Registration Dossier
Registration Dossier
Data platform availability banner - registered substances factsheets
Please be aware that this old REACH registration data factsheet is no longer maintained; it remains frozen as of 19th May 2023.
The new ECHA CHEM database has been released by ECHA, and it now contains all REACH registration data. There are more details on the transition of ECHA's published data to ECHA CHEM here.
Diss Factsheets
Use of this information is subject to copyright laws and may require the permission of the owner of the information, as described in the ECHA Legal Notice.
EC number: 230-386-8 | CAS number: 7085-19-0
- Life Cycle description
- Uses advised against
- Endpoint summary
- Appearance / physical state / colour
- Melting point / freezing point
- Boiling point
- Density
- Particle size distribution (Granulometry)
- Vapour pressure
- Partition coefficient
- Water solubility
- Solubility in organic solvents / fat solubility
- Surface tension
- Flash point
- Auto flammability
- Flammability
- Explosiveness
- Oxidising properties
- Oxidation reduction potential
- Stability in organic solvents and identity of relevant degradation products
- Storage stability and reactivity towards container material
- Stability: thermal, sunlight, metals
- pH
- Dissociation constant
- Viscosity
- Additional physico-chemical information
- Additional physico-chemical properties of nanomaterials
- Nanomaterial agglomeration / aggregation
- Nanomaterial crystalline phase
- Nanomaterial crystallite and grain size
- Nanomaterial aspect ratio / shape
- Nanomaterial specific surface area
- Nanomaterial Zeta potential
- Nanomaterial surface chemistry
- Nanomaterial dustiness
- Nanomaterial porosity
- Nanomaterial pour density
- Nanomaterial photocatalytic activity
- Nanomaterial radical formation potential
- Nanomaterial catalytic activity
- Endpoint summary
- Stability
- Biodegradation
- Bioaccumulation
- Transport and distribution
- Environmental data
- Additional information on environmental fate and behaviour
- Ecotoxicological Summary
- Aquatic toxicity
- Endpoint summary
- Short-term toxicity to fish
- Long-term toxicity to fish
- Short-term toxicity to aquatic invertebrates
- Long-term toxicity to aquatic invertebrates
- Toxicity to aquatic algae and cyanobacteria
- Toxicity to aquatic plants other than algae
- Toxicity to microorganisms
- Endocrine disrupter testing in aquatic vertebrates – in vivo
- Toxicity to other aquatic organisms
- Sediment toxicity
- Terrestrial toxicity
- Biological effects monitoring
- Biotransformation and kinetics
- Additional ecotoxological information
- Toxicological Summary
- Toxicokinetics, metabolism and distribution
- Acute Toxicity
- Irritation / corrosion
- Sensitisation
- Repeated dose toxicity
- Genetic toxicity
- Carcinogenicity
- Toxicity to reproduction
- Specific investigations
- Exposure related observations in humans
- Toxic effects on livestock and pets
- Additional toxicological data

Adsorption / desorption
Administrative data
Link to relevant study record(s)
- Endpoint:
- adsorption / desorption: screening
- Type of information:
- experimental study
- Adequacy of study:
- supporting study
- Reliability:
- 2 (reliable with restrictions)
- Rationale for reliability incl. deficiencies:
- study well documented, meets generally accepted scientific principles, acceptable for assessment
- Qualifier:
- no guideline followed
- Principles of method if other than guideline:
- The experimental data from phenoxy herbicide sorption experiments was collated and reviewed including data on the test material.
- GLP compliance:
- not specified
- Remarks:
- Publication
- Type of method:
- other: Not specified
- Details on results (Batch equilibrium method):
- SORPTION OF PHENOXY HERBICIDES IN SOIL:
Differences between the mean log Kd values of phenoxy herbicides were not statistically significant. Standard deviations indicate that logarithmic Kd values of phenoxy herbicides range over at least 2 orders of magnitude. The most comprehensive data set was assembled for 2,4-D. For 2,4-D, the fitted normal distribution with a mean of 0.16 and standard deviation of 0.55 suggests that 68 % of the Kd values are within a range from 0.4 to 5.1 (in litres per kilogram) and 95 % within a range from 0.11 to 18 (in litres per kilogram).
SORPTION OF 2,4-D AND MCPP TO WELL-DEFINED SORBENTS
Kd data characterising 2,4-D and test material sorption to the well-defined sorbent materials quartz, calcite, α-alumina, kaolinite, ferrihydrite, goethite, lepidocrocite, soil humic acid, Fluka, humic acid, and Pahokee peat are summarised in Table 'Sorbent–water distribution coefficients Kd for 2,4-D and MCPP in 0.01 M CaCl2 solutions'. Amongst the minerals, iron oxides of poorly crystallized twoline ferrihydrite (Fe2O3·nH2O), goethite (α-FeOOH), and lepidocrocite (γ-FeOOH) are the best sorbents for phenoxy herbicides because of their high PZNC and large surface area. Soil humic acid, Fluka humic acid, and Pahokee peat also have fairly high weight-normalized sorption coefficients Kd in comparison with quartz, calcite, kaolinite, and montmorillonite.
