Registration Dossier
Registration Dossier
Data platform availability banner - registered substances factsheets
Please be aware that this old REACH registration data factsheet is no longer maintained; it remains frozen as of 19th May 2023.
The new ECHA CHEM database has been released by ECHA, and it now contains all REACH registration data. There are more details on the transition of ECHA's published data to ECHA CHEM here.
Diss Factsheets
Use of this information is subject to copyright laws and may require the permission of the owner of the information, as described in the ECHA Legal Notice.
EC number: 915-009-3 | CAS number: -
- Life Cycle description
- Uses advised against
- Endpoint summary
- Appearance / physical state / colour
- Melting point / freezing point
- Boiling point
- Density
- Particle size distribution (Granulometry)
- Vapour pressure
- Partition coefficient
- Water solubility
- Solubility in organic solvents / fat solubility
- Surface tension
- Flash point
- Auto flammability
- Flammability
- Explosiveness
- Oxidising properties
- Oxidation reduction potential
- Stability in organic solvents and identity of relevant degradation products
- Storage stability and reactivity towards container material
- Stability: thermal, sunlight, metals
- pH
- Dissociation constant
- Viscosity
- Additional physico-chemical information
- Additional physico-chemical properties of nanomaterials
- Nanomaterial agglomeration / aggregation
- Nanomaterial crystalline phase
- Nanomaterial crystallite and grain size
- Nanomaterial aspect ratio / shape
- Nanomaterial specific surface area
- Nanomaterial Zeta potential
- Nanomaterial surface chemistry
- Nanomaterial dustiness
- Nanomaterial porosity
- Nanomaterial pour density
- Nanomaterial photocatalytic activity
- Nanomaterial radical formation potential
- Nanomaterial catalytic activity
- Endpoint summary
- Stability
- Biodegradation
- Bioaccumulation
- Transport and distribution
- Environmental data
- Additional information on environmental fate and behaviour
- Ecotoxicological Summary
- Aquatic toxicity
- Endpoint summary
- Short-term toxicity to fish
- Long-term toxicity to fish
- Short-term toxicity to aquatic invertebrates
- Long-term toxicity to aquatic invertebrates
- Toxicity to aquatic algae and cyanobacteria
- Toxicity to aquatic plants other than algae
- Toxicity to microorganisms
- Endocrine disrupter testing in aquatic vertebrates – in vivo
- Toxicity to other aquatic organisms
- Sediment toxicity
- Terrestrial toxicity
- Biological effects monitoring
- Biotransformation and kinetics
- Additional ecotoxological information
- Toxicological Summary
- Toxicokinetics, metabolism and distribution
- Acute Toxicity
- Irritation / corrosion
- Sensitisation
- Repeated dose toxicity
- Genetic toxicity
- Carcinogenicity
- Toxicity to reproduction
- Specific investigations
- Exposure related observations in humans
- Toxic effects on livestock and pets
- Additional toxicological data

Endpoint summary
Administrative data
Key value for chemical safety assessment
Genetic toxicity in vitro
Description of key information
The substance is a reaction mixture of Manganese (Mn), EDTA, DTPA and HEEDTA.
Manganese and manganese salts (MnCl2, MnO2, MnSO4) are not classified as mutagenic in the ECHA dissemination database. Two literature reviews (Gerber et al. 2002, Assem et al. 2011) covering both in vitro and in vivo tests indicate that manganese compounds can have mutagenic effects at high concentrations, but the weight of evidence is not sufficient to consider manganese as mutagenic.
EDTA, DTPA and HEEDTA are classified as non-mutagenic on the ECHA dissemination website. To support this, a published negative Ames test for EDTA and QSAR results for EDTA, DTPA and HEEDTA are provided that predict negative outcomes of the Ames test with sufficient reliability.
Although there are indications that Mn possibly has some mutagenic properties, Mn is complexed by the chelationg agents and not bio-available in the reaction mass of MnEDTA, MnDTPA and MnHEEDTA. Therefore, any possible mutagenic effects of Mn are highly unlikely and, because of the non-mutagenic properties of EDTA, DTPA and HEEDTA, the reaction mass of MnEDTA, MnDTPA and MnHEEDTA can be considered as not mutagenic.
Link to relevant study records
- Endpoint:
- in vitro gene mutation study in bacteria
- Type of information:
- (Q)SAR
- Adequacy of study:
- weight of evidence
- Reliability:
- 2 (reliable with restrictions)
- Rationale for reliability incl. deficiencies:
- results derived from a valid (Q)SAR model and falling into its applicability domain, with adequate and reliable documentation / justification
- Justification for type of information:
- 1. SOFTWARE
VEGA v1.4.4
2. MODEL (incl. version number)
Mutagenicity (Ames test) model (CONSENSUS) 1.0.2.
