Registration Dossier
Registration Dossier
Data platform availability banner - registered substances factsheets
Please be aware that this old REACH registration data factsheet is no longer maintained; it remains frozen as of 19th May 2023.
The new ECHA CHEM database has been released by ECHA, and it now contains all REACH registration data. There are more details on the transition of ECHA's published data to ECHA CHEM here.
Diss Factsheets
Use of this information is subject to copyright laws and may require the permission of the owner of the information, as described in the ECHA Legal Notice.
EC number: 611-025-7 | CAS number: 53651-69-7
- Life Cycle description
- Uses advised against
- Endpoint summary
- Appearance / physical state / colour
- Melting point / freezing point
- Boiling point
- Density
- Particle size distribution (Granulometry)
- Vapour pressure
- Partition coefficient
- Water solubility
- Solubility in organic solvents / fat solubility
- Surface tension
- Flash point
- Auto flammability
- Flammability
- Explosiveness
- Oxidising properties
- Oxidation reduction potential
- Stability in organic solvents and identity of relevant degradation products
- Storage stability and reactivity towards container material
- Stability: thermal, sunlight, metals
- pH
- Dissociation constant
- Viscosity
- Additional physico-chemical information
- Additional physico-chemical properties of nanomaterials
- Nanomaterial agglomeration / aggregation
- Nanomaterial crystalline phase
- Nanomaterial crystallite and grain size
- Nanomaterial aspect ratio / shape
- Nanomaterial specific surface area
- Nanomaterial Zeta potential
- Nanomaterial surface chemistry
- Nanomaterial dustiness
- Nanomaterial porosity
- Nanomaterial pour density
- Nanomaterial photocatalytic activity
- Nanomaterial radical formation potential
- Nanomaterial catalytic activity
- Endpoint summary
- Stability
- Biodegradation
- Bioaccumulation
- Transport and distribution
- Environmental data
- Additional information on environmental fate and behaviour
- Ecotoxicological Summary
- Aquatic toxicity
- Endpoint summary
- Short-term toxicity to fish
- Long-term toxicity to fish
- Short-term toxicity to aquatic invertebrates
- Long-term toxicity to aquatic invertebrates
- Toxicity to aquatic algae and cyanobacteria
- Toxicity to aquatic plants other than algae
- Toxicity to microorganisms
- Endocrine disrupter testing in aquatic vertebrates – in vivo
- Toxicity to other aquatic organisms
- Sediment toxicity
- Terrestrial toxicity
- Biological effects monitoring
- Biotransformation and kinetics
- Additional ecotoxological information
- Toxicological Summary
- Toxicokinetics, metabolism and distribution
- Acute Toxicity
- Irritation / corrosion
- Sensitisation
- Repeated dose toxicity
- Genetic toxicity
- Carcinogenicity
- Toxicity to reproduction
- Specific investigations
- Exposure related observations in humans
- Toxic effects on livestock and pets
- Additional toxicological data

Endpoint summary
Administrative data
Link to relevant study record(s)
- Endpoint:
- basic toxicokinetics in vitro / ex vivo
- Type of information:
- experimental study
- Adequacy of study:
- key study
- Study period:
- 1998-11-16 to 1999-01-26
- Reliability:
- 1 (reliable without restriction)
- Rationale for reliability incl. deficiencies:
- test procedure in accordance with generally accepted scientific standards and described in sufficient detail
- Objective of study:
- metabolism
- Qualifier:
- no guideline followed
- Principles of method if other than guideline:
- S9 homogenates of liver, blood, skin, small intestinal mucosa and nasal olfactory epithelium, prepared from healthy male Wistar rats, were used. The rates of hydrolysis of the lactate esters by these homogenates were determined, and the enzyme kinetic parameters Km and Vmax were established, where possible. The S9 homogenates used were similar to those used in a previous in vitro hydrolysis study with L-lactic acid esters (TNO-report V97.552). The esterase acitivities of the various homogenates towards the model substrate p-nitrophenyl-butyrate, were determined according to the method described by Bogdanffy et al. (1987): 100 µM p-nitrophenyl-butyrate and a suitable amount of homogenate were incubated in 0.1 M phosphate buffer pH 7.8 at 25°C in a total volume of 1 ml. The rate of hydrolysis was measured spectrophotometrically at 400 nm.
