prior to dosing, next feeding ca. 6 hours after dosing
- Housing: Conventional hygienic conditions in air-conditioned rooms
- Individual metabolism cages: yes After administration of the radiolabelled test item, the rats were kept individually in Makrolon® metabolism cages. With these cages, an almost quantitative and separate collection of urine and faeces was possible.
- Diet (e.g. ad libitum): ad libitum (Rat/mice maintenance long life diet (no. 3883.0.15), supplied by Provimi Kliba AG, CH-4303 Kaiseraugst, Switzerland (ca. 16 g per animal and day),
- Water (e.g. ad libitum): Tap water from the local mains supply (ad libitum)
- Acclimation period: 8 days
ENVIRONMENTAL CONDITIONS
- Temperature (°C): 21 - 24
- Humidity (%): 53 - 60
- Air changes (per hr): 10-15
- Photoperiod (hrs dark / hrs light): 12/12
IN-LIFE DATES: From: To: - Route of administration:
- oral: gavage
- Vehicle:
- water
- Details on exposure:
- PREPARATION OF DOSING SOLUTIONS:
The [14C]labelled test item (BECH 2014; specific radioactivity: 4.31 MBq/mg) was shipped as aqueous solution (54.86 mg IDS, Na4 salt in 15 g water). Before preparation of the administration solution, the identity was confirmed by LC-MS/MS. The radiochemical purity (sum of isomer 1 and 2) was checked by radio-HPLC. The [13C]labelled test item was shipped as solid material (1027 mg) and was dissolved in 10 g water. The unlabelled test item was shipped as aqueous solution (34.7% = 347 mg IDS, Na4 salt/g solution).
The preparation of the administration solution used for the ADME-experiment (test 1) is shown in the table 1.
Based on the calculation presented in the table 1 a final specific radioactivity of 0.039 MBq/mg (1.06 u.Ci/mg) was obtained. The administration solution was prepared one day before dosing. The radiochemical purity and concentration was determined by radio-HPLC and by LSC, respectively. The calibration results of the administration solution served as a reference for the calculation of the total radioactivity in the biological samples.
For test 2, 3.47 g of the unlabelled test items (34.7%) solution was filled up with water to a total weight of 12 g.
HOMOGENEITY AND STABILITY OF TEST MATERIAL: - Duration and frequency of treatment / exposure:
- single administration
- Remarks:
- Doses / Concentrations:
Test 1: 1000 mg/kg bw (nominal);
Test 2: 1000 mg/kg bw (nominal). - No. of animals per sex per dose / concentration:
- 5 animals dosed with the radiolabelled test item (test 1)
5 animals dosed with the non-radiolabelled test item (test 2) - Control animals:
- yes, concurrent no treatment
- Positive control reference chemical:
- None.
- Details on study design:
- - Dose selection rationale: Five male rats each were assigned to their respective test groups 1 and 2 as noted in Table 2. They received the calculated volume by oral gavage using a syringe attached to an animal-feeding knob cannula. The volume was based on the nominal average animal weight of 200 g. The concentration of each administration solution was calculated to reach an administered amount of about the nominal value of the parent compound per kg body weight (bw). Due to different animal weights at administration, the actual dose per kg varied slightly with the body weight. Two millilitre of the respective administration solution were dosed to each animal. The amounts of the test compound administered in the different experiments are shown in the Table 3.
- Details on dosing and sampling:
- PHARMACOKINETIC STUDY (Absorption, distribution, excretion)
- Tissues and body fluids sampled: urine, faeces, blood, plasma, cage washes, liver, kidney, GIT, skin and carcass
- Time and frequency of sampling: see Table 2
METABOLITE CHARACTERISATION STUDIES
- Tissues and body fluids sampled: urine and faeces
- Time and frequency of sampling: Urine (0 - 4 h, 4 - 8 h, 8 - 24 h, 24 - 48 h and 48 - 72 h); faeces (0-24 h); see also Table 2
- From how many animals: all animals (pooled)
- Method type(s) for identification (HPLC-UV, Liquid scintillation counting, NMR)
- Limits of detection and quantification: For all samples, the limit of detection (LOD) was predefined on the base of the background radioactivity counting rate which was in the range between 12 - 29 cpm (in average 21 cpm), depending on the instrument used. The LOD was established as twice the average background radioactivity. Single background subtraction and a quench and counting efficiency correction for transformation of gross counts (cpm) into net counts (dpm) were automatically performed by the instruments. Therefore, samples with individually measured values below 21 dpm (after correction for the background radioactivity) were not quantified and labelled as < LOD or LOQ in the respective tables. The limit of quantification (LOQ) was set on the level of LOD.
A typical calculation example for the LOD of combustion/LSC is given below:
Limit of detection = (BKG x Df)/representative sample weight x (1/SA) = (21dpm x 0.2/ 0.05 g) x (1 µg/2363 dpm) = 0.036 mg/kg
where:
BKG : average background radioactivity (21 dpm);
Df : freeze drying factor (e.g.: 0.2), (= weight of sample after lyophilisation / weight of sample prior to lyophilisation); this factor is equal to 1 in case of liquid samples;
0.05 : typical amount of sample used for radioactive measurement [g];
SA: specific radioactivity of the test item [dpm/ug] = 2363 dpm/µg.
The counting times of the samples were generally between seconds and 20 minutes depending on the amount of radioactivity present in the sample. The measurement was stopped after reaching a 2-a error of 0.7%. If this error was not reached within 20 minutes, the measurement was stopped and the 2-a error of the dpm-value (LKB/Wallac 1219 Spectral) or cpm-value (Beckman counter) reached at this time was recorded.
OTHER: Determination of Osmolality, pH and Metals in Urine and Plasma
The osmolality, pH-values and amount of selected metals in urine and plasma samples from rats which received nearly the same amount of the unlabelled IDS, Na4 salt (test 2) were additionally determined and compared to control samples obtained from untreated rats. - Statistics:
- see attached background material.
- Type:
- absorption
- Results:
- at least 37 % of the administered dose becomes systemically available.
- Type:
- distribution
- Results:
- 2.2 % were found in the total body. The relatively highest equivalent concentrations were detected in the liver (50 mg/kg), followed by kidney (36 mg/kg) and skin (25 mg/kg). For erythrocytes, plasma, carcass and GIT, the values were below 20 mg/kg.
- Type:
- metabolism
- Results:
- The unchanged substance was recovered in the urine (99.5 % during 0-72 h) and in the faeces (93.3 % during 0-24 h). A biotransformation pathway was not proposed because no single unknown metabolite was higher than 0.2 % in urine and 2 % in faeces.
- Type:
- excretion
- Results:
- The excretion of radioactivity via urine and faeces was fast and almost completed at 24 h after administration. The by far major part of the dose (68.7 %) was excreted with faeces and 34.7 % with urine.
- Details on absorption:
- The absorption of the test item related radioactivity from the gastrointestinal tract and distribution into the different organs and tissues was investigated by determination of the test item equivalent concentrations (= TRR) in plasma at fixed dates over the whole testing period. The individual values for the equivalent and dose-normalised concentrations are given in Table 4. Oral administration of [14C]IDS, Na4 salt to male rats resulted in a rapid appearance of substantial plasma concentrations of 14C at the first time point of 10 min postdose and quantifiable concentrations at all time points thereafter. The time course of the plasma concentrations showed a biphasic behaviour (Figure 1). A first portion of the dose of was rapidly absorbed from the gastrointestinal tract immediately after dosing without a recognisable lag-time and reached a maximum concentration (Cmax) of 186 mg/kg after 20 min. This value corresponded to 19.4% of the equidistribution concentration of the administered dose. Until 4 hours after dosing, the concentration dropped to about 10 mg/kg which indicated a fast elimination of the test item related radioactivity from the central compartment (blood) towards the peripheral compartments (organs and tissues; 1st elimination phase). A second increase of the TRR-values to approx. 65 mg/kg until 24 hours was measured thereafter followed by a decrease to a final value of 17 mg/kg of 72 hours post dosing (2nd elimination phase). An apparent saturation of absorption due to the high administered amount during the first phase might have been the reason for this biphasic behaviour. An exact value for the absorption rate could not be taken from these figures. But taking the urinary excretion behaviour and the remaining radioactivity in the body at sacrifice into account it can be concluded that at least 37% of the administered dose becomes systemically available.