COMPARISON OF PHENOXY HERBICIDES SORPTION IN SOIL
The standard deviations of log Kd values compiled indicate that log Kd values of phenoxy herbicides range over at least 2 orders of magnitude, and given this variability, a comparison of compound specific sorption should be done for identical soils. Unfortunately, only a few comparative studies investigate the sorption of a range of phenoxy herbicides on identical soils. Haberhauer et al. (2000) found for four Austrian or German agricultural soils that adsorption generally increased in the order test material< MCPA<2,4-DP<2,4-D. These authors suggest that adsorption is increased if a methyl group of the phenoxy group is replaced with a chlorine which increases the molecular volume and may also change polarity (for instance MCPA vs. 2,4-D and test material vs. 2,4-DP). On the other hand, Thorstensen et al. (2001) found for three Norwegian soils that MCPA Freundlich isotherm sorption coefficients were about a factor of 2 higher than those of 2,4-DP. Hiller et al. (2008) found for six soils from Slovakia that 2,4-D Freundlich isotherm sorption coefficients were mostly somewhat higher than MCPA Freundlich isotherm sorption coefficients by up to a factor of 2. For the mean log Kd values, the order is test material < MCPA < 2,4 DP < MCPB < 2,4-D < 2,4-DB. This seems to be in good agreement with the comparative literature studies. The higher pKa and greater hydrophobic volume of the butanoic acid as compared to the acetic acid group should facilitate partitioning from water into soil organic matter and could explained the greater Kd values of 2,4-DB vs. 2,4-D and MCPB vs. MCPA. On the other hand, a higher pKa may also imply reduced adsorption to positively charged mineral surfaces.
For well-defined materials, Tulp et al. (2009) found that Koc values of peat for the anions of phenoxy herbicides were 11, 21, and 93 L/kg for the test material, 2,4-D, and 2,4-DB, respectively. The authors argue that the additional two CH2 groups explain the higher Koc for 2,4-DB as compared to 2,4-D. Clausen et al. (2001) found that 2,4-D and the test material sorption distribution coefficients are quite comparable for quartz, calcite, and α-alumina, but Clausen and Fabricius (2001) found that they are generally a factor of 2–3 larger for the test material than 2,4-D for ferrihydrite and goethite in the presence of CaCl2. They explain this difference with different sorption mechanisms suggesting that the test material can also form inner-sphere complexes with iron minerals.
SORPTION MECHANISMS
Weak organic acids such as the phenoxy herbicides can bind via several mechanisms. In their anionic form, which dominates for most compounds under most soil pH conditions, the pesticides may adsorb to mineral surfaces, although typically only near or below the PZNC. Phenoxy herbicides may also adsorb with their deprotonated carboxyl groups to positive sites on the surface of calcite or α-alumina. For clays, an absence of adsorption is observed in experiments without electrolytes; however, some adsorption may occur in experiments at low pH and/or with electrolyte solution. Adsorption of carboxylic acids to iron oxides has been interpreted in terms of monodentate or bidentate complex formation (Clausen and Fabricius 2001; Iglesias et al. 2010). In addition, the anionic and nonionic form of the pesticide may partition into soil organic matter, which is increasingly important at low soil pH (Celis et al. 1999; Tulp et al. 2009). Since 2,4-D has a pKa of 2.85, its anionic form will dominate at most soil pH values. Only 6 % of the soils in the assembled data set had a pH below 4.
SORPTION TO QUARTZ, CALCITE, ALUMINUM OXIDES, AND CLAYS
According to Clausen et al. (2001) 2,4-D sorbs measurably to quartz at low pH, but at pH 6.5 adsorption is not significant. Phenoxy herbicides may adsorb with their –COO− groups to positive sites on the surface of calcite and α-Al2O3 (Clausen et al. 2001). According to Goyne et al. (2004), 2,4-D sorbs rapidly and reversibly by electrostatic interaction, i.e. outersphere complexation, to Al2O3. According to Clausen et al. (2001) phenoxy herbicides adsorb only minimally to kaolinite. Celis et al. (1999) show that 2,4-D sorption to Ca-saturated montmorillonite as a single sorbent is minimal. Iron precipitation or adsorption on the surface of montmorillonite results, however, in significant adsorption, partially also due to a decrease in pH during preparation of the binary sorbents.