- GLP compliance:
- yes (incl. QA statement)
- Specific details on test material used for the study:
- - Name of test material used in the report: n-Propyl-(L)-lactate
- Batch no.: MJE-8
- Purity: >99%
- Storage: ambient temperature - Radiolabelling:
- no
- Species:
- other: incubation with homogenate of rat blood, skin, liver, small intestinal mucosa and nasal olfactory epithelium.
- Strain:
- Wistar
- Sex:
- male
- Route of administration:
- other: incubation with homogenate of rat blood, skin, liver, small intestinal mucosa and nasal olfactory epithelium
- Vehicle:
- unchanged (no vehicle)
- Details on exposure:
- General incubation conditions:
The lactate esters were incubated at 37°C in 1 ml incubation mixtures containing 0.10 M potassium phosphate buffer pH 7.4. The chemical hydrolysis was determined in incubation experiments without homogenates. Blanks were homogenates without test substances. Incubations were performed in open tubes. The reaction was terminated by addition of 3 mL of ice-cold ethanol. After placing the samples in the freezer for at least 20 minutes, the tubes were centrifuged for 10 min at 4300 x g (room temperature) and decanted into new tubes. The samples were evaporated to dryness with nitrogen and stored at < -15°C until analysis.
Times and concentrations:
The lactate esters were incubated with the various homogenates for 5, 10, 20, 40 and 120 minutes. Chemical hydrolysis was measured by incubating the substrates without homogenates during 120 min. The lactate ester concentrations used were 50, 100, 250, 500 and 1250 µM.
Analysis:
The liberated amount of L-lactic acid was determined by using the Boehringer test for the determination of L-lactic acid in foodstuffs. The liberated amount of D-lactic acid was determined by using the Boehringer test for the determination of D- and L-lactic acid in foodstuffs and other materials. Instead of the glycylglycine buffer (pH 10) included in the kit, a 0.1 M potassium phosphate buffer pH 7.4 (assay buffer) was used, in order to minimize hydrolysis of the lactate esters during the measurement of the liberated amounts of L- and D-lactic acid. - Duration and frequency of treatment / exposure:
- Single application for 5, 10, 20, 40 and 120 minutes.
- Dose / conc.:
- 1 250 other: µM
- Dose / conc.:
- 500 other: µM
- Dose / conc.:
- 250 other: µM
- Dose / conc.:
- 100 other: µM
- Dose / conc.:
- 50 other: µM
- No. of animals per sex per dose / concentration:
- The amounts of tissue protein used in the incubation experiments, are: 2.38 µg of nasal olfactory epithelium protein, 192.4 µg of small intestinal mucosal protein, 7.43 µg of liver protein, 50.4 µg of skin protein and 832 µg of blood protein.
- Control animals:
- other: Chemical hydrolysis was determined in incubation experiments without homogenates. Blanks were homogenates without test substances.
- Positive control reference chemical:
- The esterase acitivities of the various homogenates towards the model substrate p-nitrophenylbutyrate were determined.
- Details on dosing and sampling:
- Analysis:
determination of L-lactic acid
The liberated amount of L-lactic acid was determined by using the Boehringer test for the determination of L-lactic acid in foodstuffs and other materials. The liberated amount of D-lactic acid was determined by using the Boehringher test for the determination of D- and L-lactic acid in foodstuffs and other materials. Instead of the glycylglycine buffer (pH 10), included in the kit, a 0.1 M potassium phosphate buffer pH 7.4 (assay buffer) was used, in order to minimize hydrolysis of the lactate esters during the measurement of the liberated amount of L- and D-lactic acid.
The detection limit of the method was arbitrarily fixed at 10 nmol (absorption ~ 0.02). - Statistics:
- Calculations:
The amounts L-lactic acid or D-lactic acid formed during the incubations were calculated from the respective standard curves.