A summary of the key pharmacokinetic parameters of total [14C] in plasma is presented in Table 5.The half-life for the first elimination phase (t1/2e1) which lasted from 40 min to 4 h postdose amounted to 0.7 h. For the second elimination phase from 24 to 72 h postdose, the terminal half-life (t1/2 e2) and the terminal elimination rate accounted for 25 h and 0.028 [h-1], respectively. The mean area under the curve from 0.16 - 4 h (AUC 0.16 -4) was 296 mg x h/kg (9%), from 4 - 72 h (AUC 4 - 72) 2393 mg x h/kg (72%), and the mean AUC extrapolated from 72 h to infinity (AUC 72 - ∞)was 602 mg x h/kg (18%). As shown for the second AUC-value from 4 - 72 h postdose, a similar high value was calculated for the respective mean area under the data value (AUD 4 -72) of 2393 mg x h/kg. The second absorption and elimination phases contributed obviously mainly to the systemic exposure of the test item and/or its metabolites. The mean residence time (MRT 0 - ∞) of 45 h was relatively high but in view of a low accumulation factor of 1.4 a retention of test item related radioactivity in the body could be excluded. - Details on distribution in tissues:
- The total radioactive residues in the organs and tissues (expressed as equivalent and dose normalised concentrations as well as percentage of administered dose) were determined at sacrifice (Table 8). In relation to the administered dose, only 2.2 % were found in the total body. The carcass, skin and liver contributed in decreasing order mostly to this value. The relatively highest equivalent concentrations were detected in the liver (50 mg/kg), followed by kidney (36 mg/kg) and skin (25 mg/kg). For all other samples (erythrocytes, plasma, carcass and GIT), the values were below 20
mg/kg. - Details on excretion:
- The excretion of radioactivity via urine and faeces is reported in Table 7. In contrast to the high administered amount, the excretion was fast and almost completed at 24 h after administration. The by far major part of the dose (68.7%) was excreted with faeces and 34.7% with urine.
- Test no.:
- #1
- Toxicokinetic parameters:
- Cmax: 186 mg/kg
- Test no.:
- #1
- Toxicokinetic parameters:
- Tmax: 0.33 h
- Test no.:
- #1
- Toxicokinetic parameters:
- half-life 1st: 0.73 h
- Test no.:
- #1
- Toxicokinetic parameters:
- half-life 2nd: 25.14 h
- Test no.:
- #1
- Toxicokinetic parameters:
- AUC: 296.38 mg x h/kg (for 0.16-4 h)
- Test no.:
- #1
- Toxicokinetic parameters:
- AUC: 23.92.71 mg x h/kg (for 4-72 h)
- Test no.:
- #1
- Toxicokinetic parameters:
- AUC: 602.4 mg x h/kg (for 72- ∞ h)
- Metabolites identified:
- no
- Details on metabolites:
- Urine pool samples from the different time period were directly taken for elucidation of the parent compound and metabolites. The 0 - 24 h faeces pool sample was conventionally extracted with ACN/water mixtures. The extraction efficiency was 97 % proving the adequacy of this procedure. The combined extracts were directly used for analysis. Faeces samples from the collection periods 24 - 48 h and 48 - 72 h were not analysed because only 3 % of the faecal radioactivity was found in these samples (Table 7 of IUCLID dataset). The parent compound only was unambiguously identified in the urine and faeces samples.
The identification rate for the unchanged IDS, Na4 salt was high and amounted 99.5 % for urine (0 - 72 h; Table 9 of IUCLID dataset) and 93.3 % for faeces (0 - 24 h; Table 10 of IUCLID dataset). Relating to the administered dose, IDS, Na4 salt amounted to 34.5 % in urine and 61.2 % in faeces (Table 11 of IUCLID dataset). No single unknown metabolite was higher than 0.2 % in urine and 2 % in faeces.
Because the unchanged IDS, Na4 salt was the only identified compound and significant degradation did not occur a biotransformation pathway was not proposed. - Conclusions:
- Interpretation of results (migrated information): no bioaccumulation potential based on study results
The kinetic and metabolic behaviour of [14C]Iminodisuccinate, Na4 salt in male rats can be characterised by the following observations:
• A first portion of the administered radioactivity was rapidly absorbed, widely distributed into organs and tissues and rapidly eliminated from the body via urine and faeces.
• For a second portion, the absorption and elimination periods were significant longer than for the first one which indicated a higher systemic exposure to the test item related radioactivity during this phase.
• The distribution of the radioactivity within the central compartments of the body (i.e. blood, liver, and kidney) showed a distinctive preference towards the liver and - to a lower extent - to the kidney which had the highest TRR (total radioactive residue) values at sacrifice.
• IDS, Na4 salt was metabolically stable as no metabolites were detected in the excreta in significant amounts.
• From the renal and faecal excretion rate as well as the low accumulation factor it was concluded that the residual radioactivity in the organs and tissues of the rat are subject to further elimination.
• The administration of IDS, Na4 led to transient changes of the urinary osmolality-and pH-values within the test period of 3 days. Based on a high variability of urine pH- and osmolality-values in animal species used in toxicological studies, these effects however were regarded as insignificant. Differences were obtained in the amount of metals in urine and plasma samples between treated and untreated rats.
Based on these results, it is believed that the depletion of residues and metabolic behaviour of [14C]Iminodisuccinate, Na4 salt in male rats is sufficiently understood. - Executive summary:
The present study describes investigations on the pharmacokinetic behaviour (absorption, distribution, excretion) and metabolism of the complexing agent IDHA for metal ions. The test material was labelled with 14C and 13C in the 2 and 3 as well as 2' and 3' - positions of imminodisuccinic acid. The radiolabelled and unlabelled test material was administered to two groups of 5 male Wistar rats, respectively, at dose level of 1000 mg/kg bw. The rats received the test item by oral gavage as an aqueous solution. They were sacrificed 72 h after dosing. The total radioactivity that included the test item and possible metabolites was determined in plasma samples, the excreta (urine and faeces) as well as in organs and tissues. The metabolism was investigated by radio-HPLC and spectroscopic methods in selected urine samples and faeces extract. Further, osmolality in urine as well as pH-values and metals in urine and plasma samples collected from rats which received the unlabelled test item were investigated.
Absorption and pharmacokinetic behaviour
The absorption of the test item related radioactivity from the gastrointestinal tract and distribution into the different organs and tissues was investigated by determination of the test item equivalent concentrations (= TRR) in plasma at fixed dates over the whole testing period.
Oral administration of [14C]IDS, Na4 salt to male rats resulted in a rapid appearance of substantial plasma concentrations of 14C at the first time point of 10 min postdose and quantifiable concentrations at all time points thereafter. The time course of the plasma concentrations showed a biphasic behaviour. A first portion of the dose of was rapidly absorbed from the gastrointestinal tract immediately after dosing without a recognisable lag-time and reached a maximum concentration (Cmax) of 186 mg/kg after 20 min. This value corresponded to 19.4% of the equidistribution concentration of the administered dose. Until 4 hours after dosing, the concentration dropped to about 10 mg/kg which indicated a fast elimination of the test item related radioactivity from the central compart¬ment (blood) towards the peripheral compartments (organs and tissues; 1st elimination phase). A second increase of the TRR-values to approx. 65 mg/kg until 24 hours was measured thereafter followed by a decrease to a final value of 17 mg/kg of 72 hours post dosing (2nd elimination phase). An apparent saturation of absorption due to the high administered amount during the first phase might have been the reason for this biphasic behaviour. An exact value for the absorption rate could not be taken from these figures. But taking the urinary excretion behaviour and the remaining radioactivity in the body at sacrifice into account it can be concluded that at least 37% of the administered dose becomes systemically available.