3. SMILES OR OTHER IDENTIFIERS USED AS INPUT FOR THE MODEL
DTPA = C(CN(CC(=O)O)CC(=O)O)N(CCN(CC(=O)O)CC(=O)O)CC(=O)OC(CN(CC(=O)O)CC(=O)O)N(CCN(CC(=O)O)CC(=O)O)CC(=O)O
4. SCIENTIFIC VALIDITY OF THE (Q)SAR MODEL
[Explain how the model fulfils the OECD principles for (Q)SAR model validation. Consider attaching the QMRF or providing a link]
See attached QMRF
5. APPLICABILITY DOMAIN
[Explain how the substance falls within the applicability domain of the model]
See attached QMRF
6. ADEQUACY OF THE RESULT
[Explain how the prediction fits the purpose of classification and labelling and/or risk assessment]
An in-vitro gene mutation study in bacteria is required for REACH dossiers in the 1-10 tonnage band. This QSAR provides a prediciton for this endpoint. - Guideline:
- other: REACH Guidance on QSARs R.6
- Specific details on test material used for the study:
- SMILES: C(CN(CC(=O)O)CC(=O)O)N(CCN(CC(=O)O)CC(=O)O)CC(=O)O
- Remarks on result:
- no mutagenic potential (based on QSAR/QSPR prediction)
- Conclusions:
- Based on the CAESAR model for Mutagenicity (Ames test) v2.1.13, DTPA is non-mutagenic.
- Endpoint:
- in vitro gene mutation study in bacteria
- Type of information:
- (Q)SAR
- Adequacy of study:
- weight of evidence
- Reliability:
- 2 (reliable with restrictions)
- Rationale for reliability incl. deficiencies:
- results derived from a valid (Q)SAR model and falling into its applicability domain, with adequate and reliable documentation / justification
- Justification for type of information:
- 1. SOFTWARE
VEGA v1.4.4
2. MODEL (incl. version number)
Mutagenicity (Ames test) model (CONSENSUS) 1.0.2.
3. SMILES OR OTHER IDENTIFIERS USED AS INPUT FOR THE MODEL
HEEDTA = C(CN(CC(=O)O)CC(=O)O)N(CCO)CC(=O)O
4. SCIENTIFIC VALIDITY OF THE (Q)SAR MODEL
[Explain how the model fulfils the OECD principles for (Q)SAR model validation. Consider attaching the QMRF or providing a link]
See attached QMRF
5. APPLICABILITY DOMAIN
[Explain how the substance falls within the applicability domain of the model]
See attached QMRF
6. ADEQUACY OF THE RESULT
[Explain how the prediction fits the purpose of classification and labelling and/or risk assessment]
An in-vitro gene mutation study in bacteria is required for REACH dossiers in the 1-10 tonnage band. This QSAR provides a prediciton for this endpoint. - Guideline:
- other: REACH Guidance on QSARs R.6
- Specific details on test material used for the study:
- SMILES: C(CN(CC(=O)O)CC(=O)O)N(CCO)CC(=O)O
- Remarks on result:
- no mutagenic potential (based on QSAR/QSPR prediction)
- Conclusions:
- Based on the CAESAR model for Mutagenicity (Ames test) v2.1.13, HEEDTA is non-mutagenic.
- Endpoint:
- in vitro gene mutation study in bacteria
- Type of information:
- (Q)SAR
- Adequacy of study:
- weight of evidence
- Reliability:
- 2 (reliable with restrictions)
- Rationale for reliability incl. deficiencies:
- results derived from a valid (Q)SAR model and falling into its applicability domain, with adequate and reliable documentation / justification
- Justification for type of information:
- 1. SOFTWARE
VEGA v1.4.4
2. MODEL (incl. version number)
Mutagenicity (Ames test) model (CONSENSUS) 1.0.2.
3. SMILES OR OTHER IDENTIFIERS USED AS INPUT FOR THE MODEL
EDTA = C(CN(CC(=O)O)CC(=O)O)N(CC(=O)O)CC(=O)O
4. SCIENTIFIC VALIDITY OF THE (Q)SAR MODEL
[Explain how the model fulfils the OECD principles for (Q)SAR model validation. Consider attaching the QMRF or providing a link]
See attached QMRF
5. APPLICABILITY DOMAIN
[Explain how the substance falls within the applicability domain of the model]
See attached QMRF
6. ADEQUACY OF THE RESULT
[Explain how the prediction fits the purpose of classification and labelling and/or risk assessment]
An in-vitro gene mutation study in bacteria is required for REACH dossiers in the 1-10 tonnage band. This QSAR provides a prediciton for this endpoint. - Guideline:
- other: REACH Guidance on QSARs R.6
- Specific details on test material used for the study:
- SMILES: C(CN(CC(=O)O)CC(=O)O)N(CC(=O)O)CC(=O)O
- Remarks on result:
- no mutagenic potential (based on QSAR/QSPR prediction)
- Conclusions:
- Based on the CAESAR model for Mutagenicity (Ames test) v2.1.13, EDTA is non-mutagenic.
- Endpoint:
- genetic toxicity in vitro, other
- Remarks:
- review of available information
- Type of information:
- other: Review
- Adequacy of study:
- weight of evidence
- Reliability:
- 2 (reliable with restrictions)
- Rationale for reliability incl. deficiencies:
- data from handbook or collection of data
- Justification for type of information:
- This publication is a review of the mutagenecity and carcinogenecity of inorganic Mn compounds, based on all information (in vitro and in vivo studies) available in literature.
- Qualifier:
- no guideline followed
- Principles of method if other than guideline:
- This study is a review of all availble literature on the genotoxic properties of inorganic Mn compounds.
- Type of assay:
- other: Literature review
- Remarks on result:
- other: The current literature indicates that Mn may be weakly mutagenic in vitro and possibly clastogenic in vivo, with unknown genotoxic effects in humans
- Conclusions:
- A number of studies indicate that under in vitro conditions, inorganic Mn (presumably Mn2+) is weakly mutagenic, particularly to S. typhimurium strain TA102, but there remains a lack of clarity as to the nature of the underlying mechanism(s) of mutagenicity or whether there is an association with the expression of cytotoxic cellular responses.