The rates of hydrolysis were corrected for the chemical hydrolysis, which was assumed to be a linear chemical proces.
The initital rates of hydrolysis were calculated from the amounts of liberated L-lactic acid/D-lactic acid (corrected for chemical hydrolysis) with the regression model:
liberated lactic acid (nmol) = a . (time) + b . (time)²,
or with the regression model
liberated lactic acid (nmol) = a.(time),
with a = regression coefficient of the linear component and b = regression coefficient of the quadratic component.
The regression coefficient 'a' respresents the initital rate of hydrolysis expressed as nmol/min.
After calculating the initial rates of hydrolysis expressed as nmol/min/mg S9 protein, the enzyme kinetic parameters Km and Vmax were determined by the curve-fitting program "EZ-FIT" (version 4.13 for MS Windows). - Metabolites identified:
- yes
- Conclusions:
- In the present study, the hydrolysis of propyl (S)-lactate was studied by conducting incubation experiments with various rat tissue homogenates. It was found that the propyl (S)-lactate is hydrolysed very rapidely by the different tissue homgenates.
- Executive summary:
The rates of hydrolysis of propyl (S)-lactate to L-lactic acid by homogenates of liver, blood, skin, small intestinal mucosa and nasal olfactory epithelium, prepared from healthy mal Wistar rats, was studied. Enzym kinetic parameters Km and Vmax were established, where possible.
All homogenates showed esterase activity to propyl (S)-lactate. Nasal olfactory epithelium, liver and skin were, in this order, the most efficient tissues with repect to the hydrolysis of propyl (S)-lactate. Enzymatic hydrolysis of n-propyl-L-lactate in vivo would be much faster than chemical hydrolysis.
- Endpoint:
- basic toxicokinetics in vitro / ex vivo
- Type of information:
- read-across from supporting substance (structural analogue or surrogate)
- Adequacy of study:
- supporting study
- Justification for type of information:
- For details and justification of read-across please refer to the read-across report attached to IUCLID section 13.
- Reason / purpose for cross-reference:
- read-across source
- Preliminary studies:
- The protein content of the nasal epithelium homogenate was determined to be 15.3 mg per mL. The increase of absorbance towards p-nitro-phenylbutyrate of the nasal epithelium homogenate using 15.3 µg of protein was 0.191 ± 0.006 (n= 2), corresponding to an esterase activity of 0.71 ± 0.02 µmol per min per mg of epithelial protein. The rate of chemical hydrolysis was investigated with the three smaller lactate esters methyl, ethyl and isopropyl-S-lactate in 0.05 M phosphate buffer pH 7.0 at 37 °C. The lactate esters were incubated at a concentration of 500 µM for 5 and 20 minutes, and 1, 3 and 19.5 hours. The rate of chemical hydrolysis was very low. Liberated L-lactic acid was only detected for methyl lactate and after 19.5 hours (6.1 mol corresponding to 1.2 % of the total amount of ester). Metabolism of L-lactic acid (buffered) was not observed when L-lactic acid was incubated with nasal epithelium homogenate. The use of inhibitors to prevent enzymatic oxidation to pyruvate was therefore not necessary. The recovery of L-lactic acid, as added to the incubation mixture in the absence of esters, was 91-93%. Using a set of enzymatic incubations of ethyl-S-lactate without addition of buffer, the effect on the pH was measured as a function of incubation time. At the last time point (150 min), the amount of protons detected was approximately 20-fold less than the amount of lactic acid produced. An initial lag phase was observed in the detection of protons: an increase in proton concentration was only measured after 60 minutes of incubation. This lag phase cannot be completely explained by the difference between rate of appearance of lactic acid and protons (as a consequence of the buffer present in the nasal epithelium homogenate), since the slope of the two curves after this lag phase still differed considerably.
- Type:
- metabolism
- Results:
- The following kinetic parameters of the enzymatic hydrolysis of ethylhexyl-S-lactate by rat olfactory epithelium homogenate: KM: 0.17 mM; Vmax: 420 nmol/min/mg protein.
- Metabolites identified:
- yes
- Details on metabolites:
- L-lactic acid is formed by enzymatic hydrolysis of ethylhexyl-S-lactate by carboxylesterase present in rat nasal olfactory tissue.
- Conclusions:
- In an in vitro study to assess the hydrolysis of L-lactate esters by rat nasal olfactory epitheliun homogenate the following observations were made:
Lactic acid is formed by enzymatic hydrolysis of ethylhexyl-S-lactate by carboxylesterase present in rat nasal olfactory tissue. Kinetic parameters of the enzymatic hydrolysis of ethylhexyl-S-lactate by rat olfactory epithelium homogenate were: Km = 0.17 mM and Vmax = 420 nmol/min/mg protein. In general, the olfactory epithelium carboxylesterase showed increasing capacity (increasing Vmax) and affinity (decreasing Km) towards L-lactate esters with increasing molecular weight of the alkyl group. From a large discrepancy between the amount of lactic acid formed and the increase in proton concentration even in very poorly buffered systems it is suggested that a certain defense against acidification exists. - Executive summary:
The hydrolysis of ethylhexyl-S-lactate by rat nasal olfactory epithelium homogenate was investigated in vitro. The ester was incubated with male Wistar rat olfactory epithelium at pH 7.0 and 37 °C. The amount of liberated L-lactic acid (buffered) was quantified at a series of time points, from which the initial rate of hydrolysis was estimated. Using a concentration range of 0.05–0.8 mM, the enzyme kinetic parameters were calculated to be Km = 0.17 mM and Vmax = 420 nmol/min/mg protein. Based on these values, the calculated half-life of the enzymatic hydrolysis of ethylhexyl-S-lactate is 0.0004 min or 0.024 sec.
Seven other lactate esters were also tested. In general, the olfactory epithelium carboxylesterase showed increasing capacity (increasing Vmax) and affinity (decreasing Km) towards L-lactate esters with increasing molecular weight of the alkyl group.
Since the pKa value of lactic acid is 3.80, the formation of lactic acid will (in non-buffered systems) directly result in acidification of the solution. However, even in poorly buffered systems (non-buffered incubation mix) a large discrepancy between the amount of lactic acid formed and the increase in proton concentration is observed. This suggest that a certain defense against acidification exists, and that in vivo, only high doses and/or prolonged exposure will result in acidification of tissues.
- Endpoint:
- basic toxicokinetics in vitro / ex vivo
- Type of information:
- read-across from supporting substance (structural analogue or surrogate)
- Adequacy of study:
- supporting study
- Justification for type of information:
- For details and justification of read-across please refer to the read-across report attached to OUCLID section 13.
- Preliminary studies:
- The protein content of the nasal epithelium homogenate was determined to be 15.3 mg per mL. The increase of absorbance towards p-nitro-phenylbutyrate of the nasal epithelium homogenate using 15.3 µg of protein was 0.191 ± 0.006 (n = 2), corresponding to an esterase acitivity of 0.71 ± 0.02 µmol per min per mg of epithelial protein.
The rate of chemical hydrolysis was investigated with ethyl (S)-lactate in 0.05 M phosphate buffer pH 7.0 at 37 °C. Ethyl (S)-lactate was incubated in a concentration of 500 µM for 5 and 20 minutes, and 1, 3 and 19.5 hours. The rate of chemical hydrolysis was very low. Liberated L-lactic acid could not be detected. The additonal 2-hours incubations with concentrations of ethyl (S)-lactate of 0.8, 1.6 and 3.2 mM showed detectable chemical hydrolysis at the highest concentration tested only. The rate of chemical hydrolysis, measured as the rate of lactic acid formation, was 0.04 nmol/min at a concentration of 3.2 mM ethyl (S)-lactate.
Metabolism of L-lactic acid (buffered) was not observed when L-lactic acid was incubated with nasal epithelium homogenate. The use of inhibitors to prevent enzymatic oxidation to pyruvate was therefore not necessary.
The recovery of L-lactic acid, as added to the incubation mixture in the absence of esters, was 91–93 %.
