Registration Dossier
Registration Dossier
Data platform availability banner - registered substances factsheets
Please be aware that this old REACH registration data factsheet is no longer maintained; it remains frozen as of 19th May 2023.
The new ECHA CHEM database has been released by ECHA, and it now contains all REACH registration data. There are more details on the transition of ECHA's published data to ECHA CHEM here.
Diss Factsheets
Use of this information is subject to copyright laws and may require the permission of the owner of the information, as described in the ECHA Legal Notice.
EC number: 240-841-2 | CAS number: 16812-54-7
- Life Cycle description
- Uses advised against
- Endpoint summary
- Appearance / physical state / colour
- Melting point / freezing point
- Boiling point
- Density
- Particle size distribution (Granulometry)
- Vapour pressure
- Partition coefficient
- Water solubility
- Solubility in organic solvents / fat solubility
- Surface tension
- Flash point
- Auto flammability
- Flammability
- Explosiveness
- Oxidising properties
- Oxidation reduction potential
- Stability in organic solvents and identity of relevant degradation products
- Storage stability and reactivity towards container material
- Stability: thermal, sunlight, metals
- pH
- Dissociation constant
- Viscosity
- Additional physico-chemical information
- Additional physico-chemical properties of nanomaterials
- Nanomaterial agglomeration / aggregation
- Nanomaterial crystalline phase
- Nanomaterial crystallite and grain size
- Nanomaterial aspect ratio / shape
- Nanomaterial specific surface area
- Nanomaterial Zeta potential
- Nanomaterial surface chemistry
- Nanomaterial dustiness
- Nanomaterial porosity
- Nanomaterial pour density
- Nanomaterial photocatalytic activity
- Nanomaterial radical formation potential
- Nanomaterial catalytic activity
- Endpoint summary
- Stability
- Biodegradation
- Bioaccumulation
- Transport and distribution
- Environmental data
- Additional information on environmental fate and behaviour
- Ecotoxicological Summary
- Aquatic toxicity
- Endpoint summary
- Short-term toxicity to fish
- Long-term toxicity to fish
- Short-term toxicity to aquatic invertebrates
- Long-term toxicity to aquatic invertebrates
- Toxicity to aquatic algae and cyanobacteria
- Toxicity to aquatic plants other than algae
- Toxicity to microorganisms
- Endocrine disrupter testing in aquatic vertebrates – in vivo
- Toxicity to other aquatic organisms
- Sediment toxicity
- Terrestrial toxicity
- Biological effects monitoring
- Biotransformation and kinetics
- Additional ecotoxological information
- Toxicological Summary
- Toxicokinetics, metabolism and distribution
- Acute Toxicity
- Irritation / corrosion
- Sensitisation
- Repeated dose toxicity
- Genetic toxicity
- Carcinogenicity
- Toxicity to reproduction
- Specific investigations
- Exposure related observations in humans
- Toxic effects on livestock and pets
- Additional toxicological data

Endpoint summary
Administrative data
Key value for chemical safety assessment
Additional information
Manyin vitrogenetic toxicity studies, were identified characterizing the genetic toxicity of NiS. The primary cell types used in the studies included, Chinese Hamster Ovary (CHO) or Syrian hamster embryo (SHE) cells, though some assays also utilized mouse FM3A or C3H10T1/2 cells. Genotoxicity endpoints included chromosomal aberrations, morphological transformation, DNA strand breakage, and mutation frequency. Several studies evaluated cytotoxicity and/or cellular uptake of NiS along with genotoxicity. Most studies clarified the type of NiS (i. e., crystalline, amorphous) assessed in the study and several evaluated the comparative genotoxicity of multiple types.
The majority of studies identified reported findings of genotoxicity in CHO cells. Patierno and Costa (1985) reported that treatment of CHO cells with crystalline NiS induced single strand DNA breaks and DNA-protein crosslinks, but double stranded DNA breaks were not detected. Similar findings were reported by Sen and Costa (1985); in this assessment, crystalline NiS particles induced all four types of chromosome aberrations (gaps, breaks, exchanges, and dicentrics) at all doses and during all time points, and appeared to show a time-dependent, dose-response relationship. NiS also caused selective fragmentation of the heterochromatic long arms of the X-chromosomes. Sen and Costa (1986) further studied the genotoxicity of crystalline NiS and reported that exposure had minimal effect on cell cycle kinetics but caused a time-dependent (not dose-dependent) induction of sister chromatid exchanges. This group of investigators also demonstrated that NiS induced a significant, time-dependent number of chromosomal aberrations in heterochromatic regions in both CHO and C3H10T1/2 cells (Senet al. 1987).
Several studies compared genotoxicity of crystalline and amorphous NiS in CHO or SHE cells. Costaet al. (1982) reported that the reduced form of both crystalline or amorphous NiS (which allowed for an increased uptake into cells relative to the non-reduced form) increased morphological transformation. This study also demonstrated that treatment of CHO cells with crystalline NiS resulted in increased DNA strand breakage relative to untreated cells and cells exposed to amorphous NiS. The authors concluded that surface charge differences between the two forms affects phagocytosis into the cell and the ability to transform cells was correlated with the ability of cells to phagocytize NiS. The importance of the role of uptake of NiS was supported by data demonstrating a dose-dependent decrease in the number of surviving colonies treated with NiS or Ni3S2; however, transformed colonies were only observed with Ni3S2.
In a separate evaluation, Robisonet al. (1982) also reported DNA breakage following exposure to crystalline NiS but not amorphous NiS; DNA breakage did not initially slow the growth of CHO cells, but evidence that DNA breakage slowed cell proliferation was evident at 48 hours. The authors concluded that Ni2+ions liberated from phagocytized NiS particles were more accessible to the nucleus (relative to NiCl2) to cause DNA damage. The relative importance of uptake was also reported in an assay using modified CHO cells (AS52 cells). A 24-hr exposure to NiS resulted in a significant dose-response correlation coefficient calculated for “amorphous NiS” based on the mutation frequencies for each dose (Fletcheret al. 1994). In comparing the administered doses to nickel levels in the cytosol and nucleus, the authors concluded that intracellular nickel (II) concentrations constituted an important determinant in cytotoxicity and mutagenicity responses by AS52 CHO cells to nickel compounds.
In FM3A cells from a mouse mammary carcinoma, NiS induced a dose-dependent (0.32 to 2 mM) increase in chromosomal aberrations – including gaps, breaks, and exchanges but no fragmentations (Umeda and Nishimura 1979; Nishimura and Umeda 1979). This group of authors also reported that the percent of aberrant metaphases decreased as recovery time increased.
Dose-dependent increases in cytotoxicity and mutation frequency were reported in Chinese hamster V79 cells and two V79 hprt deficient transgenic variants (G12 and G10) with the bacterial gpt gene integrated at different chromosome sites (Kargacinet al. 1993). Because mutation frequency varied between the cell types; the authors speculated that mechanisms of mutagenicity might differ across the transgenic cell lines. Positive results in G12 cells were later found to be due to epigenetic effects of nickel on DNA methylation rather than true mutagenic effects. Leeet al. (1993) reported that NiS caused dose-dependent (0.08 to 0.6 μg Ni/cm2) increases in cytotoxicity and reported a mutation frequency as high as 55-fold greater than the spontaneous mutation rate in G12 cells exposed to NiS for 24 hr.
The study published by Arrouijalet al. (1990) demonstrated positivein vitroclastogenic activity of α-Ni3S2in human lymphocytes. Statistically significant, dose-dependent increases in aberrations (generally ≥ 20 per 200 cells) were observed. Mutagenic effects were also assessed by Ames test in five strains; high toxicity (growth inhibition) was observed at concentrations of ≥ 500 μg/plate, though no mutagenic activity was observed in any of the strains. Mutagenic effects were also negative at the HPRT locus in V79 cells under the conditions tested. The authors of this study concluded that the soluble fraction was responsible for genetic toxicities observed.