SORPTION TO IRON OXIDES
According to Clausen and Fabricius (2001) 2,4-D adsorption to the iron oxides of poorly crystallized two-line ferrihydrite, goethite, and lepidocrocite is significant below the PZNC and increases five to tenfold with decreasing pH value between pH 5.6 and 3.6. Thirty-eight percent of the 271 Kd values for 2,4-D compiled for this review were measured in soils with a pH below 5.6. For the test material, a similar pH dependency is observed, but the test material adsorption is already significant to all iron oxides at the PZNC. Iglesias et al. (2010) demonstrate that coating of iron oxide surfaces by humic acid increases MCPA adsorption for low aqueous pesticide concentrations, but decreases MCPA adsorption at high aqueous pesticide concentrations.
INFLUENCE OF THE IONIC COMPOSITION OF THE SOLUTION: CALCIUM CHLORIDE
Hyun and Lee (2005) find that sorption in the absence of Ca2+ (using KCl as electrolyte) diminished 2,4-D sorption by up to a factor of 2 for soils in which kaolinite or quartz is the dominant sorbent material. For soils containing mainly gibbsite, the effect of Ca2+ was negligible. Moreale and Van Bladel (1980) also find that 2,4-D sorption decreases by up to a factor of 3 when comparing 1.25 M CaCl2 as solution with H2O, although they note that pH increased with decreasing CaCl2 concentration. For well-defined materials, it was found that CaCl2 solutions increase test material and 2,4-D sorption to clay (kaolinite) and quartz surfaces, but it decreases the test material and 2,4-D sorption to calcite and α-alumina (Clausen et al. 2001). The latter phenomena could be explained by increasing competition for the same sorption sites between Cl− and anionic pesticides, increased aqueous complexation of anionic pesticides with the Ca2+ cations, and decreased activity of the anionic pesticides caused by increased electrolyte solution. For instance, Cl− is known to adsorb to α-alumina and could compete with anionic pesticides for adsorption sites on the α-alumina surface. On the other hand, Ca2+ can sorb onto silanol surface sites and thereby facilitate the attachment of anionic pesticides. Such complexes can explain the increased test material and 2,4-D sorption to clay (kaolinite) and quartz surfaces with increasing CaCl2 concentration at a pH for which the surface is negatively charged (pH 6 for kaolinite, pH 6.7 for quartz). Clausen and Fabricius (2001) found a substantial decrease in phenoxy herbicide adsorption to iron oxides with increasing CaCl2 concentration when comparing 0 and 0.0025 M CaCl2 as solution, and fairly consistent values between 0.0025 and 0.01 M CaCl2. Tulp et al. (2009) found that 2,4-D and test material anion sorption to peat increases by about a factor of 3.5 as Ca2+ concentration increases from 0.001 to 0.1 M. They conclude that for typical environmental concentrations, the impact should be fairly small. - Validity criteria fulfilled:
- not specified
- Conclusions:
- The data review suggests that sorption of 2,4-D can be rationalised based on the soil parameters pH, foc, Oxalate Fe, and DCB Fe in combination with sorption coefficients measured independently for humic acids and ferrihydrite, and goethite. Soil organic matter and iron oxides appear to be the most relevant sorbents for phenoxy herbicides. The mean log Kd (L/kg) was found to be – 0.38 for the test material, the pKa and log Kow were also reported as 3.1 and 3.13.
- Executive summary:
2,4-dichlorophenoxyacetic acid (2,4-D) and other phenoxy herbicide sorption experiments were reviewed.
A database with 469 soil–water distribution coefficients Kd (in litres per kilogram) was compiled: 271 coefficients are for the phenoxy herbicide 2,4-D, 9 for 4-(2,4- dichlorophenoxy)butyric acid, 18 for 2-(2,4-dichlorophenoxy)propanoic acid, 109 for 2-methyl-4-chlorophenoxyacetic acid, 5 for 4-(4-chloro-2-methylphenoxy)butanoic acid, and 57 for the test material. The following parameters characterising the soils, solutions, or experimental procedures used in the studies were also compiled if available: Solution CaCl2 concentration, pH, pre-equilibration time, temperature, soil organic carbon content (foc), percent sand, silt and clay, oxalate extractable aluminium, oxalate extractable iron (Oxalate Fe), dithionite–citrate–bicarbonate extractable aluminium, dithionite–citrate–bicarbonate extractable iron (DCB Fe), point of zero negative charge, anion exchange capacity, cation exchange capacity, soil type, soil horizon or depth of sampling, and geographic location. Kd data were also compiled characterising phenoxy herbicide sorption to the following well-defined sorbent materials: Quartz, calcite, α-alumina, kaolinite, ferrihydrite, goethite, lepidocrocite, soil humic acid, Fluka humic acid, and Pahokee peat.
The data review suggests that sorption of 2,4-D can be rationalised based on the soil parameters pH, foc, Oxalate Fe, and DCB Fe in combination with sorption coefficients measured independently for humic acids and ferrihydrite, and goethite.