There is no convincing evidence that Mn is mutagenic or clastogenic in vivo, and the available data from studies on fruit flies and rodents are predominantly negative.However, there is conflicting evidence of Mn clastogenicity, although it should be noted that the available published literature is of limited quality.
Overall, the weight of evidence from the available experimental studies suggests that Mn is probably clastogenic in vitro and may also be an in vivo clastogen. While a number of possible mechanisms have been suggested, the evidence is considered insufficient to establish their validity or relative significance. - Executive summary:
Manganese (Mn), a naturally occurring element present in many foodstuffs, is an essential trace element with many biological functions. In industry, inorganic Mn compounds have a range of different applications, although the majority of Mn is used to make alloys and steel. For the general population, the major source of exposure to Mn is dietary, although drinking water may constitute an additional source in some regions. However, in occupationally exposed humans, inhalation of Mn is likely to be an important additional route. In general, Mn and its inorganic compounds are considered to possess low mutagenic or carcinogenic potential compared with some heavy metals. In this review, an up-to-date analysis of the available published studies on the carcinogenic and genotoxic potential of inorganic Mn is provided (organic Mn compounds are not considered). The current literature indicates that Mn may be weakly mutagenic in vitro and possibly clastogenic in vivo, with unknown genotoxic effects in humans; the possible mechanisms underlying these effects are discussed. The experimental evidence on carcinogenicity (quantitative increase in incidence of thyroid tumors in mice but not rats) does not provide any clear evidence, while the available occupational and environmental epidemiological evidence is equivocal as to whether exposure to inorganic Mn is associated with a significant cancer risk. Hence, it is concluded that there is insufficient evidence to indicate that inorganic Mn exposure produces cancer in animals or humans.
- Endpoint:
- genetic toxicity in vitro, other
- Remarks:
- Review of available information
- Type of information:
- other: Review
- Adequacy of study:
- weight of evidence
- Reliability:
- 2 (reliable with restrictions)
- Rationale for reliability incl. deficiencies:
- data from handbook or collection of data
- Justification for type of information:
- This publication is a review of the mutagenecity, carcinogenecity and teratogenicity of Mn compounds, based on all information (in vitro and in vivo studies) available in literature.
- Qualifier:
- no guideline followed
- Principles of method if other than guideline:
- This study is a review of all availble literature on the genotoxic properties of Mn compounds.
- Type of assay:
- other: Literature review
- Remarks on result:
- other: In summary, some manganese compounds in the order MnCl2\KMnO4,\MnSO4 gave positive results in several short-term tests.
-
[47] De Meo M, Laget M, Castegnaro M, Duménil G. Genotoxic activity of potassium permanganate in acidic solutions. Mutat Res 1991;260:295–306.
- [48] Van de Sande JH, McIntosh IP, Jovin TN. Mn2+and other transition metals at low concentrations at low concentration induce the right-to-left helical transformation of poly d(G–C). EMBO J 1982;1:777–82.
-
[49] Beckman RA, Mildvan AS, Loeb LA. On thefidelity of DNA replication: manganese mutagenesis in vitro. Biochem 1985;24:5810–7.
-
[50] Scicchitano DA, Pegg AE. Inhibition of O6-alkylguanine- DNA-alkyltransferase by metals. Mutat Res 1987;192:207–10.
- [51] Olivier P, Marzin D. Study of the genotoxic potential of 48 inorganic derivatives with SOS chromotest. Mutat Res 1987;189:263–9.
-
[52] Bhanot OS, Solomon JJ. The role of mutagenic metal ions in mediating in vitro mispairing by alkylpyrimidines. Environ Health Perspect 1994;102(Suppl. 3):81–90.
[53] Orgel A, Orgel LE. Induction of mutations in bacteriophage T4 with divalent manganese. J Mol Biol 1965;14:453–7.
[54] Rossman T.G., Molina M., Meyer L.W. The genetic toxicology of metal compounds: I. Induction of lambda prophage inE coliWP2s(lambda), 1984;6:59–69.
[55] Rossman T.G., Molina M. The genetic toxicology of metal compounds: II. Enhancement of ultraviolet light-induced muta- genesis inEscherichia coliWP2, 1986;8:263–271.
[56] Nishioka H. Mutagenic activities of metal compounds in bacte- ria. Mutat Res 1975;31:185–9.
[57] Léonard A. Mechanisms in metal genotoxicity: the significance of in vitro approaches. Mutat Res 1988;198:321–6.
[58] De Flora S. Study of 106 organic and inorganic compounds in theSalmonella microsometest. Carcinogenesis 1981;2:283–98.
[59] Marzin DR, Vo Phi H. Study of the mutagenicity of metal derivatives withSalmonella typhimuriumTA102. Mutat Res 1985;155:49–51.
[60] Francis AR, Shetty TK, Bhattacharya RK. Modifying role of dietary factors on the mutagenicity of aflatoxin B1: in vitro effect of trace elements. Mutat Res 1988;199:85–93.
[61] Hatcher JF, Bryan GT. Factors affecting the mutagenic activity of quercetin forSalmonella typhimuriumTA98 metal ions, antioxidants and pH. Mutat Res 1985;148:13–23.
[62] Zakour RA, Glickman BW. Metal-induced mutagenesis in the lacI gene ofEscherichia coli. Mutat Res 1984;126:9–18.