Using a set of enzymatic incubations of ethyl (S)-lactate without addition of buffer, the effect on the pH was measured as a funtion of incubation time. At the last timepoint (150 min), the amount of protons detected was approximately 20-fold less than the amount of lactic acid produced. An initial lag phase was observed in the detection of protons: an increase in proton concentration was only measured after 60 minutes of incubation. This lag phase cannot be completely explained by the difference between rate of appearance of lactic acid and protons (as a consequence of the buffer present in the nasal epithelium homogenate), since the slope of the two curves after this lag phase still differed considerably. - Type:
- metabolism
- Results:
- The following kinetic parameters of the enzymatic hydrolysis of ethyl (S)-lactate by rat olfactory epithelium homogenate: KM: 1.1 mM; Vmax: 170 nmol/min/mg protein
- Metabolites identified:
- yes
- Details on metabolites:
- L-lactic acid is formed by enzymatic hydrolysis of ethyl (S)-lactate by carboxylesterase present in rat nasal olfactory tissue.
Kinetic parameters of the enzymatic hydrolysis of ethyl (S)-lactate by rat olfactory epithelium homogenate were: Km = 1.1 mM and Vmax = 170 nmol/min/mg protein. - Conclusions:
- In a in vitro study to assess the hydrolysis of L-lactate esters by rat nasal olfactory epitheliun homogenate the following observations were made:
Lactic acid is formed by enzymatic hydrolysis of ethyl (S)-lactate by carboxylesterase present in rat nasal olfactory tissue.
Kinetic parameters of the enzymatic hydrolysis of ethyl-S-lactate by rat olfactory epithelium homogenate were: Km = 1.1 mM and Vmax = 170 nmol/min/mg protein. In general, the olfactory epithelium carboxylesterase showed increasing capacity (increasing Vmax) and afinity (decreasing Km) towards L-lactate esters with increasing molecular weight of the alkyl group. From a large discrepancy between the amount of lactic acid formed and the increase in proton concentration even in very poorly buffered systems it is suggested that a certain defence against acidification exisits. - Executive summary:
The hydrolysis of ethyl (S)-lactate by rat nasal olfactory epithelium homogenate was investigated. The ester was incubated with rat (male, Wistar) olfactory epithelium at pH 7.0 and 37 °C. The amount of liberated L-lactic acid (buffered) was quantified at a series of time points, from which the initial rat of hydrolysis was estimated. Using a concentration range of 0.05–3.2 mM, the enzyme kinetic parametes were calculated to be Km = 1.1 mM and Vmax = 170 nmol/min/mg protein. The calculated half-life of the enzymatic hydrolysis is 0.045 min or 0.27 sec.
Seven other L-lactate esters were also tested. In general, the olfactory epithelium carboxylesterase showed increasing capacity (increasing Vmax) and afinity (decreasing Km) towards L-lactate esters with increasing molecular weight of the alkyl group.
Since the pKa value of lactic acid is 3.80, the formation of lactic acid will (in non-buffered systems) directly result in acidification of the solution. However, even in poorly buffered systems (non-buffered incubation mix) a large discrepancy between the amount of lactic acid formed an the increase in proton concentration is observed. This suggests that a certain defence against acidification exists, and that in vivo, only high doses and/or prolonged exposure will result in acidification of tissues.
This information is used in a read-across approach in the assessment of the target substance. For details and justification of read-across please refer to the read-across report attached to IUCLID section 13.
- Endpoint:
- basic toxicokinetics
- Type of information:
- read-across from supporting substance (structural analogue or surrogate)
- Adequacy of study:
- supporting study
- Justification for type of information:
- For details and justification of read-across please refer to the read-across report attached to IUCLID section 13.
- Reason / purpose for cross-reference:
- read-across source
- Conclusions:
- In the evaluation of the use of lactic acid as the active substance in biocidal products, the natural occurrence of lactic acid in human food and the human body, as well as the role of the compound in human metabolism and physiology should be taken into account. This means that, when the risk for its use in biocidal products is assessed, the natural exposure to lactic acid in food and via endogenous sources, as well as exposure via the use of lactic acid as a food additive should be considered.
In the present report it is concluded that lactic acid can no longer be considered as a “dead-end” waste product of human metabolism, but should instead be seen to play an important role in cellular, regional, and whole body metabolism. Lactic acid has been detected in blood, several other body fluids and tissues. Concentrations of lactic acid increase significantly during intense exercise. At rest, blood concentrations have been reported of 1-1.5 mMol/L (90.1-135.12 mg/L), which can increase up to 10 mMol/L (900.8 mg/L) during exercise.
External human exposure to lactic acid can occur via its natural presence in food, for example in fruit, vegetables, sour milk products, and fermented products such as sauerkraut, yogurt and beer. Based on the available information on concentrations of lactic acid in some of these products, an estimate of the daily consumption of lactic acid due to its natural presence in food was made using the ‘FAO/WHO standard European diet’. A (minimum) daily intake of 1.175 g/person/day was calculated using the available information.
Another source of external exposure is its use as food additive; as such it is authorized in Europe (E270) and the United States (generally recognized as safe = GRAS). A daily intake of 1.65-2.76 g/person/day was estimated using the “Per Capita times 10” method, based on the amount of lactic acid put onto the market (EU and USA) as a food additive by Purac.
Based on the high levels of lactic acid in the human body and in human food, and its use as food additive, the evaluation of the human health effects of lactic acid should first and for all be based on a comparison of this background exposure and the potential contribution of lactic acid in biocidal products to these levels. Therefore, a risk assessment should not be based on the comparison with effects of exposure, but on the comparison with the total daily intake of lactic acid via food, both naturally and as food additive, which was estimated to be 2.8 g/person/day. When the application of Purac’s products will not result in a systemic exposure that contributes substantially to the total systemic exposure, many of the standard human toxicological studies dealing with systemic effects are deemed superfluous. - Executive summary:
The natural occurrence of lactic acid in human food and the human body, as well as the role of the compound in human metabolism and physiology is of primary importance in the understanding of the metabolism and toxicology of lactic acid. This means that, in risk assessment, the natural exposure to lactic acid in food and via endogenous sources, as well as exposure via the use of lactic acid as a food additive should be considered.
In the present report it is concluded that lactic acid, in contrast to previously held belief, can no longer be considered as a “dead-end” waste product of human metabolism, but should instead be seen to play an important role in cellular, regional, and whole body metabolism. Lactic acid has been detected in blood, several other body fluids and tissues. Concentrations of lactic acid increase significantly during intense exercise. At rest, blood concentrations have been reported of 1-1.5 mMol/L (90.1-135.12 mg/L), which can increase up to 10 mMol/L (900.8 mg/L) during exercise.
External human exposure to lactic acid can occur via its natural presence in food, for example in fruit, vegetables, sour milk products, and fermented products such as sauerkraut, yogurt and beer. Based on the available information on concentrations of lactic acid in some of these products, an estimate of the daily consumption of lactic acid due to its natural presence in food was made using the ‘FAO/WHO standard European diet’. A (minimum) daily intake of 1.175 g/person/day was calculated using the available information.
Another source of external exposure is its use as food additive; as such it is authorized in Europe (E270) and the United States (generally recognized as safe = GRAS). A daily intake of 1.65-2.76 g/person/day was estimated using the “Per Capita times 10” method, based on the amount of lactic acid put onto the market (EU and USA) as a food additive by the registrant.
Based on the high levels of lactic acid in the human body and in human food, and its use as food additive, the evaluation of the human health effects of lactic acid should first and for all be based on a comparison of this background exposure and the potential contribution of lactic acid in biocidal products to these levels. Therefore, a risk assessment should not be based on the comparison with effects of exposure, but on the comparison with the total daily intake of lactic acid via food, both naturally and as food additive, which was estimated to be 2.8 g/person/day. When the application of Purac’s products will not result in a systemic exposure that contributes substantially to the total systemic exposure, many of the standard human toxicological studies dealing with systemic effects are deemed superfluous.
This information is used in a read-across approach in the assessment of the target substance. For details and justification of read-across please refer to the read-across report attached to IUCLID section 13.