The half-life for the first elimination phase (t1/2 e1) which lasted from 40 min to 4 h postdose amounted to 0.7 h. For the second elimination phase from 24 to 72 h postdose, the terminal half-life (t1/2 e2) and the terminal elimination rate accounted for 25 h and 0.028 [h-1], respectively. The mean area under the curve from 0.16 - 4 h (AUC 0.16 - 4) was 296 mg x h/kg (9%), from 4 - 72 h (AUC 4 - 72) 2393 mg x h/kg (72%), and the mean AUC extrapolated from 72 h to infinity (AUC 72 - ∞ was 602 mg x h/kg (18%). As shown for the second AUC-value from 4 - 72 h postdose, a similar high value was calculated for the respective mean area under the data value (AUD 4 - 72) of 2393 mg x h/kg. The second absorption and elimination phases contributed obviously mainly to the systemic exposure of the test item and/or its metabolites. The mean residence time (MRT 0 - ∞) of 45 h was relatively high but in view of a low accumulation factor of 1.4 a retention of test item related radioactivity in the body could be excluded.
Excretion
The excretion of radioactivity via urine and faeces was fast and almost completed at 24 h after administration. The by far major part of the dose (68.7%) was excreted with faeces and 34.7% with urine.
Radioactive residues in organs and tissues at sacrifice
The total radioactive residues in the organs and tissues were determined at sacrifice. In relation to the administered dose, only 2.2% were found in the total body. The carcass, skin and liver contributed in decreasing order mostly to this value. The relatively highest equivalent concentrations were detected in the liver (50 mg/kg), followed by kidney (36 mg/kg) and skin (25 mg/kg). For all other samples (erythrocytes, plasma, carcass and GIT), the values were below 20 mg/kg. Because of the high the renal and faecal excretion rate it is expected that the small amounts of residual radioactivity in the organs and tissues of the rat are subject to further elimination.
Metabolism
The metabolism of [14C]IDS, Na4 salt was investigated in selected urine and faeces samples. The radio-HPLC-profiles were very similar and highly comparable to each other. Significant qualitative differences did not occur. The identification rate for the unchanged IDS, Na4 salt was high and amounted 99.5% for urine (0 - 72 h) and 93.3% for faeces (0 - 24 h). Relating to the administered dose IDS, Na4 salt amounted to 34.5% in urine and 61.2% in faeces. A biotransformation pathway was not proposed because no single unknown metabolite was higher than 0.2% in urine and 2% in faeces.
Determination of osmolality, pH and metals in urine and plasma samples
The osmolality, pH-values and amount of selected metals in urine and plasma samples from rats which received nearly the same amount of the unlabelled IDS, Na4 salt (test 2) were additionally determined.
The administration of IDS, Na4 led to transient changes of the urinary osmolality- and pH-values within the test period of 3 days. Based on a high variability of urine pH- and osmolality-values in animal species used in toxicological studies [1], these effects however were regarded as insignificant.
The osmolality of the 4 hour urine samples from the treated rats were by a factor of 6.2 lower (mean of 143 mOsmol/kg) than the control urine sample from untreated rats (884 mOsmol/kg). After about 8 hours, the values increased clearly together with the total urine volume and entered into a plateau phase at about 24 h postdose. The highest value measured at 48 hours after administration (568 mOsmol/kg). The curve progression was similar to the urinary excretion curve which showed that an assumed osmotic imbalance was recovered within this period probably due to the final excretion of the unchanged test item. The plasma value of 314 mOsmol/kg at sacrifice showed no discrepancy to a respective value obtained from untreated rats (302 mOsmol/kg).
The time courses of metals in urine samples showed clear differences. Sodium was reduced by nearly the half compared to the reference-value until 4 hours postdose. It increased significantly until 24 h postdose to a peak level of about 1700 mg/L before it declined to 686 mg/L at sacrifice. The curve progression was comparable to the plasma curve of the test item related radioactivity. After low levels at 4 hours, a steady increase until test end was determined for calcium and also for magnesium until 48 h postdose. The final values for these two metals were higher than the respective reference-values. The 4 to 24 hours values for iron and zinc were higher than the reference values. They declined afterwards until test end. The final values were comparable to the reference values for zinc and higher for iron.
For the respective plasma data from the treated and untreated rats, slight differences were recognized for the metals likewise. Compared to the reference values, those for calcium and iron were by a factor of 1.3 higher and those for sodium and zinc by a factor of 1.2 lower. Equal amounts were measured for magnesium.
- Endpoint:
- basic toxicokinetics, other
- Type of information:
- read-across from supporting substance (structural analogue or surrogate)
- Adequacy of study:
- supporting study
- Reliability:
- 2 (reliable with restrictions)
- Rationale for reliability incl. deficiencies:
- data from handbook or collection of data
- Objective of study:
- absorption
- distribution
- excretion
- Details on absorption:
- Calcium is absorbed by active transport (transcellularly) and by passive diffusion (paracellularly) across the intestinal mucosa. Active transport of calcium is dependent on the action of calcitriol and the intestinal vitamin D receptor (VDR). This transcellular mechanism is activated by calcitriol and accounts for most of the absorption of calcium at low and moderate intake levels. Transcellular transport occurs primarily in the duodenum where the VDR is expressed in the highest concentration, and is dependent on up-regulation of the responsive genes including the calcium transport protein called transient receptor potential cation channel, vanilloid fam¬ily member 6 or TRPV6 (Li et al., 1993; Xue and Fleet, 2009 cited in DRI, 2011). These features—up-regulation of VDR and TRPV6—are most obvious during states in which a high efficiency of calcium absorption is required.
Passive diffusion or paracellular uptake involves the movement of cal¬cium between mucosal cells and is dependent on luminal:serosal electro¬chemical gradients. Passive diffusion occurs more readily during higher calcium intakes (i.e., when luminal concentrations are high) and can oc¬cur throughout the length of the intestine (Ireland and Fordtran, 1973). However, the permeability of each intestinal segment determines passive diffusion rates. The highest diffusion of calcium occurs in the duodenum, jejunum, and ileum (Weaver and Heaney, 2006b cited in DRI, 2011).
Fractional calcium absorption varies during critical periods of life. In infancy, it is high at approximately 60 percent, although the range is large (Fomon and Nelson, 1993; Abrams et al., 1997). Calcium absorption in newborns is largely passive and facilitated by the lactose content of breast milk (Kocian et al., 1973; Kobayashi et al., 1975). As the neonate ages, pas¬sive absorption declines and calcitriol-stimulated active intestinal calcium absorption becomes more important (Ghishan et al., 1980; Halloran and DeLuca, 1980; Ghishan et al., 1984). From a recent series of controlled metabolic studies, mean calcium absorption in adult men and non-pregnant women — across a wide age range — has been demonstrated to be approximately 25% of calcium intake (Hunt and Johnson, 2007). With aging and after menopause, fractional calcium absorption has been reported to decline on average by 0.21 percent per year after 40 years of age (Heaney et al., 1989). Nordin et al. (2004) and Aloia et al. (2010) also reported decreased absorption with age. - Details on distribution in tissues:
- Calcium ions Ca2+ derived from decomposed Ca(2Na)IDHA in acidic condition of stomach, after absorption to serum, fist are deposited into the organic matrix of skeleton. The skeleton is the principal depot for calcium, containing 98% of total body calcium. It can be called on repeatedly, through the processes of bone formation and resorption, to maintain circulating levels of calcium at a constant level. A principal physiological function of calcium apart from its role in maintaining the skeleton, is as an essential intracellular messenger in cells and tissues throughout the body. Although this pool of calcium is quanti¬tatively small, the ionized calcium present in the circulatory system, extra¬cellular fluid, muscle, and other tissues, is critical for mediating vascular contraction and vasodilatation, muscle function, nerve transmission, and hormonal secretion. Ionized calcium is the most common signal transduc¬tion element in biology, owing to its ability to reversibly bind to proteins and to complex with anions such as citrate and bicarbonate (Weaver and Heaney, 2006b cited in DRI, 2011).