Soil organic matter and iron oxides appear to be the most relevant sorbents for phenoxy herbicides. The mean log Kd (L/kg) was found to be – 0.38 for the test material, the pKa and log Kow were also reported as 3.1 and 3.13.
- Endpoint:
- adsorption / desorption: screening
- Type of information:
- experimental study
- Adequacy of study:
- supporting study
- Study period:
- 08 May 1986 to 02 June 1986
- Reliability:
- 2 (reliable with restrictions)
- Rationale for reliability incl. deficiencies:
- study well documented, meets generally accepted scientific principles, acceptable for assessment
- Qualifier:
- according to guideline
- Guideline:
- other: Section 163-1 of Subdivision N of the EPA Pesticide Assessment Guidelines.
- Deviations:
- no
- GLP compliance:
- yes (incl. QA statement)
- Type of method:
- other: Soil TLC
- Media:
- soil
- Radiolabelling:
- yes
- Test temperature:
- Room temperature.
- Analytical monitoring:
- no
- Matrix no.:
- #1
- Matrix type:
- sand
- % Clay:
- 2
- % Silt:
- 8
- % Sand:
- 90
- % Org. carbon:
- 0.8
- pH:
- 5.6
- CEC:
- 1 meq/100 g soil d.w.
- Bulk density (g/cm³):
- 1.62
- Matrix no.:
- #2
- Matrix type:
- sandy loam
- % Clay:
- 10
- % Silt:
- 34
- % Sand:
- 56
- % Org. carbon:
- 2.3
- pH:
- 7.6
- CEC:
- 9 meq/100 g soil d.w.
- Bulk density (g/cm³):
- 1.22
- Matrix no.:
- #3
- Matrix type:
- silt loam
- % Clay:
- 23
- % Silt:
- 64
- % Sand:
- 13
- % Org. carbon:
- 2.1
- pH:
- 7
- CEC:
- 15 meq/100 g soil d.w.
- Bulk density (g/cm³):
- 1.34
- Matrix no.:
- #4
- Matrix type:
- silty clay loam
- % Clay:
- 34
- % Silt:
- 42
- % Sand:
- 24
- % Org. carbon:
- 2.5
- pH:
- 6.6
- CEC:
- 14.7 meq/100 g soil d.w.
- Bulk density (g/cm³):
- 1.21
- Details on matrix:
- COLLECTION AND STORAGE
- Geographic location: Mississippi silt loam was collected from a delta farm in Mississippi. Fox sandy loam was collected from a farm near Madison in Dane County, Wisconsin. Plainfield sand was collected near the south shore of Petenwell Lake in Adams County, Wisconsin. Hagerstown silty clay loam was collected near Lime Kiln in Frederick County, Maryland.
- Soil preparation: Before use, the soils were passed through a 1.18 mm sieve.
PROPERTIES
- Field moisture capacity: 20.1 % (silt loam), 20.3 % (sand), 21.0 % (sandy loam), 31 % (silty clay loam). - Details on test conditions:
- PLATE PREPARATION
- A TLC linear anaylser was used to determine the distribution of radioactivity on TLC plates.
- Each soil was sieved through a 1.18 mm screen. A tape strip was placed 17.5 cm from one edge of each 20 cm x 20 cm glass plate. For each soil type, a slurry of soil and water was prepared, coated uniformly on a 20 cm x 17.5 cm area of the plate, and dried. Channels were then cut in the soil to separate each plate into seven strips, each approximately 17.5 cm long and 2.5 cm wide.
SAMPLE ANALYSIS
- Each strip or channel of each plate was spotted at the origin (about 1.5 cm from the bottom) with a solution containing approximately 0.01 to 0.03 μCi of test material After solvent evaporation, the glass plate was developed in water at room temperature until the solvent front reached the top of each strip. The plate was then dried, and the location of radioactivity in each strip was determined by using the linear analyser.
- Frontal Rf values were calculated from each linear analyser scan by measuring the distance from the spotting location to the leading edge of the radioactivity and dividing by the distance from the spotting location to the solvent front. Three determinations were made for the test material in each soil, while the reference standards were determined in duplicate.
- A mobility class was assigned to each pesticide in each soil based on its frontal Rf value.
- A sorption coefficient (K) was determined. - Sample No.:
- #1
- Type:
- other: K
- Value:
- 0.4 dimensionless
- pH:
- 5.6
- Matrix:
- Plainfield sand
- % Org. carbon:
- 0.8
- Sample No.:
- #2
- Type:
- other: K
- Value:
- 0.481 dimensionless
- pH:
- 7.6
- Matrix:
- Fox sandy loam
- % Org. carbon:
- 2.3
- Sample No.:
- #3
- Type:
- other: K
- Value:
- 0.457 dimensionless
- pH:
- 7
- Matrix:
- