Registration Dossier
Registration Dossier
Data platform availability banner - registered substances factsheets
Please be aware that this old REACH registration data factsheet is no longer maintained; it remains frozen as of 19th May 2023.
The new ECHA CHEM database has been released by ECHA, and it now contains all REACH registration data. There are more details on the transition of ECHA's published data to ECHA CHEM here.
Diss Factsheets
Use of this information is subject to copyright laws and may require the permission of the owner of the information, as described in the ECHA Legal Notice.
EC number: 203-894-2 | CAS number: 111-67-1
- Life Cycle description
- Uses advised against
- Endpoint summary
- Appearance / physical state / colour
- Melting point / freezing point
- Boiling point
- Density
- Particle size distribution (Granulometry)
- Vapour pressure
- Partition coefficient
- Water solubility
- Solubility in organic solvents / fat solubility
- Surface tension
- Flash point
- Auto flammability
- Flammability
- Explosiveness
- Oxidising properties
- Oxidation reduction potential
- Stability in organic solvents and identity of relevant degradation products
- Storage stability and reactivity towards container material
- Stability: thermal, sunlight, metals
- pH
- Dissociation constant
- Viscosity
- Additional physico-chemical information
- Additional physico-chemical properties of nanomaterials
- Nanomaterial agglomeration / aggregation
- Nanomaterial crystalline phase
- Nanomaterial crystallite and grain size
- Nanomaterial aspect ratio / shape
- Nanomaterial specific surface area
- Nanomaterial Zeta potential
- Nanomaterial surface chemistry
- Nanomaterial dustiness
- Nanomaterial porosity
- Nanomaterial pour density
- Nanomaterial photocatalytic activity
- Nanomaterial radical formation potential
- Nanomaterial catalytic activity
- Endpoint summary
- Stability
- Biodegradation
- Bioaccumulation
- Transport and distribution
- Environmental data
- Additional information on environmental fate and behaviour
- Ecotoxicological Summary
- Aquatic toxicity
- Endpoint summary
- Short-term toxicity to fish
- Long-term toxicity to fish
- Short-term toxicity to aquatic invertebrates
- Long-term toxicity to aquatic invertebrates
- Toxicity to aquatic algae and cyanobacteria
- Toxicity to aquatic plants other than algae
- Toxicity to microorganisms
- Endocrine disrupter testing in aquatic vertebrates – in vivo
- Toxicity to other aquatic organisms
- Sediment toxicity
- Terrestrial toxicity
- Biological effects monitoring
- Biotransformation and kinetics
- Additional ecotoxological information
- Toxicological Summary
- Toxicokinetics, metabolism and distribution
- Acute Toxicity
- Irritation / corrosion
- Sensitisation
- Repeated dose toxicity
- Genetic toxicity
- Carcinogenicity
- Toxicity to reproduction
- Specific investigations
- Exposure related observations in humans
- Toxic effects on livestock and pets
- Additional toxicological data

Endpoint summary
Administrative data
Link to relevant study record(s)
- Endpoint:
- basic toxicokinetics in vivo
- Type of information:
- experimental study
- Adequacy of study:
- key study
- Study period:
- 1993
- Reliability:
- 2 (reliable with restrictions)
- Rationale for reliability incl. deficiencies:
- other: see 'Remark'
- Remarks:
- This study is classified as reliable with restrictions because while there is no statement regarding whether this study was conducted according to GLP or equivalent, the information provided indicates that this study was conducted in a similar manner to OECD 417 guideline.
- Justification for type of information:
- A discussion and report on the read across strategy is given as an attachment in Section 13.
- Reason / purpose for cross-reference:
- read-across: supporting information
- Qualifier:
- equivalent or similar to guideline
- Guideline:
- OECD Guideline 417 (Toxicokinetics)
- Deviations:
- not specified
- Principles of method if other than guideline:
- Though a GLP guideline is not specified in the study, the study was performed in a manner similar to the OECD 417 guideline.