[63] Putrament A, Baranowska H, Prazmo W. Induction by man- ganese of mitochondrial antibiotic resistance mutations in yeast 1973;126:357–66.
[64] Prazmo W, Balbin E, Baranowska H, Ejchart A, Putrament A. Manganese mutagenesis in yeast. II. Conditions of induction and characteristics of mitochondrial respiratory deficientSac-charomyces cere70Tisiaemutants induced with manganese and cobalt. Genet Res 1975;26:21–9.
[65] Putrament A, Bazanowska H, Eychart A, Jachymezyk W. Manganese mutagenesis in yeast VI Mn2uptake, mitDNA replication and ERinduction. Comparison with other divalent cations 1977;18:69–76.
[66] Slater ML. Effect of reversible inhibition of deoxyribonucleic acid synthesis on the yeast cell cycle. J Bacteriol 1973;113:263–70
-
[67]
Ogawa HI, Shibahara T, Iwata H, Okada T,
Tsuruta S, Kaki- moto K, Sakata K, Kato Y, Ryo
H, Itoh T, et al. Genotoxic activities in vivo
of cobaltous chloride and other metal chlo-
rides as assayed in the Drosophila wing spot
test. Mutat Res 1994;320:133–40.
[68]
Miyaki M, Akamatsu N, Ono T, Koyama H.
Mutagenicity of metal cations in cultured
cells from Chinese hamsters. Mutat Res
1979;68:259–63.
[69]
Oberly TJ, Piper CE, McDonald DS. Mutagenicity
of metal salts in the L5178 Y mouse lymphoma
assay. J Toxicol Environ Health 1982;9:367–76.
[70]
Casto BC, Meyers J, DiPaolo JA. Enhancement of
viral trans- formation for evaluation of the
carcinogenic or mutagenic potential of
inorganic metal salts. Cancer Res 1979;39:193–8.
[71]
Gläss
E. Untersuchungen über
die Einwirkung von Schwer- metallsalzen auf
die Wurzelspitzenmitose von Vicia faba.
Ztschrft Botan 1955;43:359–403.
[72]
Gläss
E. Untersuchungen über
die Einwirkung von Schwer- metallsalzen auf
die Wurzelspitzenmitose von Vicia faba.
Ztschrft Botan 1956;44:1–58.
[73]
Singh O.P. Effects of certain chemical
polluants on plant chromosomes [Dissertation].
University of Calcutta, 1979.
[74] Snyder RD. Role of active oxygen species in metal-induced DNA strand breakage in human diploidfibroblasts. Mutat Res 1988;193:237–46.[75] Tan EL, Williams MW, Schenkley RL, Perdue SW, Hayden TL, Turner JE, Hsie AW. The toxicity of sixteen metallic compounds in Chinese hamster ovary cells. Toxic Appl Phar- macol 1984;74:330–6.
- [77] Joardar M, Sharma A. Comparison of clastogenicity of inor- ganic Mn administered in cationic and anionic forms in vivo. Mutat Res 1990;240:159–63.
- Conclusions:
- Relatively high doses of manganese affect DNA replication and repair in bacteria and causes mutations in microorganism and mammalian cells although the Ames test does not appear to be particularly responsive to manganese. In mammalian cells, manganese causes DNA damage and chromosome aberrations. Informa- tion on organic manganese derivatives is still insufficient.
- Endpoint:
- in vitro gene mutation study in bacteria
- Type of information:
- experimental study
- Adequacy of study:
- weight of evidence
- Reliability:
- 2 (reliable with restrictions)
- Rationale for reliability incl. deficiencies:
- comparable to guideline study with acceptable restrictions
- Qualifier:
- equivalent or similar to guideline
- Guideline:
- OECD Guideline 471 (Bacterial Reverse Mutation Assay)
- GLP compliance:
- not specified
- Type of assay:
- bacterial reverse mutation assay
- Species / strain / cell type:
- S. typhimurium TA 1535, TA 1537, TA 98 and TA 100
- Species / strain / cell type:
- E. coli WP2 uvr A
- Metabolic activation:
- with and without
- Metabolic activation system:
- uninduced and arochlor induced liver S9 mix of male Fischer 344 rats, B6C3F1 mice, and Syrian hamsters
- Test concentrations with justification for top dose:
- 10, 33, 100, 333, 1000, 3333, 10000 µg/plate
- Vehicle / solvent:
- distilled water
- Untreated negative controls:
- no
- Negative solvent / vehicle controls:
- yes
- Remarks:
- distilled water
- True negative controls:
- no
- Positive controls:
- yes
- Positive control substance:
- 2-nitrofluorene
- N-ethyl-N-nitro-N-nitrosoguanidine
- other:
- Details on test system and experimental conditions:
- All plates were prepared in triplicate, and concurrent positive and negative controls were run at all times.