Referenceopen allclose all
The protein concentrations, and the esterase activities towards the model substrate p-nitrophenylbutyrate of the various tissue homogenates are presented in Table 1. These results show that esterase activity was present in the various homogenates and thus could be used for the measurement of esterase activity towards the various lactate esters.
Table 1: Mean protein concentrations, and esterase activities towards p-nitrophenylbutyrate (mean ± sd) of the various rat tissue homogenates.
Homogenate |
Protein concentration homogenate (mg/ml) |
Esterase activity towards p-nitrophenylbutyrate |
|
|
|
Amount of protein used in assay (µg) |
Activity (µmol/min/mg protein) |
Nasal olfactory |
7.14 |
11.9 |
1.013 ± 0.028 |
Small intestinal mucosa |
9.62 |
2.41 |
7.836 ± 0.064 |
Liver |
22.3 |
11.2 |
0.852 ± 0.010 |
Skin |
2.52 |
63.0 |
0.113 ± 0.003 |
Blood |
41.6 |
104 |
0.0134 ± 0.0001 |
The amounts of lactic acid formed by chemical hydrolysis after 2 hour incubations are presented in Table 2. From the results it can be concluded that the rate of chemical hydrolysis of n-propyl-L-lactate at pH 7.4 and 37°C is very low.
Table 2: Chemical hydrolysis of n-propyl-L-lactate to L-lactic acid.
Lactate ester |
nmol of L-lactic acid formed at the various concentrations |
||||
50 µM |
100 µM |
250 µM |
500 µM |
1250 µM |
|
n-propyl-(L)-lactate |
< 10 |
< 10 |
< 10 |
< 10 |
15.3 |
The initial rates of hydrolysis obtained for the various incubation time periods are presented in Table 3. The hydrolyses of n-propyl-L-lactate was most efficiently catalyzed by nasal olfactory epithelium and liver homogenates. The lowest activities were found for blood.
Table 3. Initial rates of hydrolysis of n-propyl-L-lactate by various rat tissue homogenates to L-lactic acid. Enzyme activities are expressed as nmol/min/mg protein.
|
Concentration (µM) |
Initial rates of hydrolysis |
|||||
Nasal olfactory epithelium |
Small intestinal mucosa |
Liver |
Skin |
Blood |
|
||
n-propyl-(L)-lactate |
50 |
260 |
3.7 |
165 |
23.6 |
0.7 |
|
100 |
559 |
7.0 |
283 |
40.0 |
1.3 |
|
|
250 |
982 |
13.0 |
516 |
75.0 |
2.8 |
|
|
500 |
1128 |
21.4 |
674 |
104.3 |
4.9 |
|
|
1250 |
1216 |
56.9 |
795 |
127.8 |
8.9 |
|
The enzyme kinetic parameters Km and Vmax, presented in Table 4, were calculated from the initial rates of hydrolysis of n-propyl-L-lactate. The hydrolysis of n-propyl-L-lactate by the homogenates of nasal epithelium, liver and skin showed Km values in the same order of magnitude (range 150-278 µM), while blood and small intestinal mucosa showed a high Km value, or first order kinetics in the tested concentration range. With respect to the obtained Vmax values it is observed that n-propyl-L-lactate was most efficiently hydrolyzed by nasal olfactory epithelium and liver homogenate.
Table 4. Enzyme kinetic parameters (mean ± sd) of the hydrolysis of n-propyl-L-lactate. Vmax is expressed as nmol/min/mg protein.