- GLP compliance:
- no
- Specific details on test material used for the study:
- - Name of test material (as cited in study report): 1-Octene
- Substance type: C8 alpha olefin
- Analytical purity: 99% - Species:
- rat
- Strain:
- Sprague-Dawley
- Sex:
- male
- Details on test animals or test system and environmental conditions:
- TEST ANIMALS
- Source: Mollegaard A/S, L1 Skensved, Denmark
- Weight at study initiation: 150 to 200 grams
- Housing: 4 animals/cage
- Diet (e.g. ad libitum): Ad libitum
- Water (e.g. ad libitum): Ad libitum
- Acclimation period: 4 to 6 days
ENVIRONMENTAL CONDITIONS
- Temperature (°C): 23 +/- 1
- Humidity (%): 70 +/- 20 RH
- Photoperiod (hrs dark / hrs light): 10 hrs dark/14 hrs light
- Route of administration:
- inhalation
- Vehicle:
- other: air
- Details on exposure:
- TYPE OF INHALATION EXPOSURE: Whole body
GENERATION OF TEST ATMOSPHERE / CHAMBER DESCRIPTION
- Exposure apparatus: Conically shaped 0.7 cubic metre steel chambers with glass front door and walls
- Method of holding animals in test chamber: 4 animals/cage with 4 cages/chamber
- Source and rate of air: Source not provided; air rate at 5 m3/hr
- Concentration of test material in vehicle (if applicable): 100 parts per million in air
- Duration and frequency of treatment / exposure:
- All animals exposed for 12 hrs/day during daytime for 3 consecutive days.
- Remarks:
- Doses / Concentrations:
100 parts per million - No. of animals per sex per dose / concentration:
- Authors state that 4 animals/cage with a maximum of 4 cages per exposure chamber were used. A total of six separate experiments were performed. Specific details regarding number of animals used per experiment are not provided.
- Control animals:
- not specified
- Positive control reference chemical:
- Not applicable
- Details on study design:
- Details regarding dose selection or assignment of animals to the six experiments performed are not provided.
- Details on dosing and sampling:
- PHARMACOKINETIC STUDY (Absorption, distribution, excretion)
- Tissues and body fluids sampled (delete / add / specify): Blood, brain, liver, kidney, and fat
- Time and frequency of sampling: Immediately after 12 hrs exposure on days 1, 2, and 3 of the exposure period and 12 hrs after cessation of last exposure on day 3
- Statistics:
- Information regarding statistical analysis not provided.
- Preliminary studies:
- 1-Octene showed efficient absorption in the blood with extensive accumulation in the organs as compared to the iso-alkanes. Accumulation also was shown to increase with increasing carbon number. At day 3, concentrations of 1-octene were 12.4±0.5, 69.7±4.0, 78.9±9.7, 139.3±23.4, 720±176 μmol/kg in the blood, brain, liver, kidney, and fat, respectively. Concentrations of the 1-alkenes remained high in the fat even after the 12 hour recovery period, with 226±85 μmol/kg of 1-octene. According to the study authors, the extensive accumulation of 1-alkenes in the blood and organs as compared to other hydrocarbons may have toxicological significance and, therefore, products containing 1-alkenes should be handled cautiously to minimize inhalation exposure.
- Details on absorption:
- 1-Octene was efficiently absorbed into the blood.
- Details on distribution in tissues:
- 1-Octene was distributed in the following manner in the male Sprague-Dawley rats: fat>kidney>liver>brain>blood following measurements taken aft12 hours on exposure days 1, 2, and 3. However distribution in tissues following measurements taken 12 hours after exposure had ceased, indicated that fat had the highest concentration of 1-octene followed by the liver.
- Details on excretion:
- Data not provided
- Metabolites identified:
- not specified
- Conclusions:
- Interpretation of results (migrated information): other: Several organs showed bioaccumulation during exposure and following exposure cessation. However, no data are provided for bioaccumulation potential several days after exposure ended.
According to the study authors, the extensive accumulation of 1-alkenes in the blood and organs as compared to other hydrocarbons may have toxicological significance and, therefore, products containing 1-alkenes should be handled cautiously to minimize inhalation exposure. - Executive summary:
Male Sprague-Dawley rats were acclimatized for 4 to 6 days prior to exposure. Animals were housed 4/ cage with the number of cages limited to 4 per exposure chamber. Food and water were givenad libitum, except during exposure period. Animals were exposed to individual hydrocarbons selected for the experiment in six different experiments. The approximate concentration of the hydrocarbons (1 -alkenes andiso-alkanes) tested was 100 parts per million. All exposures occurred during daytime for 12 hours for 3 consecutive days. Concentrations of the hydrocarbons was measured in blood, brain, liver, kidney and perirenal fat immediately after 12 hour exposure on each exposure day, and 12 hours after the last exposure had been completed. The animals were removed one at a time from the exposure chamber and killed by decapitation. A standard process, not described in the article, made it possible to obtain blood and organ samples within 3 minutes of removal of animals from the exposure chamber.
Experimental results indicated that 1-octene was efficiently absorbed in the blood with extensive accumulation in the organs compared to the iso-alkanes. Accumulation also was shown to increase with increasing carbon number. At day 3, concentrations of 1-octene were 12.4±0.5, 69.7±4.0, 78.9±9.7, 139.3±23.4, 720±176 μmol/kg in the blood, brain, liver, kidney, and fat, respectively. Concentrations of 1-decene were 16.4±1.1, 138.1±2.7, 192.8±13.5, 162±22.9, and 2986±305 μmol/kg at day 3 in the blood, brain, liver, kidney, and fat, respectively. Concentrations of the 1-alkenes remained high in the fat even after the 12 hour recovery period, with 226±85 μmol/kg of 1-octene and 1971±134 μmol/kg of 1-decene. According to the study authors, the extensive accumulation of 1-alkenes in the blood and organs when compared to other hydrocarbons may have toxicological significance and, therefore, products containing 1-alkenes should be handled cautiously to minimize inhalation exposure.
Based on study design and results, this study is classified as reliable with restrictions because while there is no statement regarding whether this study was conducted according to GLP or equivalent, the information provided indicates that this study was conducted in a manner similar to OECD 417 guideline.
- Endpoint:
- basic toxicokinetics in vitro / ex vivo
- Type of information:
- experimental study
- Adequacy of study:
- supporting study
- Reliability:
- 2 (reliable with restrictions)
- Rationale for reliability incl. deficiencies:
- other: see 'Remark'
- Remarks:
- This study is classified as reliable with restrictions because while there is no statement regarding whether this study was conducted according to GLP or equivalent, adequate information is provided regarding study methods and test conditions. This study does not follow any specific guidelines because it is an in vitro toxicokinetic study.
- Justification for type of information:
- A discussion and report on the read across strategy is given as an attachment in Section 13.
- Reason / purpose for cross-reference:
- read-across: supporting information
- Objective of study:
- metabolism
- Qualifier:
- no guideline available
- Principles of method if other than guideline:
- Though this method does not follow any specific guidelines, it was well conducted and provides results relevant to chemical metabolism and toxicokinetics.
- GLP compliance:
- no
- Specific details on test material used for the study:
- - Name of test material (as cited in study report): n-1-Octene
- Substance type: C8 alpha olefin - Species:
- other: Rat liver microsomes
- Strain:
- Sprague-Dawley
- Sex:
- male
- Route of administration:
- other: In vitro
- Vehicle:
- other: Not applicable to this study
- Details on exposure:
- A total of four exposures were conducted during the course of the study with first one done to determine the test chemicals rate constant.