- Statistics:
- not needed
- Species / strain:
- S. typhimurium TA 1535
- Metabolic activation:
- with and without
- Genotoxicity:
- negative
- Cytotoxicity / choice of top concentrations:
- not specified
- Vehicle controls validity:
- valid
- Untreated negative controls validity:
- not examined
- Positive controls validity:
- valid
- Species / strain:
- S. typhimurium TA 1537
- Metabolic activation:
- with and without
- Genotoxicity:
- negative
- Cytotoxicity / choice of top concentrations:
- not specified
- Vehicle controls validity:
- valid
- Untreated negative controls validity:
- not examined
- Positive controls validity:
- valid
- Species / strain:
- S. typhimurium TA 98
- Metabolic activation:
- with and without
- Genotoxicity:
- negative
- Cytotoxicity / choice of top concentrations:
- not specified
- Vehicle controls validity:
- valid
- Untreated negative controls validity:
- not examined
- Positive controls validity:
- valid
- Species / strain:
- S. typhimurium TA 100
- Metabolic activation:
- with and without
- Genotoxicity:
- negative
- Cytotoxicity / choice of top concentrations:
- not specified
- Vehicle controls validity:
- valid
- Untreated negative controls validity:
- not examined
- Positive controls validity:
- valid
- Species / strain:
- E. coli WP2 uvr A
- Metabolic activation:
- with and without
- Genotoxicity:
- negative
- Cytotoxicity / choice of top concentrations:
- not specified
- Vehicle controls validity:
- valid
- Untreated negative controls validity:
- not examined
- Positive controls validity:
- valid
- Conclusions:
- Based on the Ames test, EDTA has no mutagenic properties.
- Endpoint:
- in vitro gene mutation study in mammalian cells
- Data waiving:
- study scientifically not necessary / other information available
- Justification for data waiving:
- other:
- Justification for type of information:
- The substance consists of Manganese and the chelating agents EDTA, DTPA and HEEDTA. Mn is an inorganic that is very common in nature and the human body and is typically not considered mutagenic. None of the chelating agents are classified as mutagenic on the ECHA dissemination website.
Because none of its constituents are considered mutagenic, MnEDTA/DTPA/HEEDTA is also not expected to have mutagenic properties and further tests are not required. - Endpoint:
- in vitro cytogenicity / chromosome aberration study in mammalian cells
- Data waiving:
- study scientifically not necessary / other information available
- Justification for data waiving:
- other:
- Justification for type of information:
- The substance consists of Manganese and the chelating agents EDTA, DTPA and HEEDTA. Mn is an inorganic that is very common in nature and the human body and is typically not considered mutagenic. None of the chelating agents are classified as mutagenic on the ECHA dissemination website.
Because none of its constituents are considered mutagenic, MnEDTA/DTPA/HEEDTA is also not expected to have mutagenic properties and further tests are not required.
Referenceopen allclose all
GENOTOXICITY
In Vitro Studies
Prokaryotic Test Systems
All identified studies on the prokaryotic mutagenicity of Mn compounds used Salmonella typhimurium bacteria as the test organism in a standard or modified Ames assay (Ames et al. 1975). Many compounds require metabolic activation to exhibit mutagenic activity; thus, Ames tests are generally performed in both the presence (+S9) and absence (–S9) of a metabolizing system (usually the S9 fraction of rat liver from Aroclor-preexposed animals). Studies were conducted both with and with- out metabolic activation using several strains of S. typhimurium that are predictive of different types of mutational event, e.g., TA100, TA1535 (base pair), TA97, TA97a, TA98, TA1537 (frameshift), YG1041, YG1042 (mutagenicity of aromatic nitroso compounds and aromatic amines), TA102, YG3003 (oxidative mutagenic- ity), and YG7108 (alkylating agent mutagenic- ity). Despite the underlying similarity of the test systems utilized, both positive and nega- tive results were reported. In a well-reported robust study conducted as part of the U.S. National Toxicology Program (NTP), MnSO4 was nonmutagenic to S. typhimurium strains TA97, TA98, TA100, TA1535, and TA1537 (±S9) in the standard Ames test (Mortelmans et al. 1986; NTP 1993). In contrast, in a poorly reported study by De Meo et al. (1991), potassium permanganate (KMnO4) under acidic or neutral conditions was negative in TA97 and TA98 (±S9) and in TA100 and TA102 (+S9), while a strong positive response was noted for strain TA102 (–S9). A positive response was also reported in TA100 in the presence of KMnO4 in organic solvent (acetone or ethanol) (–S9). The TA102 strain of S. typhimurium detects oxidative and cross-linking mutagens, and De Meo et al. (1991) attributed the observed mutagenic activity of KMnO4 to the generation of Mn2+ions. MnCl2 and MnSO4 were mutagenic to the TA102 strain (–S9) specifically using the microplate technique; activity was not noted in TA100 (De Meo et al. 1991).
Pagano and Zeiger (1992) reported a concentration-related increase in mutagenicity of MnSO4 (and a number of other divalent inorganic metal salts) in TA97 cells when incubated using distilled deionized water or 4-(2-hydroxyethyl)-1-piperazineethanesulfonic acid (HEPES)/saline buffer but not when phosphate buffer was used; the positive response was abolished by preincubation with various components of the Vogel–Bonner agar used in the standard test method (particularly citrate in
the case of Mn) or by co-incubation with the organic chelator diethyldithiocarbamate, which indicates reduced bioavailability of metal ions. Pagano and Zeiger (1992) suggested that Salmonella plate test models may be insensitive for the detection of metal mutagenicity.
MnCl2 tested positive for mutagenicity in S. typhimurium TA1537 in the absence of S9, but not in strains TA98, TA102, TA1535; no positive control data were reported (Wong 1988). Wong (1988) also suggested the lack of positive findings with S9 may have been due to binding of metal ions to metalloproteins present in the S9 mix resulting in reduced bioavailablity to the cells. The strongest, albeit limited, evidence of mutagenic activity relates principally to the TA102 strain, which is sensitive to compounds that produce reactive oxygen species (ROS) (Levin et al. 1982); this may be suggestive of an underlying mechanism of action. Several other studies investigated the mutagenic potential of various mixtures containing Mn (Al-Bataina et al. 2003; Orescanin et al. 2002; Tsukatani et al. 2002). However, these were not considered informative due to coexposures to other potentially mutagenic substances. Nonetheless, it is of interest to note that in one such study, the mutagenic activity of roadside soil samples containing various concentrations of heavy metals and polycyclic aromatic hydrocarbons (PAH) showed no correlation with soil Mn content (Tsukatani et al. 2002).