- Details on excretion:
- The excretion of calcium in humans occurs mainly via the urine and faeces, but also in other body tissues and fluids, such as sweat. Calcium excretion in the urine is a function of the balance between the calcium load filtered by the kidneys and the efficiency of reabsorption from the renal tubules. Nearly 98% of filtered calcium (i.e., glomerular filtrate) is reabsorbed by either passive or active processes occurring at four sites in the kidney, each con¬tributing to maintaining neutral calcium balance. Overall, a typical daily calcium loss for a healthy adult man or woman via renal excretion is 5 mmol/day (Weaver and Heaney, 2006a). Calcium is excreted through the feces as unabsorbed intestinal cal¬cium and is shed in mucosal cells and secretions including saliva, gastric juices, pancreatic juice, and bile. Endogenous fecal calcium losses are approximately 2.1 mg/kg per day in adults and about 1.4 mg/kg per day in children (Abrams et al., 1991). These intestinal losses as well as minor losses in sweat are referred to collectively as endogenous calcium excretion. Endogenous calcium excretion, in contrast to urinary excretion, does not change appreciably with aging (Heaney and Recker, 1994).
- Conclusions:
- From a recent series of controlled metabolic studies, mean calcium absorption in adult men and non-pregnant women — across a wide age range — has been demonstrated to be approximately 25% of calcium intake.
Calcium ions Ca2+ after absorption to serum, fist are deposited into the organic matrix of skeleton. The skeleton is the principal depot for calcium, containing 98% of total body calcium. Although this pool of calcium is quanti¬tatively small, the ionized calcium present in the circulatory system, extra¬cellular fluid, muscle, and other tissues, is critical for mediating vascular contraction and vasodilatation, muscle function, nerve transmission, and hormonal secretion.
The excretion of calcium in humans occurs mainly via the urine and faeces, but also in other body tissues and fluids, such as sweat. - Executive summary:
Calcium as a nutrient is most commonly associated with the formation and metabolism of bone. Over 99% of total body calcium is found as calcium hydroxyapatite (Ca10[PO4]6[OH]2) in bones and teeth, where it provides hard tissue with its strength. Calcium in the circulatory system, extracellular fluid, muscle, and other tissues is critical for mediating vascular contraction and vasodilatation, muscle function, nerve transmission, intracellular signaling, and hormonal secretion. Bone tissue serves as a reservoir for and source of calcium for these critical metabolic needs through the process of bone remodeling.(DRI, 2011). Calcium metabolism is regulated in large part by the parathyroid hormone (PTH)–vitamin D endocrine system, which is characterized by a series of homeostatic feedback loops.
Calcium is absorbed by active transport (transcellularly) and by passive diffusion (paracellularly) across the intestinal mucosa. Active transport of calcium is dependent on the action of calcitriol and the intestinal vitamin D receptor (VDR). This transcellular mechanism is activated by calcitriol and accounts for most of the absorption of calcium at low and moderate intake levels. Transcellular transport occurs primarily in the duodenum where the VDR is expressed in the highest concentration, and is dependent on up-regulation of the responsive genes including the calcium transport protein called transient receptor potential cation channel, vanilloid family member 6 or TRPV6.
Passive diffusion or paracellular uptake involves the movement of calcium between mucosal cells and is dependent on luminal:serosal electrochemical gradients. Passive diffusion occurs more readily during higher calcium intakes (i.e., when luminal concentrations are high) and can occur throughout the length of the intestine (Ireland and Fordtran, 1973). However, the permeability of each intestinal segment determines passive diffusion rates. The highest diffusion of calcium occurs in the duodenum, jejunum, and ileum (Weaver and Heaney, 2006b cited in DRI, 2011).
From a recent series of controlled metabolic studies, mean calcium absorption in adult men and non-pregnant women — across a wide age range — has been demonstrated to be approximately25%of calcium intake.
Calcium ions Ca2+ after absorption to serum, fist are deposited into the organic matrix of skeleton.The skeleton is the principal depot for calcium, containing 98% of total body calcium. It can be called on repeatedly, through the processes of bone formation and resorption, to maintain circulating levels of calcium at a constant level.A principal physiological function of calcium apart from its role in maintaining the skeleton, is as an essential intracellular messenger in cells and tissues throughout the body.
The excretion of calcium in humans occurs mainly via the urine and faeces,but also in other body tissues and fluids, such as sweat. Calcium excretion in the urine is a function of the balance between the calcium load filtered by the kidneys and the efficiency of reabsorption from the renal tubules. Nearly 98% of filtered calcium (i.e., glomerular filtrate) is reabsorbed by either passive or active processes occurring at four sites in the kidney, each contributing to maintaining neutral calcium balance.Overall, a typical daily calcium loss for a healthy adult man or woman via renal excretion is 5 mmol/day (Weaver and Heaney, 2006a).Calcium is excreted through the feces as unabsorbed intestinal calcium and is shed in mucosal cells and secretions including saliva, gastric juices, pancreatic juice, and bile. Endogenous fecal calcium losses are approximately 2.1 mg/kg per day in adults and about 1.4 mg/kg per day in children (Abrams et al., 1991).
- Endpoint:
- basic toxicokinetics
- Type of information:
- other: statement
- Adequacy of study:
- key study
- Reliability:
- 2 (reliable with restrictions)
- Rationale for reliability incl. deficiencies:
- other: An extended assessment of the toxicokinetic behaviour of Cu(2Na)IDHA was performed, taking into account the chemical structure, the available physico-chemical-data and the available toxicity data.
- Objective of study:
- absorption
- distribution
- excretion
- metabolism
- Qualifier:
- according to guideline
- Guideline:
- other: TGD, Part I, Annex IV, 2003); ECHA guidance R7c., 2012
- Deviations:
- no
- Principles of method if other than guideline:
- An assessment of toxicological behaviour of Ca(2Na)IDHA is based on its physico-chemical properties and on the results of available toxicity data data.
- GLP compliance:
- no
- Type:
- absorption
- Results:
- GI absorption of 37 and 25 % for the chelating agent IDHA and free calcium, respectively, are reported. Dermal absorption is very low and absorption via inhalation is confined to the amount deposited in upper airways which can be swallowed.
- Type:
- distribution
- Results:
- Distribution of decomposed products absorbed from the gastrointestinal tract is expected to be extensive throughout the body. However, no wide distribution is expected in case of dermal or inhalation exposure.
- Type:
- excretion
- Results:
- Ca(2Na)IDHA and released free IDHA and calcium are expected to be excreted mainly via the faeces. IDHA was excreted with faeces (61.2 %) and 34.5 % with urine. Calcium are also excreted by body fluids, such as swet.