- Duration and frequency of treatment / exposure:
- Exposure no. Duration
1 10 to 40 minutes
2 60 minutes
3 30 minutes
4 30 minutes - Remarks:
- Doses / Concentrations:
Exposure Dose/Concentration
1 250mM
2 10 µM
3 10 µM
4 10 µM - No. of animals per sex per dose / concentration:
- Not applicable
- Control animals:
- other: Not applicable
- Positive control reference chemical:
- Not applicable
- Conclusions:
- Interpretation of results (migrated information): other: Formation of oct-1-ene metabolites
n-Octane-1,2-diol is the final metabolite of 1-octene when the chemical is reacted with rat liver microsomes in the presence of NADPH. The authors did not see the formation of this glycol metabolite when rat liver microsomes were incubated with the test chemical with NADP, NADH, and in the absence of NADPH. The authors also concluded that when an epoxide hydrolase inhibitor is used in the experiment both the intermediate epoxide metabolite (1,2-epoxy n-octane) and the glycol metabolite (n-Octane-1,2-diol) are formed in measurable quantities. Contrary to this, in the absence of epoxide hydrolase inhibitors only glycol metabolites were formed indicating that the formation of epoxide intermediates is a key step in 1-Octene metabolism in a liver microsome system. - Executive summary:
This study outlines an approach used to determine the role of epoxides as a key intermediate to 1-octene metabolism using various fractions of liver microsomes. Sprague-Dawley rat liver microsomes were homogenized at 4 degrees C in 2 volumes of isotonic KCI. Prior to using rat liver homogenate to determine the metabolic activation of -octene, the authors determined the rate constant of the test chemical by making a solution of 250 millimolar of 1-octene in chloroform and mixing it with 50 millimolar of perbenzoic acid at 10 degrees C and taking aliquots of this mixture at 10, 20 and 40 minutes and performing iodometric analyses to measure unchanged perbenzoic acid. Based on this experiment, the authors reported a rate constant (k) of 0.07 M-1min-1for n-1-octene. The purpose of this experiment in relation to the metabolism experiments is not clear and not explained in the study.
Following the determination of the rate constant, the authors conducted three metabolism related experiments: 1) Enzymatic oxidation of olefins; 2) Enzymatic hydrolysis of epoxides; and 3) Inhibition of epoxide hydrolase. The first two experiments were conducted using various fractions of the liver microsomes in combination with NADPH, NADP, NADH, or without addition of cofactors. The third experiment was conducted using various epoxide hydrolase inhibitors to determine the role of the epoxide metabolite in the metabolism of 1-octene.
Based on results obtained from these three experiments, the authors concluded that in the presence of rat microsomes and NADPH, n-1-octene was converted to glycolic products with no trace of epoxides. However, there was no conversion of 1-octene to the glycolic metabolites when the rat microsomes were incubated with the test chemical in the presence of NADP and NADH. In the second experiment similar results were obtained, with the formation of glycols from the epoxides in the presence of NADPH. In the third experiment, the authors used several epoxide hydrolase inhibitors added to a 1 -octene mixture and concluded that both the intermediate epoxide metabolite (1,2-epoxy n-octane) and the glycol metabolite (n-octane-1,2-diol) were formed in measurable quantities under the experimental conditions used. Contrary to this, in the absence of epoxide hydrolase inhibitors, only glycol metabolites were formed indicating that the formation of epoxide intermediates is a key step in 1-Octene metabolism in a liver microsome system. Based on the results obtained from these experiments, the authors concluded that the epoxide is an obligatory intermediate in the conversion of olefins to the corresponding glycols.
This study received a Klimisch score of 2 and is classified as reliable with restrictions because while there is no statement regarding whether this study was conducted according to GLP or equivalent, adequate information is provided regarding study methods and test conditions. This study does not follow any specific guidelines because it is an in vitro toxicokinetic study.
[This study was selected as a supportive study because…]
- Endpoint:
- basic toxicokinetics in vitro / ex vivo
- Type of information:
- experimental study
- Adequacy of study:
- supporting study
- Study period:
- 1985
- Reliability:
- 2 (reliable with restrictions)
- Rationale for reliability incl. deficiencies:
- other: see 'Remark'
- Remarks:
- This study is classified as reliable with restrictions because while there is no statement regarding whether this study was conducted according to GLP or equivalent, the study appears to be well conducted and provides important information on the metabolism of 1-octene.
- Justification for type of information:
- A discussion and report on the read across strategy is given as an attachment in Section 13.
- Reason / purpose for cross-reference:
- read-across: supporting information
- Objective of study:
- metabolism
- Qualifier:
- no guideline available
- Principles of method if other than guideline:
- Though this method does not follow any specified guidelines, it was well conducted and provides results relevant to chemical metabolism and toxicokinetics.
- GLP compliance:
- not specified
- Specific details on test material used for the study:
- - Name of test material (as cited in study report): Oct-1-ene
- Substance type: C8 alpha olefin - Radiolabelling:
- no
- Species:
- other: Rat liver microsomes
- Strain:
- Fischer 344
- Sex:
- male
- Route of administration:
- other: In vitro
- Vehicle:
- DMSO
- Details on exposure:
- Experiment 1: Rat liver microsomes were incubated with octene and a NADPH genterating system. Incubation was at 37 degrees in a shaking water bath until reactions were stopped by taking 0.2 mL of the mixture and adding it to 40 ul 0.1 M NaOH in tubes on ice.