As noin vivostudies with NiS were located, the study conducted by Arrouijalet al. (1990) can be used to read-across from Ni3S2. Following a single i. p. injection (a non physiological route of administration for a water insoluble nickel compound like nickel subsulphide), mice exhibited a significant increase of micronuclei frequency in polychromatic erythrocytes (PCEs) and a significant decrease in the number PCEs. Together within vitrofindings, the authors concluded that Ni3S2when injected as a suspension can causein vivoclastogenic effects.
Mayeret al.(1998) also conducted a multi-component study to evaluate the mutagenicity and genotoxicity of Ni3S2bothin vitroandin vivo. Results indicated that Ni3S2administration increased mutation frequency up to 4.5-fold duringin vitromutation studies using a BigBlueTM Rat 2 embryo fibroblast cell line (lac1 transgenic line). Assays were also conductedin vivoin transgenic rodent mutation models to investigate the DNA damaging effect and mutagenic potential of nickel subsulphide in target cells of carcinogenesis. CD2F1 mice and lacZ transgenic CD2F1 mice (MutaMouse mice), as well as F344 rats and lac1 transgenic F344 rats (Big Blue rats), exposed to Ni3S2via inhalation did not exhibit the same type of responses observedin vitro, and treatment-related genotoxic effects were generally limited to DNA damage in mice; no mutagenicity was observed. Thus, the authors suggested that the collective findings were supportive of a non-genotoxic model for nickel carcinogenesis. A repeated dose inhalation study with nickel subsulphide in rats can be read across to nickel sulphide (Bensonet al., 2002). This study found that inhalation exposuresto nickel sulphate and nickel subsulphide at toxic levels can cause genotoxicity in the respiratory tractin vivo.However, only Ni3S2exposure (0.6 mg/m3, 0.44 mg Ni/m3) significantly increased epithelial and nonepithelial cell proliferation after 3 and 13 weeks.
Collectively, NiS – both crystalline and amorphous - have demonstrated the potential to cause genotoxicity, including DNA damage, under laboratory conditions in a number of cell lines. However, in a number of these studies, negative and positive controls were not incorporated, nor were statistical analyses implemented to determine the significance of findings. Taken together with the results ofin vivostudies conducted with another sulfidic Ni compound, Ni3S2, the weight of the evidence evaluation indicates that a genotoxic potential should be considered for NiS.
The following information is taken into account for any hazard / risk assessment:
Collectively, NiS – both crystalline and amorphous - have demonstrated the potential to cause genotoxicity, including DNA damage, under laboratory conditions in a number of cell lines. However, in a number of these studies, negative and positive controls were not incorporated, nor were statistical analyses implemented to determine the significance of findings. Taken together with the results ofin vivostudies conducted with another sulfidic Ni compound, Ni3S2, the weight of the evidence evaluation indicates that the genotoxic potential of NiS should be considered. Recently, nickel compounds have been recognized as genotoxic carcinogens with threshold mode of action in ECHA RAC opinion on nickel and nickel compounds OELs (see discussion of ECHA 2018 report inAppendix C2).
Justification for classification or non-classification
Ni sulfide is classified as Muta. 2;H341 according to the 1st ATP to the CLP Regulation. Background information can be found in the discussion section.
Information on Registered Substances comes from registration dossiers which have been assigned a registration number. The assignment of a registration number does however not guarantee that the information in the dossier is correct or that the dossier is compliant with Regulation (EC) No 1907/2006 (the REACH Regulation). This information has not been reviewed or verified by the Agency or any other authority. The content is subject to change without prior notice.
Reproduction or further distribution of this information may be subject to copyright protection. Use of the information without obtaining the permission from the owner(s) of the respective information might violate the rights of the owner.
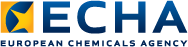