- Details on absorption:
- Oral absorption is favoured for small (with MW below 500 g/mol) water soluble molecules. Molecular weight of CaIDHA is 331.18 g/mol pointing to a possible absorption in the gastrointestinal (GI) tract while good water solubility (695.83 g/L) and the very low logPow value (-10.15) suggest that Ca(2Na)IDHA may be too hydrophilic to be readily absorbed via GI tract. The substance may be taken up by passive diffusion. As the substance’s molecular weight is lower than 500, it is likely to pass through aqueous pores or be carried through the gastrointestinal epithelial barrier by the bulk passage of water. However, the substance is instable under acidic condition (by pH under 5; PPC ADOB, 2016, own data not published). Therefore, the complex Ca(2Na)IDHA is expected to be in de-chelated form in the stomach: sodium ions, released Ca2+ ions from the complex and free IDHA. In lower part of stomach and small intestines, free IDHA can chelate again calcium or other metal ions because of increased pH. The binding of other metal cations like Fe, Zn, Mg, Cu ect. is quite possible because of a higher affinity of IDHA moiety to this cations (please see Table 6). Thus, oral absorption of chelated Ca(2Na)IDHA will result from absorption of calcium ions, sodium ions and free IDHA. Additionally, toxicity data on free IDHA and Ca(2Na)EDTA can elucidate the pattern and rate of absorption.
With regard to the toxicity and ADME data on free IDHA chelating agent:
In the acute oral and sub-acute oral studies, conducted with IDHA chelating agent (in form of its sodium salt), only minimal effects were noted in treated animals. LD50 of greater than 2000 mg/kg bw in the acute study and NOAEL of 1000 mg/kg bw and 200 mg/kg bw were established for females and males in the sub-acute study, respectively (Stropp, 1996, Report No. T4060981; Stropp and Popp, 1997; Report No. PH 26446). The effects in males demonstrate certain absorption of IDHA but it also shows its relatively low intrinsic toxicity. In the toxicokinetic study, the chelating agent IDHA was extensively absorbed and at least 37% of the administered dose became systemically available in rats (Koester, 2007; Report No. M 81819180).
With regard to the absorption data on free EDTA and Ca(2Na)EDTA metal complexes:
The toxicokinetics study with EDTA sodium salt and Ca(2Na)EDTA were conducted in animals and in humans. The study in animals: following oral administration of the calcium salt of 14C-EDTA (50 mg/kg bw) to rats the chelate was poorly absorbed from the gastrointestinal tract (2 to 18% within 24 hours). After parenteral injection of a comparable dose, 95 to 98% radioactivity was excreted in the urine within 6 hours with an elimination half-life of approximately 50 minutes (Foreman et al., 1953 cited in RAR, 2004).
Foreman and Trujillo (1954 cited in RAR, 2004) have studied the toxicokinetics of 14C-EDTA, (CaNa2EDTA; 2 mg) using young healthy adult men. Studies were carried out after peroral and parenteral application and after application on the skin. EDTA is poorly absorbed from the gastrointestinal tract. 24 hours after oral application, a maximum of 5% of the dose was detected in the urine; faeces still contained the substance up until day three (RAR, 2004).
With regard to the absorption data on free metal cations released from EDTA or IDHA metal complexes:
Regarding absorption of released calcium from both IDHA and EDTA, the rates of their absorption are assumed to be dependent on the pH optimum at which chelated agents form efficiently complexes with metal cations. For instance, taking into account the stability constant of the calcium EDTA complex (about 1010.M-1) the concentrations of free anionic EDTA species in CaNa2EDTA solutions can be estimated to amount < 0.01% according to the mass action law. Thus, almost all proportion of the CaNa2EDTA complex is still present as CaEDTA2- species, whereas only a very minor proportion of the CaNa2EDTA complex exists as free anionic EDTA species in solution which is considered to be too low for detecting generally toxic (systemic) effects of EDTA or Na4EDTA. The CaNa2EDTA will chelate any other metal that has a higher binding affinity than Ca2+ (e.g. lead, iron, zinc, and copper) (RAR, 2004).
Ca(2NA)IDHA complex in environment with pH below 5 (e.g. in stomach) decompose on Ca2+ and Na+ cations and anions IDHA4- and/or IDHANa3-. The proportion between ions depend on pH. In acid solutions, the proportion of IDHA anions that can form complexes declines as a result of progressive protonation. In the alkaline range, many metal ions form hydrocomplexes that influence the concentration of metal ions. For calcium the optimum pH for binding with IDHA anions is between 6 and 14 with stability constant 5.2 (Baypure DS leaflet, Bayer). Because other cations (e.g. Fe3+, Zn2+, Cu2+) have higher stability constants in similar pH range, it is very possible that they will compete with calcium cations and will replace them.
With regard to calcium:
Calcium as a nutrient is most commonly associated with the formation and metabolism of bone. Over 99% of total body calcium is found as calcium hydroxyapatite (Ca10[PO4]6[OH]2) in bones and teeth, where it provides hard tissue with its strength. Calcium in the circulatory system, extracellular fluid, muscle, and other tissues is critical for mediating vas¬cular contraction and vasodilatation, muscle function, nerve transmission, intracellular signaling, and hormonal secretion. Bone tissue serves as a res¬ervoir for and source of calcium for these critical metabolic needs through the process of bone remodeling.(DRI, 2011). Calcium metabolism is regulated in large part by the parathyroid hor¬mone (PTH)–vitamin D endocrine system, which is characterized by a series of homeostatic feedback loops.
Calcium is absorbed by active transport (transcellularly) and by passive diffusion (paracellularly) across the intestinal mucosa. Active transport of calcium is dependent on the action of calcitriol and the intestinal vitamin D receptor (VDR). This transcellular mechanism is activated by calcitriol and accounts for most of the absorption of calcium at low and moderate intake levels. Transcellular transport occurs primarily in the duodenum where the VDR is expressed in the highest concentration, and is dependent on up-regulation of the responsive genes including the calcium transport protein called transient receptor potential cation channel, vanilloid fam¬ily member 6 or TRPV6 (Li et al., 1993; Xue and Fleet, 2009 cited in DRI, 2011). These features—up-regulation of VDR and TRPV6—are most obvious during states in which a high efficiency of calcium absorption is required.
Passive diffusion or paracellular uptake involves the movement of cal¬cium between mucosal cells and is dependent on luminal:serosal electro¬chemical gradients. Passive diffusion occurs more readily during higher calcium intakes (i.e., when luminal concentrations are high) and can oc¬cur throughout the length of the intestine (Ireland and Fordtran, 1973). However, the permeability of each intestinal segment determines passive diffusion rates. The highest diffusion of calcium occurs in the duodenum, jejunum, and ileum (Weaver and Heaney, 2006b cited in DRI, 2011).
Fractional calcium absorption varies during critical periods of life. In infancy, it is high at approximately 60 percent, although the range is large (Fomon and Nelson, 1993; Abrams et al., 1997). Calcium absorption in newborns is largely passive and facilitated by the lactose content of breast milk (Kocian et al., 1973; Kobayashi et al., 1975). As the neonate ages, pas¬sive absorption declines and calcitriol-stimulated active intestinal calcium absorption becomes more important (Ghishan et al., 1980; Halloran and DeLuca, 1980; Ghishan et al., 1984). From a recent series of controlled metabolic studies, mean calcium absorption in adult men and non-pregnant women — across a wide age range — has been demonstrated to be approximately 25% of calcium intake (Hunt and Johnson, 2007). With aging and after menopause, fractional calcium absorption has been reported to decline on average by 0.21 percent per year after 40 years of age (Heaney et al., 1989). Nordin et al. (2004) and Aloia et al. (2010) also reported decreased absorption with age.
Based on these data, it can be concluded that a moderate direct absorption across the gastrointestinal tract epithelium will occur when Ca(2Na)IDHA is applied orally. The complex Ca(2Na)IDHA is expected to decompose at very high acidic conditions of stomach (pH 1-3). In the upper intestines, calcium cations will tend to be complexed again with free ligand IDHA because pH reaches about 5 when complex formation started. Depend on presence other metal cations with higher affinity to IDHA moiety in this pH conditions e.g. Fe3+, Mn, Zn or Cu, these cation could compete with calcium and replace it. Therefore, the absorbed fraction will result from intact Ca(2Na)IDHA and its released components: Ca2+ ions and free IDHA. Intestinal uptake of released Ca2+ ions is approximately 25%, while intestinal uptake of sodium IDHA is at least 37% comparing to 5% of sodium EDTA. Therefore, oral absorption of intact calcium IDHA chelates is expected to be somewhat higher than oral absorption of calcium of EDTA complexes or free EDTA.