Experiment 2: Rat liver microsomes were incubated with octene, N-acetylcysteine, and a NADPH generating system at 37 degrees Celsius. Reactions were stopped by adding HCl. - Duration and frequency of treatment / exposure:
- Experiment 1: Incubation time not reported
Experiment 2: Varied incubation periods - Remarks:
- Doses / Concentrations:
10 μL in dimethyl sulphoxide - No. of animals per sex per dose / concentration:
- Not reported
- Control animals:
- no
- Positive control reference chemical:
- Not applicable
- Details on study design:
- Microsomal fractions were prepared from the liver and other organs. Intestinal microsomes were prepared from the duodenal segment. The lumen was perfused with ice-cold saline and the tissue was sectioned longitudinally. These microsomal fractions were used to determine microsomal conversion of 1-octene to octane-1-2-diol and S-3 -oxo octyl-N-acetylcysteine. Based on the experiments performed, the authors describe two metabolic pathways when 1-octene is administered as a single acute dose.
- Conclusions:
- Interpretation of results (migrated information): other: Activation of oct-1-ene occurs via two separate reaction pathways
The study authors concluded that 1-octene when incubated with rat liver in the presence of NADPH, undergoes metabolic activation to an intermediate which was trapped with N-acetylcysteine, as S3-oxo-octyl-N-acetylcysteine. They also concluded that relative to the formation of the S3-oxo-octyl-N-acetylcysteine intermediate, the classical route of activation of octene via the 1,2 epoxide followed by hydrolysis to octane-1,2-
diol is about 40 times higher. In addition, the authors reported that unlike other epoxides prepared chemically that tend to have carcinogenic or mutagenic properties, the 1-octene derived epoxide will not be hepatotoxic when the test chemical is administered as a single acute dose. - Executive summary:
Male Fisher 344/N rats were used in this toxicokinetic study. The authors reported that in some instances the animals were pretreated with either phenobarbitone, 3 -methylcholanthrene, or 2 -(p-chlorophenoxy)-2 -methyl propionic acid via drinking water (phenobarbitone only) or intraperitoneally for 7, 3 and 4 days respectively to induce mixed function oxidase activity. All animals were killed 24 hours after the last dose. In one experiment, animals pretreated with phenobarbitone were given 1-octene at 100 mg/kg in trioctanoyl glycerol and sacrificed 2 hours after dosing. Details regarding number of animals and experiments are not provided. Following animal sacrifice, microsomal fractions were prepared from the liver and other organs. Intestinal microsomes were prepared from the duodenal segment. The lumen was perfused with ice-cold saline and the tissue was sectioned longitudinally. These microsomal fractions were used to determine mircosomal conversion of 1-octene to octane-1-2-diol and S-3 -oxo octyl-N-acetylcysteine. Based on the experiments performed, the authors describe two metabolic pathways when 1-octene is administered as a single acute dose.
In the first pathway, 1-octene was converted to octane-1,2-oxide, which was rapidly hydrolysed to octane-1,2 -diol using an in vitro NADPH generating system. In the second pathway, 1-octene was metabolized to octane-3-ol in the presence of NADPH and cytochrome P-450, which was then converted to octen-3-one in the presence of NAD(P) and then finally converted to S-3-oxo-octyl-N-acetylcysteine in the presence of N-acetylcysteine. Octen-3-one reacts non-enzymatically with N-acetylcysteine to form S-3-oxo-octyl-N-acetylcysteine with a half-life of about 6 seconds. The rate of conversion for 1-octene, but not octen-3-ol, was influenced by the pretreatment of rats with phenobarbitone or 3-methylcholanthrene, known inducers of cytochrome P-450. Octen-3-ol was at least 2 -fold more reactive than 1-octene indicating the activation of 1-octene is a rate limiting step. Microsomal preparations of the following tissues metabolized 1-octene to S-3-octyl-N-acetylcysteine: liver, adrenal, intestine, lung, kidney, spleen, and brain.