Homogenate |
Km (µM) |
Vmax |
Nasal olfactory epithelium |
150 ± 37 |
1420 ± 109 |
Small intestinal mucosa |
first order¹ v = 0.0455xS |
|
Liver |
220 ± 17 |
949 ± 25 |
Skin |
278 ± 18 |
158 ± 4 |
Blood |
1450 ± 62 |
19.2 ± 0.5 |
* v = rate expressed as nmol/min/mg protein; S = ester concentration
In order to extrapolate the obtained kinetic parameters in terms of disappearance rates of n-propyl-L-lactate in the organs/tissues, the obtained kinetic parameters were scaled up to hydrolysis rates expressed per weight of tissue, by using the total amount of protein/gram of tissue. Subsequently, the disappearance in time of the compound in the organs/tissues was calculated by the Michaelis-Menten or first order equation and the data presented in Table 4. A starting concentration of 500 µM was used. However, it had to be assumed that the equilibrium of the reactions are completely on the side of the hydrolyzed compounds. The calculated disappearance rates would be higher in vivo. The times were calculated in which at least 99% of the ester would be hydrolyzed (Table 5). Table 5 shows that n-propyl-L-lactate were hydrolysed very rapidly by nasal olfactory epithelium and liver homogenate, while blood and small intestinal mucosa were much less efficient. Table 5 also shows that chemical hydrolysis, compared to the enzymatical hydrolysis by nasal olfactory epithelium and liver, is not detectable.
Table 5. Calculated times (seconds) in which at least 99% of n-propyl-L-lactate would be hydrolyzed. The starting concentration was 500 µM. The reactions were assumed to be completely oriented towards the hydrolyzed compounds.
Homogenate |
Time (s) |
Nasal olfactory epithelium |
0.9 |
Small intestinal mucosa |
136 |
Liver |
1.0 |
Skin |
31 |
Blood |
132 |
Chemical hydrolysis |
- |
- = no detectable rate of hydrolysis
Kinetic parameters of the enzymatic hydrolysis of ethylhexyl-S-lactate by rat olfactory epithelium homogenate were: Km = 0.17 mM and Vmax = 420 nmol/min/mg protein.
Kinetic parameters of the enzymatic hydrolysis of ethyl (S)-lactate by rat olfactory epithelium homogenate were: Km = 1.1 mM and Vmax = 170 nmol/min/mg protein. The calculated half-life of the enzymatic hydrolysis of ethyl (S)-lactate is 0.045 min or 0.27 sec.
The role of lactic acid in metabolism has kept researchers occupied for a long time. For many years, lactic acid was considered a dead-end waste product of the glycolysis, the conversion of glucose into pyruvate (producing a relatively small amount of ATP), in the absence of oxygen. Recently, the role of lactic acid in metabolism was reconsidered, and L-lactate is considered as a functional metabolite and mammalian fuel. It was observed that lactate can be transferred from its site of production (cytosol) to neighbouring cells and other organs, as well as intracellularly, where its oxidation or continued metabolism can occur. This "lactate shuttle" results in the distribution of lactic acid to other cells, where it is directly oxidised, re-converted back to pyruvate or glucose, allowing the process of glycolysis to restart and ATP provision maintained.
Description of key information
Propyl (S)-lactate, is rapidly hydrolysed (as all lactate esters) in vivo into lactic acid and propanol.
Key value for chemical safety assessment
- Bioaccumulation potential:
- no bioaccumulation potential
Additional information
The rates of hydrolysis of propyl (S)-lactate to L-lactic acid by homogenates of liver, blood, skin, small intestinal mucosa and nasal olfactory epithelium, prepared from healthy male Wistar rats, was studied ex vivo. Enzyme kinetic parameters Km and Vmax were established, where possible.
All tissue homogenates showed esterase activity to propyl (S)-lactate. Nasal olfactory epithelium, liver and skin were, in this order, the most efficient tissues with respect to the hydrolysis of propyl (S)-lactate. Enzymatic hydrolysis of propyl (S)-lactate in vivo would be much faster than chemical hydrolysis. Based on the results of the key study and also shown in similar studies conducted with further lactate esters it can be stated, that the investigated lactate esters are rapidly enzymatically hydrolsed in vivo into lactic acid and the corresponding alcohol.
Information on Registered Substances comes from registration dossiers which have been assigned a registration number. The assignment of a registration number does however not guarantee that the information in the dossier is correct or that the dossier is compliant with Regulation (EC) No 1907/2006 (the REACH Regulation). This information has not been reviewed or verified by the Agency or any other authority. The content is subject to change without prior notice.
Reproduction or further distribution of this information may be subject to copyright protection. Use of the information without obtaining the permission from the owner(s) of the respective information might violate the rights of the owner.
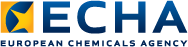