Eukaryotic Test Systems
Of the studies that investigated the in vitro genotoxic effects of Mn compounds in eukaryotic cells, two related specifically to mutagenic potential. In a study on yeast, MnSO4 was weakly mutagenic to Saccharomyces cerevisiae strain D7 (sensitive to gene conversion at the trp5 locus and to reverse mutation at the ilv1 locus) at concentrations at which some cytotoxicity was observed (Singh 1984). MnCl2 (5–100μg/ml) induced a concentration-related increase in the frequency of forward mutation of the thymidine kinase (TK) gene in L5178Y mouse lymphoma cells at concentrations above 10μg/ml in the absence of S9; data on activity in the presence of S9 were not presented (Oberly et al. 1982). At 10μg/ml Mn, the mutation frequency was 1.2-fold higher than the solvent control, rising to approximately 7- to 8-fold higher at 60μg/ml and above. The rise in mutation frequency was associated with a concentration- related increase incidence of cytotoxicity; cell survival was 100% at 10μg/ml, 82% at 20μg/ml, and decreased to 10% at 60 and 80μg/ml and to less than 10% at the highest concentration (Oberly et al. 1982).
A number of other studies reported effects on a wider range of endpoints in eukaryotic test systems. In a recent study, MnCl2 (25μM, equivalent to 5μg/ml) induced chromosomal aberrations (CA) (gaps and breaks) in cultured human lymphocytes treated during the G2 cell cycle phase and DNA damage (single- strand breaks [SSB] and alkali-labile sites) at a concentration that was cytotoxic to cells; no CA were reported following treatment during other cell cycle phases (Lima et al. 2008). In another study, MnCl2 (0.1–1 mM) treatment for between 30 and 60 min induced SSB in mitochondrial DNA isolated from the liver of rats (Jiao et al. 2008). Frequency of mitochondrial SSB was significantly increased above controls at 0.5 mM(30, 60, or 90 min incubation) and at 1 mMMn following a 60-min incubation (Jiao et al. 2008). An NTP (1993) study on MnSO4 reported a significant increase in the frequency of CA at 176–300μg/ml without S9, and sister chromatid exchanges (SCE) at 5–50μg/ml with or without S9, in Chinese hamster ovary (CHO) cells. The incidence of CA occurred within a narrow concentration range and chemical-induced cell cycle delay was observed at the two highest concentrations in the absence of S9 (NTP 1993). Galloway et al. (1987) reported a similar pattern in CHO-W-B1 cells; positive SCE responses were observed at 5–50μg/ml MnSO4in the absence of S9, and above 300μg/ml in the presence of S9, concentrations where marked cell cycle delay occurred. A quantitative increase in CA was also noted above 200μg/ml (MnSO4 range tested: 140– 300μg/ml) in the absence of S9, although a negative
response was seen in the presence of S9. De Meo et al. (1991) also reported that MnCl2 (1.5–4.5 mmol) and acidified KMnO4(concentration not reported) induced significant DNA damage at all concentrations without any evidence of cytotoxicity (based on cell survival; cell viability>95%) in human peripheral lymphocytes. MnO2 treatment was associated with an increased frequency of DNA strand breaks in human polymorphonuclear leukocytes and CA and micronuclei (MN) in human peripheral lymphocytes (Dutta et al. 2006), although the study was poorly reported, only one concentration of MnO2 (1.2 mM; 0.76 mM Mn) was tested, and cytotoxicity was not reported. KMnO4 induced only a numerical increase in CA at concentrations above 1 mM (pH not reported) in a FM3A mouse mammary carcinoma cell line, while MnCl2 at up to 1 mM was negative (Umeda and Nishimura 1979). Cytotoxicity was found to be too high above this concentration of MnCl2 to allow accurate assessment and no data relating to these higher exposures were therefore presented in the paper (Umeda and Nishimura 1979). These findings suggest that the response to Mn exposure may depend on cell type and experimental conditions. A number of other studies were identified that were conducted on environmental mixtures known to contain Mn ( Bakare et al. 2007; Evseeva et al. 2003; Feng et al. 2007; Lingard et al. 2005), but these are considered of limited value in assessing the mutagenicity of Mn per se.