In conclusion, taking into account low absorption potential based on physico-chemical properties of chelate Ca(2Na)IDHA, its instability at low pH values, absorption of 25% for calcium and 37% for free IDHA, 50% oral absorption (as worst-case) for Ca(2Na)IDHA is considered appropriate in case of hazard assessment (DNEL derivation).
Absorption by inhalation:
Based on the low vapour pressure of Ca(2Na)IDHA, inhalation exposure is not likely. There are no particles with aerodynamic diameter less than 100 μm (Januszkiewicz K., 2017, Report No. 196/2017). Moreover, final product has a microgranulated form. Thus, it is very unlikely, that big amounts of the substance reach the lung. In case of dust formation, it is expected that 100% of the inhaled substance will be deposited in the upper respiratory tract, where the particles may be moved by mucociliary transport to the throat and where the substance is swallowed and, conclusively, enters the stomach. The particles are not expected to reach alveolar region. If the substance reaches the lung, it is not very likely that the substance is taken up rapidly (based on physical-chemical properties). The substance is expected to be predominantly in chelated form since pH of healthy lungs is between 7.38 and 7.43 (Effros and Chinard, 1969).
There no available data for inhalation toxicity for chelating agent IDHA and its salt, but Ca(2Na)EDTA was used for therapy in patients which were exposed for lead oxide by inhalation route. Ten patients received solution of Ca(2Na)EDTA in an aerosol in total dose 6.6 g or 9.6 g of calcium EDTA (Pertovic et al., 1960 cited in lanigen and Yamarik, 2002). Similar therapy by inhalation of CaNa2EDTA solution were also described by Horiuchi et al. (1961) and Ashabel et al. (1965), Tesinger and Srobarova (1956). All patients tolerated Ca(2Na)EDTA aerosol treatment well and gained a beneficial therapeutic effects.
Based on this information, the absorption by inhalation is expected to confine to the amount of Ca(2Na)IDHA deposited in upper airways which can be swallowed. Therefore, as worst case, 50% absorption by inhalation (similar with oral absorption) is considered appropriate for the purposes of hazard assessment (DNEL derivation). This absorption rate covers sufficiently also oral absorption of free IDHA chelating agent which is at least 37%.
Dermal absorption:
Similarly, based on physico-chemical properties of Ca(2Na)IDHA, the substance is not likely to penetrate skin to a large extent due to negative logPow: -10.15 and water solubility: 695.83 g/L. Water solubility between 100-10,000 mg/l can indicate moderate to high dermal uptake, but on the other hand log P values <–1 suggest that a substance is not likely to be sufficiently lipophilic to cross the stratum corneum, therefore dermal absorption is likely to be low. The molecular weight of 331.18 g/mol indicates theoretically a certain potential to penetrate the skin (< 500) but in case of such a hydrophilic substance it is rather unlikely. This is supported by the findings of acute dermal toxicity studies of the free IDHA and as well other IDHA chelate like Mn(2Na)IDHA, Fe(III)IDHA, Cu(II)IDHA and Zn(2Na)IDHA where no systemic toxicity after exposure via the skin was noted (LD50 > 2000 mg/kg bw; Stropp, 1997a, Report No. T3061600, Kropidło, 2013, Report No. DER-11/13, Szewczyk et al., 2007, Report No. OS-16/07; Kropidło, 2010, Report No. DER-13/10; Olek, 2008 report No. OS-18/08 part I). Moreover, an acute dermal irritation / corrosion study in the rabbit (according to OECD 404) for free IDHA, Cu(2Na)IDHA, Mn(2Na)IDHA, Fe(III)IDHA and Zn(2Na)IDHA did not demonstrate skin irritation (Leuschner, 1998 rep.No. R 7266; Kropidło, 2010; Sornat, 2013; Szewczyk et al., 2007, Report No. OS-16/07; Olek, 2008, Report No OS-18/08 part II). This information indicates that Ca(2Na)IDHA is unlikely to penetrate the skin. In a human study, EDTA-CaNa2 did not penetrate the skin, only 0.001% was absorbed within 24 hours of administration (RAR, 2004). Low absorption potential through the skin would also apply to free IDHA chelating agent due to its high hydrophilicity (water solubility 564 g/L; data for Baypure CX 100 (Bayer)).
Based on very low logPow values, good water solubility and absence of toxicity effects in animal studies conducted with different aminopolycarboxylate chelates: free IDHA, Fe(III)IDHA, Mn(2Na)IDHA, Cu(2Na)IDHA, Zn(2Na)IDHA and Ca(2Na)EDTA, a similar behaviour regarding absorption through the skin is expected for Ca(2Na)IDHA. Dermal absorption is considered to be negligible and equal to 10% (worst-case) as established for substances which meet criteria of low dermal absorption potential mentioned in ECHA guidance R7c. (the value used for hazard assessment: DNEL derivation). - Details on distribution in tissues:
- When reaching the body, only a limited amount of Ca(2Na)IDHA is expected to be available for distribution.
The minor amount absorbed into the body, will most likely exist only in the intravascular compartment (due to its low LogPow and high water solubility) and will not be distributed into the cells, as the cell membranes require a substance to be soluble also in lipids to be taken up. Toxicokinetics study for chelating agent IDHA (sodium salt) indicate that IDHA after absorption is not metabolised (the identification rate for the unchanged IDHA, Na4 salt was high and amounted 99.5% for urine (0 – 72 h) and 93.3% for faeces (0 – 24 h), but rapidly eliminated from the body via urine and faeces (Koester, 2007).
In a human study with Ca(2Na)EDTA, intravenously injected EDTA was excreted within 24 hours in the urine, 50% of the substance in the first hour and 90% within 7 hours (RAR, 2004). No test substance was detected in blood. On the other hand, Ca(2Na)EDTA was also excreted in the expired air in treated animals. This indicates a wide distribution potential and no accumulative potential.
Calcium ions Ca2+ derived from decomposed Ca(2Na)IDHA in acidic condition of stomach, after absorption to serum, fist are deposited into the organic matrix of skeleton. The skeleton is the principal depot for calcium, containing 98% of total body calcium. It can be called on repeatedly, through the processes of bone formation and resorption, to maintain circulating levels of calcium at a constant level. A principal physiological function of calcium apart from its role in maintaining the skeleton, is as an essential intracellular messenger in cells and tissues throughout the body. Although this pool of calcium is quanti¬tatively small, the ionized calcium present in the circulatory system, extra¬cellular fluid, muscle, and other tissues, is critical for mediating vascular contraction and vasodilatation, muscle function, nerve transmission, and hormonal secretion. Ionized calcium is the most common signal transduc¬tion element in biology, owing to its ability to reversibly bind to proteins and to complex with anions such as citrate and bicarbonate (Weaver and Heaney, 2006b cited in DRI, 2011).
Based on these data and taking into account the fact, that “substances with LogPow values of 3 or less would be unlikely to accumulate with the repeated intermittent exposure patterns normally encountered in the workplace” (TGD, Part 1; ECHA guidance R.7C, 2012), no enhanced risk for accumulation will be associated with the target substance Ca(2Na)IDHA. - Details on excretion:
- In analogy with free IDHA, sodium salt, Ca(Na2)EDTA or other salts of EDTA it is assumed that most of Ca(2Na)IDHA will be excreted unchanged in the chelated form mainly in the faeces. Small amount of absorbed fraction will be also excreted unchanged via the urine. The excretion pattern of IDHA were similar to those observed for Ca(Na2)EDTA (RAR, 2004). The excretion via urine and faeces was fast and almost completed at 24 h after administration. The major part of the administered dose (68.7 %) was excreted with faeces and 34.7% with urine (Koester, 2007).