Metabolism of 1-octene via its 1,2 epoxide to octane-1,2-diol (pathway 1) was more than 40 times more rapid than its metabolism to S-3-oxo-octyl-N-acetylcysteine (pathway 2). N-(2-hydroxyoctyl)protoporhyrin IX, an adduct formed by the alkylation of the cytochrome P450 haem, was found in the livers of rats administered a single intraperitoneal injection of 100 mg/kg of 1-octene in trioctanoyl glycerol after pretreatment with phenobarbitone (0.1%) in the drinking water for 7 days, as well as in microsomal mixtures. The formation of this adduct was found to be 17-fold lower via the first pathway compared to the second pathway. In addition to determining the metabolic pathway in an in vitro system, the authors reported that 1-octene derived epoxides will serve as substrates for both epoxide hydrolase and glutathione transferase and are not likely to be acutely hepatotoxic.
This study was given a Klimisch score of 2 and is classified as reliable with restrictions because while there is no statement regarding whether this study was conducted according to GLP or equivalent, the study appears to be well conducted and provides important information on the metabolism of 1-octene.
Referenceopen allclose all
Description of key information
Olefinic structures related to substances included in this category are metabolised to diols via an epoxide intermediate by hepatic microsomal enzymes.
The position of the double bond as well as the degree of substitution influences this metabolism, with alpha olefins with no adjacent branching appearing more biologically reactive relative to internal and/or branched olefins.
Key value for chemical safety assessment
Additional information
Metabolism in vivo
The in vivo absorption and distribution of a number of linear alpha olefins (oct-1-ene, non-1-ene, dec-1-ene) and their corresponding isoalkanes in the rat after inhalation exposure was investigated by Zahlsen et al. (1993). Male Sprague-Dawley rats (16/dose) were exposed via whole body inhalation to 100 ppm vapour of the individual test substances for 12 hours/day for 3 consecutive days. Concentrations of the hydrocarbons were measured in blood, brain, liver, kidney and perirenal fat immediately following each 12 hour exposure and 12 hours following the last exposure. Higher concentrations of linear alpha olefins were measured in each of the respective organs compared with measured concentrations of the corresponding isoalkanes, with the highest concentrations measured in fat. All measured concentrations of linear alpha olefins and isoalkenes were significantly decreased following the 12 hour recovery period; however the largest residual accumulations were measured in the fat. With the exception of the kidney, accumulation of the respective test substances in all organs was shown to increase with increasing carbon number.
Metabolism in vitro
Montellano and Mico (1980) demonstrated the NADPH-dependent destruction of hepatic microsomal cytochrome P-450 from phenobarbital pre-treated rats by ethylene and other olefins in vitro. The study demonstrated loss of cytochrome P-450 due to alkylation of the haeme function, with mono-oxygenase inactivation occurring to a greater extent with linear and branched alpha olefins relative to that found with internal olefins (with or without branching) where no or minimal loss of cytochrome P-450 was detected. The level of substitution in the region of the olefin double bond appeared to influence the extent of haemoprotein inactivation (2-methyl-1-heptene was inactive while 1-heptene was active).
In an in vitro study designed to determine if carbon-carbon double bonds are metabolized to glycols via direct dihydroxylation of the olefinic bond or via epoxide intermediates, complete metabolic conversion of oct-1-ene to glycolic products was accomplished using an NADPH generating system and rat liver microsomes (Maynert et al., 1970). When competitive inhibitors of epoxide hydrolase were used in the experiment, both epoxides and glycols were formed. In the absence of the inhibitor, only glycols were formed confirming that the metabolism of olefins proceeds via epoxide intermediates. While hydrolysis of the oxirane ring by epoxides hydrolase is rapid for alpha-olefins, glycol formation for internal- and branched chain olefins is less efficient due to steric hindrance in the region of the oxirane ring by alkyl groups. This can be illustrated for a series of C8-olefins, where hydrolysis of the epoxide intermediate follows the trend below (Maynert, 1970):
1,2 epoxy-n-C8 > 4,5 epoxy-n-C8 > 2,3 epoxy-iso-C8
Epoxide formation via cytochrome P-450 dependent processes is similarly subject to steric hindrance, with ready accessibility of the alpha double bond in linear olefins contrasting with the relative inaccessibility of the double bond in internal olefins (Maynert et al., 1970).