In Vivo Animal Studies
A limited number of non-GLP studies investigated the genotoxicity of Mn compounds in insects and rodents. An OECD guideline study for sex-linked recessive lethal mutations in germ cells in fruit fly Drosophila melanogaster treated with MnSO4 at 1000 ppm (injection) or 12,500 ppm (feeding) was negative (NTP 1993). However, while MnCl2 was also reported to be negative for somatic cell mutation in larvae exposed to a 14 mM solution (Rasmuson 1985), in a wing spot test in this species, oral MnCl2 exposure of larvae
trans-heterozygous for the wing-hair mutationsmhw and flr during the third instar stage was effective in inducing spots with one or two mutant hairs (small spots; cited in Gerber et al. 2002). Conflicting results were noted in two studies in rodents. Dikshith and Chandra (1978) reported no changes in the frequency of CA in bone marrow cells and spermatogonia of male ITRC albino rats (10 per group) given MnCl2 (50μg/kg/d) orally for 180 d compared to control animals; only data from 7 animals (4 exposed, 3 control) were presented. In another study, Joardar and Sharma (1990) found a concentration-related increase in the number of DNA-strand breaks/cell, CAs, sperm-head abnormalities, and micronucleated polychromatic erythrocytes (PCE) in Swiss albino mice given daily oral doses of MnSO4 (36.9, 72.9, or 219.6 mg Mn/kg body weight [bw]; 10.25, 20.25, and 61 mg MnSO4/100 g bw) or KMnO4 (22.8, 45.5, and 133 mg Mn/kg bw; 6.5, 13, and 38 mg KMnO4/100 g bw) for up to 3 wk. Joardar and Sharma (1990) concluded that MnSO4 was more clastogenic than KMnO4 although the effects were only apparent at high concentrations and may have been secondary to cytotoxicity. In considering these rodent studies, it should be noted that they lacked a positive control and the methodology and results were not well reported. In a study on wild rodents (wood mice Clethrionomys glareolus and bank voles Apodemus flavicollis), animals collected from a polluted area were found to have higher levels of Mn in the liver and kidneys and a higher frequency of MN in bone marrow cells (BMC) and peripheral blood than animals from the control site (Ieradi et al. 2003), although the presence of other heavy metals and possibly organic compounds in the soil at the polluted location and in the exposed animals’ body tissues precludes ascribing the changes seen to Mn exposure.
Evidence of Genotoxicity and Mutagenicity
This review aimed to critically evaluate the available literature on the carcinogenic
and genotoxic potential of inorganic forms of Mn and draw conclusions using a weight-of- evidence approach, where possible. A number of studies indicate that under in vitro conditions, inorganic Mn (presumably Mn2+) is weakly mutagenic, particularly to S. typhimurium strain TA102, but there remains a lack of clarity as to the nature of the underlying mechanism(s) of mutagenicity or whether there is an association with the expression of cytotoxic cellular responses. Given that the TA102 strain detects oxidizing mutagens, Mn2+ may potentially act as a mutagen by generating ROS. Occasional positive results have been noted with a number of other strains ofS. typhimurium,but in these cases the outcome appears to be highly dependent upon test conditions. Some other metals (Fe,
Ni, and vanadium [V], among others) generate ROS through redox processes such as the Fenton reaction, leading to the generation of superoxide radicals and hydroxyl radicals; the ROS generated could potentially interact with macromolecules such as DNA to induce oxidative damage (Valko et al. 2005). Although direct evidence for such a mechanism is currently lacking in the case of Mn, indirect evidence comes from in vitro studies which reported cytokine release and induction of cell signaling pathways in cells exposed to elemental Mn (Pascal and Tessier 2004; Tessier and Pascal 2006) and increased GSH levels in rats treated with MnCl2 (Jiao et al. 2008). Other studies published in the early 1980s suggest that divalent Mn may exert its weak mutagenic activity by interacting directly with DNA and/or with DNA polymerases (Beckman et al. 1985; Bhanot and Solomon 1994; El- Deiry et al. 1984; Zakour et al. 1981), while the recent study by Jiao et al. (2008) also provides evidence of interactions with DNA, including at the mitochondrial level. While the mechanistic studies summarized here are considered informative, they are insufficient to fully elucidate the relative importance of possible ROS generation or interactions with DNA polymerases as potential mechanisms of Mn mutagenicity.
There is no convincing evidence that Mn is mutagenic or clastogenic in vivo, and the available data from studies on fruit flies and rodents are predominantly negative. However, there is conflicting evidence of Mn clastogenicity, although it should be noted that the available published literature is of limited quality. Chromosomal abnormalities were noted in a study in mice (Joardar and Sharma 1990), although another study that addressed both somatic and germ-cell endpoints in rats was negative (Dikshith and Chandra 1978). Overall, the weight of evidence from the available experimental studies suggests that Mn is probably clastogenic in vitro and may also be an in vivo clastogen. While a number of possible mechanisms have been suggested, the evidence is considered insufficient to establish their validity or relative significance.
The development of cancer diseases involves a variety of successive steps of which thefirst one, the malignant transformation of a cell,—but probably not the following ones, promotion and progression of the tumor—is related to changes in the genome. In order to rapidly screen a variety of agents for a possible carcinogenic action and, thereby, to avoid lengthy and expensive animal experiments, more than 100 short-term assays have been developed to evaluate the mutagenic/carcinogenic potential of chemicals. These tests observe several different types of changes in the genetic material such as the sequence or integrity of nuclear DNA, the production of gene mutations (forward and reverse), cyto-
genetic changes of the structure of the chromosome etc. Such tests can be performed in vitro on prokaryotic or eukaryotic cells or in vivo on different organisms (plants, insects, mammals, etc) but they would, obviously, fail for agents that cause cancer disease by promoting an already transformed cells or by modify- ing the progression of a tumor. Moreover, it must be recognized that the biokinetics and metabolism as well as individual susceptibility of a cell in a given tissue and the likelihood that it can be promoted and may pro- gress will greatly influence the risk of cancer from an agent.