The excretion of calcium in humans occurs mainly via the urine and faeces, but also in other body tissues and fluids, such as sweat. Calcium excretion in the urine is a function of the balance between the calcium load filtered by the kidneys and the efficiency of reabsorption from the renal tubules. Nearly 98% of filtered calcium (i.e., glomerular filtrate) is reabsorbed by either passive or active processes occurring at four sites in the kidney, each con¬tributing to maintaining neutral calcium balance. Overall, a typical daily calcium loss for a healthy adult man or woman via renal excretion is 5 mmol/day (Weaver and Heaney, 2006a). Calcium is excreted through the feces as unabsorbed intestinal cal¬cium and is shed in mucosal cells and secretions including saliva, gastric juices, pancreatic juice, and bile. Endogenous fecal calcium losses are approximately 2.1 mg/kg per day in adults and about 1.4 mg/kg per day in children (Abrams et al., 1991). These intestinal losses as well as minor losses in sweat are referred to collectively as endogenous calcium excretion. Endogenous calcium excretion, in contrast to urinary excretion, does not change appreciably with aging (Heaney and Recker, 1994). - Metabolites identified:
- yes
- Details on metabolites:
- No metabolites are expected for Ca(2Na)IDHA. In case of released IDHA, the substance was stable and no metabolites were detected in significant amounts in the excreta in the toxicokinetic study (Koester, 2007). With the aid of skin metabolism simulator, a tool of the OECD QSAR Toolbox (v3.2, 2013), several metabolites were predicted for IDHA. They are all intermediates in mammals: oxaloacetate, aspartic acid, ß-alanine and pyruvate.
- Conclusions:
- In conclusion, taking into account absorption potential based on physico-chemical properties of chelate Ca(2Na)IDHA, its instability at low pH values, absorption of 25 % for calcium and 37% for free IDHA, 50% oral absorption (as worst-case) for Ca(2Na)IDHA is considered appropriate in case of hazard assessment (DNEL derivation).
Ca(2Na)IDHA is not expected to be metabolised but it will be excreted in its chelated state with calcium from the body.
The most of Ca(2Na)IDHA will be excreted unchanged in the chelated form mainly in the feaces. Small amount of absorbed fraction will be also excreted unchanged via urine. The excretion of IDHA, sodium salt via urine and feaces was fast and almost completed at 24h after administration. The by far major part of the dose (68.7%) was excreted with feaces and 34.7% with urine (Koester, 2007).
The excretion of calcium in humans occurs mainly via the urine and faeces, but also in other body tissues and fluids, such as sweat. - Executive summary:
Ca(2Na)IDHA is expected to be moderately absorbed after oral exposure, based on its high water solubility, negative logPow as well as absorption rates known for free IDHA and calcium. The substance is poorly available for inhalation since it is in a micro granulated form with particles above 100 µm, has a low vapour pressure, and is highly hydrophilic. Ca(2Na)IDHA is also not expected to be absorbed following dermal exposure into the stratum corneum and into the epidermis, due to its high water solubility. Concerning its distribution in the body Ca(2Na)IDHA, if absorbed, is expected to be distributed mainly in the intravasal compartment, due to its high water solubility, and may be also transported to the liver. The substance does not indicate a significant potential for accumulation. Ca(2Na)IDHA is not expected to be significantly metabolised but to be eliminated unchanged via the bile and, to a lesser extent, via the urine.
Referenceopen allclose all
Table 4 . Test 1 : Time courses of the equivalent and dose normalized concentrations in plasma of male rats after administration of 1000 mg [1 4C]IDS, Na4 salt/kg bw
Equivalent concentration С [mg/kg] |
|||||||
Time |
Animal no. |
||||||
(h p. admin.) |
347 |
348 |
349 |
350 |
351 |
Mean |
CV |
0.16 |
125.0 |
82.66 |
153.0 |
102.0 |
189.0 |
130.0 |
32.2 |
0.33 |
178.0 |
138.0 |
182.0 |
192.0 |
239.0 |
186.0 |
19.5 |
0.66 |
185.0 |
142.0 |
163.0 |
198.0 |
221.0 |
182.0 |
16.8 |
1.0 |
172.0 |
130.0 |
134.0 |
169.0 |
212.0 |
163.0 |
20.4 |
15 |
108.0 |
84.57 |
88.55 |
114.0 |
135.0 |
106.0 |
19.2 |
2.0 |
56.55 |
36.3 |
42.63 |
45.92 |
52.08 |
46.7 |
17.0 |
3.0 |
24.96 |
13.68 |
12.83 |
13.05 |
16.90 |
16.28 |
31.4 |
4.0 |
11.50 |
9.15 |
9.53 |
8.50 |
12.27 |
10.19 |
15.8 |
6.0 |
8.15 |
16.41 |
7.65 |
6.65 |
52.11 |
18.19 |
106.4 |
8.0 |
12.19 |
47.16 |
9.03 |
7.63 |
12.71 |
17.74 |
93.5 |
24.0 |
63.36 |
102.00 |
57.69 |
54.51 |
44.85 |
64.51 |
34.2 |
32.0 |
38.00 |
85.29 |
44.29 |
50.04 |
39.42 |
51.41 |
38.0 |
48.0 |
19.13 |
48.65 |
25.21 |
26.96 |
23.26 |
28.64 |
40.4 |
56.0 |
17.64 |
45.13 |
21.80 |
24.17 |
21.34 |
26.02 |
42.1 |
72.0 |
11.67 |
29.70 |
15.30 |
16.34 |
14.21 |
17.44 |
40.5 |
Dose normalized concentration CN |
|||||||
Time |
Animal no. |
||||||
(h p. admin.) |
347 |
348 |
349 |
350 |
351 |
Mean |
CV |
0.16 |
0.131 |
0.086 |
0.158 |
0.108 |
0.198 |
0.136 |
32.3 |
0.33 |
0.186 |
0.143 |
0.189 |
0.202 |
0.250 |
0.194 |
19.9 |
0.66 |
0.193 |
0.147 |
0.169 |
0.209 |
0.231 |
0.190 |
17.3 |
1.0 |
0.180 |
0.135 |
0.139 |
0.177 |
0.222 |
0.170 |
20.9 |
1.5 |
0.113 |
0.088 |
0.092 |
0.120 |
0.141 |
0.111 |
19.7 |
2.0 |
0.059 |
0.038 |
0.044 |
0.048 |
0.055 |
0.049 |
17.4 |
3.0 |
0.026 |
0.014 |
0.013 |
0.014 |
0.018 |
0.017 |
31.7 |
4.0 |
0.012 |
0.009 |
0.010 |
0.009 |
0.013 |
0.011 |
16.0 |
6.0 |
0.009 |
0.017 |
0.008 |
0.007 |
0.055 |
0.019 |
106.7 |
8.0 |
0.013 |
0.049 |
0.009 |
0.008 |
0.013 |
0.018 |
92.9 |
24.0 |
0.066 |
0.106 |
0.060 |
0.057 |
0.047 |
0.067 |
33.7 |
32.0 |
0.040 |
0.088 |
0.046 |
0.053 |
0.041 |
0.054 |
37.5 |
48.0 |
0.020 |
0.050 |
0.026 |
0.028 |
0.024 |
0.030 |
39.8 |
56.0 |
0.018 |
0.047 |
0.023 |
0.025 |
0.022 |
0.027 |
41.5 |
72.0 |
0.012 |
0.031 |
0.016 |
0.017 |
0.015 |
0.018 |
40.0 |
Table 5. Test 1 : Pharmacokinetic data analysis of the mean equivalent concentrations in plasma of male rats after administration of 1000 mg [14C]IDS, Na4 salt/kg bw
Pharmacokinetic non-compartmental data analysis |
||||
Time |
Measured |
Calculated |
Pharmacokinetic parameters |
|
(h p. admin.) |
value [mg/kg] |
value [mg/kg] |
||
0.16 |
130.0 |
— |
Сmах[mg/kg] |
186.0 |
0.33 |
186.0 |
— |
tmax[h] |
0.33 |
0.66 |
182.0 *) |
— |
t1/2 e1[h] |
0.73 |