Similar principles were demonstrated by Leibman and Ortiz (1970), who evaluated the role of epoxides as intermediaries in the microsomal oxidation of olefins to glycols. Hepatic microsomal fractions from phenobarbital induced rabbits were used along with tested compounds xylene, indene, and cyclohexene. Using gas chromatography and thin layer chromatography, the authors demonstrated that epoxides were intermediates in the oxidation of alkenes to glycols.
In another study, two metabolic pathways for oct-1-ene were described by White et al. (1986). Oct-1-ene was converted to octane-1,2-oxide which was rapidly hydrolyzed to octane-1,2-diol using an in vitro NADPH generating system (pathway 1). An alternative metabolic route was described in which oct-1-ene was converted to a reactive intermediate, octen-3-one, which formed S-3-oxo-octyl-acetylcysteine in in vitro systems using NADPH, cytochrome P450, NAD(P), and N-acetylcysteine (pathway 2). Metabolism of oct-1-ene via pathway 1 was significantly more rapid (40 times) that metabolism via pathway 2.
In a study that investigated the relative importance of electronic and steric factors in determining the suicide substrate activity of terminal alkenes, Luke et al. (1990) used ab initio quantum mechanics coupled with molecular mechanics and the known crystal structure of cytochrome P-450 to assess the relative potency of three prototypical terminal olefins (ethylene and propene, known suicide substrates; 2-methylpropene, known to be inactive as a suicide substrate) towards cytochrome P-450. The observations from this study suggested that while an unsaturated bond appeared necessary for haemoprotein alkylation, only terminal olefins seemed capable of binding irreversibly to cytochrome P-450 with steric hindrance, due to branching, playing a modulating role (e.g. 2-methyl-1-heptene was modelled as inactive with regard to haeme alkylation). The heme alkylation reaction runs in parallel and is independent of epoxide formation. Steric hindrance by substitution at the terminal double bond favours epoxides formation over heme alkylation. Internal olefins (such as cis/trans 3-hexene and cyclohexene) appeared capable of being metabolised to epoxides but were predicted inactive with regard to the haeme alkylation. The authors concluded that a terminal double bond, with no immediate alkyl substitutes, was a likely requirement for haeme alkylation, suggesting that alpha olefins might be biologically more reactive than internal and/or branched olefins.
The relationship between allylic oxidation and epoxidation of alkenes (with increasing alkyl substitution on an olefin promoting allylic oxidation at the expense of epoxidation) was examined by Gorycki and MacDonald (1994) using hexamethyl Dewar benzene (HMDB); 1,2-dimethylcyclohexene (DMCH); and 1,2,4,5-tetramethyl-1,4-cyclohexadiene (TMCH). These chemicals were selected to probe the intermediacy of an alkene radical cation during cytochrome P-450 catalyzed epoxidation. Cytochrome P-450 catalyzed the allylic oxidation of HMDB exclusively; no rearranged products nor epoxide was detected. Additionally, cytochrome P-450 exclusively catalyzed the allylic oxidation of 1,2-dimethylcyclohexene (DMCH) and 1,2,4,5-tetramethyl-1,4-cyclohexadiene (TMCH). The double bonds of HMDB and DMCH were readily epoxidized by (tetrapheny1porphyrinato) iron-(III) chloride with minor production of allylic alcohols. The results appear to indicate that in this model systemt he rates of alkene epoxidation tend to increase with increasing alkene substitution, but it is apparent that cytochrome P-450 has additional mechanistic options or constraints that depress the rate of epoxidation with increasing alkene substitution.
Information on Registered Substances comes from registration dossiers which have been assigned a registration number. The assignment of a registration number does however not guarantee that the information in the dossier is correct or that the dossier is compliant with Regulation (EC) No 1907/2006 (the REACH Regulation). This information has not been reviewed or verified by the Agency or any other authority. The content is subject to change without prior notice.
Reproduction or further distribution of this information may be subject to copyright protection. Use of the information without obtaining the permission from the owner(s) of the respective information might violate the rights of the owner.
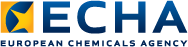