Potassium permanganate (KMnO4)can cause damage to the integrity of the DNA chain as shown by the result of the single- cell gel assay (SCGA, or comet test) in human peripheral blood lymphocytes [47]. Fidelity of DNA replication decreases substantially in the presence of Mn2+by way of modifying the activity of DNA polymerase [48,49]. Mn2+does, however, not seem to interfere with the repair of chemically induced DNA damage [50]. Mn2+and, to a lesser extent, Mn7+, can induce a pleiotropic cellular response called SOS repair when the normal progression of the replication fork is impeded [51]. Divalent manganese may also mediate in vitro mispairing of ethylating agents or aliphatic epoxides [52].
Manganese sulfate (MnSO4) induces mutations in T4 phage growing on Escherichia coli [53,54] or enhance UV mutagenesis in Escherichia coli [55]; KMnO4 is less effective than MnSO4in these respects.
Three principal assays are used to assess the production of mutations by manganese in bacteria: the Rec-assay in Bacillus subtilis, the reversion assay in Salmonella typhimurium (Ames test) and the formation of mutations in the lac I gene of Escherichia coli. MnCl2, Mn(NO3)2, MnSO4, Mn(CH3COO)2 at concentrations of 0.05 M gave slightly positive results in the Rec-assay in the Bacillus subtilis strains H17 (Rec+, arg−, and trp−) and M45 ( Rec−, arg−and trp−) [56]. Positive results in the Rec-assay usually indicate a covalent binding to DNA or a chemical breakage of DNA, but the mode of action is obscure and perhaps unrelated to genetic damage. The conventional Ames test does not appear very suitable for detecting the genotoxicity of metal salts [57]. It is, therefore, not surprising that studies performed to assess the ability of manganese compounds such as MnSO4 and KMnO4 yielded negative results in this assay [58,59]. Manganese chloride was the only compound to give positive results with the TA102 strain but, conversely, appears to display a dose-dependent anti-mutagenic activity towards aflatoxin B1 [60] and quercetin [61]. However, a micro- technique adapted from the classical Ames test suggests that MnSO4 and Mn Cl2 might behave as directly-acting mutagens requiring no metabolic activation to in- duce reverse mutations [47]. Mn2+ is also mutagenic in
the lac I gene of Eschericchia coli but the mutations observed involve changes that do not yield nonsense mutations [62].
Manganese acts as an error-producing factor during mit-DNA replication in Saccharomyces cerevisiae. This fact is in agreement with thefinding that Mn2+ induces efficiently erythromycine-resistant mitochondrial mutations [63–65], and that it cannot induce such mutations in the presence of hydoxyurea which inhibits nuclear and mitochondrial DNA synthesis [66].
Manganese chloride given orally to larvae trans-heterozygous for the wing-hair mutations mwh andflr during the third instar stage was clearly effective in inducing spots with one or two mutant hairs (small spots) in the wing spot test of Drosophila melanogaster [67]. The ability of MnCl2to induce forward mutations was confirmed in vitro in eukaryotic cells in Chinese hamster V79 mammalian cells (mutants resistant to 8-azaguanine), in CHO-K1-BH4 cells and in L5178Y mouse lymphoma cells (mutants resistant to 6-thiogua- nine) [68,69]. The in vitro transformation ability by the simian adenovirus SA7 in Syrian hamster embryo cells was enhanced by MnCl2 [70], but the relevance of this test for an evaluation of the mutagenic potential of chemicals is questionable.
The carcinogenic potential of chemicals has often been considered as associated to their ability to cause cytogenetic changes. In this respect, it is of interest to note that Vicia faba roots cultured in the presence of Mn(NO3)2 display aberrations in mitotic cells [71,72], and that MnCl2 also causes disturbances in spindle formation in Allium cepa [73]. Regarding mammalian cells in vitro, Mn2+ and to a lesser extent KMnO4 induce chromosome damage in humanfibroblasts, Chinese hamster ovary cells and FM3A cells isolated from a C3H mouse mammary carcinoma [74,75]. Yet, no strand breaks or cross links were observed by McLean et al [76] in human leucocytes treated with Mn2+. The clastogenic activity of manganese salts has been con-firmed in vivo in mice given orally daily doses of KMnO4 but not for MnSO4. [77]. Sheep fed 0.75 or 1.5 g daily for one year of a metal mixture from an aluminium processing plants containing in addition to 0.063% Mn also Al, As, Cd displayed an increase in SCE in cultured lymphocytes [78]. In view of the complex composition of this material, the positive results can hardly be attributed to manganese. It should be added that MnDPDP was reported not to be genotoxic in a battery of several different tests [36].
In summary, some manganese compounds in the order MnCl2\KMnO4,\MnSO4 gave positive results in several short-term tests which might be related to the small carcinogenic activity shown in some long-term studies. It should be pointed out that even where manganese gave positive responses, they were always lower than those from other metals such as nickel and
chromium, metals with pronounced carcinogenic activity.
Endpoint conclusion
- Endpoint conclusion:
- no adverse effect observed (negative)
Additional information
Justification for classification or non-classification
Information on Registered Substances comes from registration dossiers which have been assigned a registration number. The assignment of a registration number does however not guarantee that the information in the dossier is correct or that the dossier is compliant with Regulation (EC) No 1907/2006 (the REACH Regulation). This information has not been reviewed or verified by the Agency or any other authority. The content is subject to change without prior notice.
Reproduction or further distribution of this information may be subject to copyright protection. Use of the information without obtaining the permission from the owner(s) of the respective information might violate the rights of the owner.
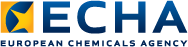