1.0 |
163.0 *) |
— |
t1/2 e2 = terminal half-life) [h] |
25.14 |
1.5 |
106.0 *) |
— |
Terminal elimination rate [1/hour] = к |
0.028 |
2.0 |
46.7 *) |
— |
Intercept at time 0 [mg/kg] = С (0) |
120.9 |
3.0 |
16.28 *) |
— |
Correlation coefficient |
0.9841 |
4.0 |
10.19 *) |
— |
AUD(0.16-4)[mg x hour/kg] |
296.38 |
6.0 |
18.19 |
— |
AUD(4-24)[mg x hour/kg] |
722.31 |
8.0 |
17.74 |
— |
AUD(24-72)[mg x hour/kg] |
1670.40 |
24.0 |
64.51 **) |
62.4+) |
AUC(0.16-4)[mg x hour/kg] |
296.38 |
32.0 |
51.41 **) |
50.0+) |
AUC(0.16-4)[%] |
8.98 |
48.0 |
28.64 **) |
32.2+) |
AUD(4-72)[mg x hour/kg] |
2392.71 |
56.0 |
26.02 **) |
25.8+) |
AUD(4-72)[%] |
72.46 |
72.0 |
17.44 **) |
16.6+) |
AUD(72 -∞)[mg x hour/kg]++ |
602.40 |
AUD(72 ∞-)[%]++ |
18.24 |
|||
MRT (0-∞)[hour] |
45.26 |
|||
Accumulation factor (AF) |
1.36 |
Table 6. Test 1 : Balance of radioactivity in excreta as well as organs and tissues of male rats after administration of 1000 mg [1 4C]IDS, Na4 salt/kg bw
Radioactivity in percent of dose administered |
|||||||
|
Animal no. |
|
|
||||
|
347 |
348 |
349 |
350 |
351 |
Mean |
CV |
Urine |
43.20 |
18.27 |
25.32 |
28.38 |
58.18 |
34.67 |
46.1 |
Faeces |
59.86 |
75.95 |
83.93 |
82.21 |
41.30 |
68.65 |
26.2 |
Total excreted |
103.06 |
94.22 |
109.25 |
110.59 |
99.48 |
103.32 |
5.9 |
Skin |
0.38 |
1.01 |
0.63 |
0.65 |
0.61 |
0.66 |
34.7 |
Sum Organs |
0.91 |
2.02 |
1.39 |
1.40 |
1.21 |
1.38 |
29.3 |
Body without GIT |
1.29 |
3.03 |
2.01 |
2.04 |
1.82 |
2.04 |
31.0 |
GIT |
0.15 |
0.31 |
0.16 |
0.19 |
0.16 |
0.20 |
32.6 |
Total in body |
1.44 |
3.34 |
2.18 |
2.24 |
1.98 |
2.23 |
31.0 |
Balance |
104.50 |
97.55 |
111.40 |
112.80 |
101.50 |
105.60 |
6.2 |
Radioactivity in percent of dose recovered |
|||||||
|
Animal no. |
|
|
||||
|
347 |
348 |
349 |
350 |
351 |
Mean |
CV |
Urine |
41.34 |
18.73 |
22.73 |
25.15 |
57.34 |
33.06 |
48.6 |
Faeces |
57.28 |
77.85 |
75.32 |
72.86 |
40.71 |
64.80 |
24.2 |
Total excreted |
98.62 |
96.58 |
98.05 |
98.01 |
98.05 |
97.86 |
0.7 |
Skin |
0.36 |
1.04 |
0.56 |
0.57 |
0.60 |
0.63 |
39.5 |
Sum Organs |
0.87 |
2.07 |
1.24 |
1.24 |
1.19 |
1.32 |
33.7 |
Body without GIT |
1.23 |
3.11 |
1.81 |
1.81 |
1.79 |
1.95 |
35.5 |
GIT |
0.15 |
0.31 |
0.15 |
0.17 |
0.16 |
0.19 |
38.0 |
Total in body |
1.38 |
3.42 |
1.96 |
1.98 |
1.95 |
2.14 |
35.6 |
Norm.-factor |
0.96 |
1.03 |
0.90 |
0.89 |
0.99 |
0.95 |
6.2 |
Osmolality
The osmolality of the 4 hour urine samples from the treated rats were by a factor of 6.2 lower (mean of 143 mOsmol/kg) than the control urine sample from untreated rats (884 mOsmol/kg). After about 8 hours, the values increased clearly together with the total urine volume and entered into a plateau phase at about 24 h postdose. The highest value measured at 48 hours after administration (568 mOsmol/kg). The curve progression was similar to the urinary excretion curve which showed that an assumed osmotic imbalance was recovered within this period probably due to the final excretion of the unchanged test item. The plasma value of 314 mOsmol/kg at sacrifice showed no discrepancy to a respective value obtained from untreated rats (302 mOsmol/kg).
pH-Values and Metals
An only slight decrease of the pH-values of the urine samples from treated rats (pH 6.9 - 7.8) compared to a reference-value from untreated rats (pH 8.4) was measured.
The time courses of inorganic metals however showed clear differences. Sodium was reduced by nearly the half compared to the reference-value until 4 hours postdose. It increased significantly until 24 h postdose to a peak level of about 1700 mg/L before it declined to 686 mg/L at sacrifice. The curve progression was comparable to the plasma curve of the test item related radioactivity. After low levels at 4 hours, a steady increase until test end was determined for calcium and also for magnesium until 48 h postdose. The final values for these two metals were higher than the respective reference-values. The 4 to 24 hours values for iron and zinc were higher than the reference values. They declined afterwards until test end. The final values were comparable to the reference values for zinc and higher for iron. For the respective plasma data from the treated and untreated rats, slight differences were recognized for the metals only. Compared to the reference values, those for calcium and iron were by a factor of 1.3 higher and those for sodium and zinc by a factor of 1.2 lower. Equal amounts were measured for magnesium.
Description of key information
Ca(2Na)IDHA is expected to be moderately absorbed after oral exposure, based on its high water solubility, negative logPow as well as absorption rates known for free IDHA and calcium. The substance is poorly available for inhalation since it is in a micro granulated form with particles above 100 µm, has a low vapour pressure, and is highly hydrophilic. Ca(2Na)IDHA is also not expected to be absorbed following dermal exposure into the stratum corneum and into the epidermis, due to its high water solubility. Concerning its distribution in the body Ca(2Na)IDHA, if absorbed, is expected to be distributed mainly in the intravasal compartment, due to its high water solubility, and may be also transported to the liver. The substance does not indicate a significant potential for accumulation. Ca(2Na)IDHA is not expected to be significantly metabolised but to be eliminated unchanged mainly via the feaces and small amount via the urine.
Key value for chemical safety assessment
- Bioaccumulation potential:
- no bioaccumulation potential
- Absorption rate - oral (%):
- 50
- Absorption rate - dermal (%):
- 10
- Absorption rate - inhalation (%):
- 50
Additional information
Information on Registered Substances comes from registration dossiers which have been assigned a registration number. The assignment of a registration number does however not guarantee that the information in the dossier is correct or that the dossier is compliant with Regulation (EC) No 1907/2006 (the REACH Regulation). This information has not been reviewed or verified by the Agency or any other authority. The content is subject to change without prior notice.
Reproduction or further distribution of this information may be subject to copyright protection. Use of the information without obtaining the permission from the owner(s) of the respective information might violate the rights of the owner.
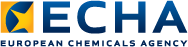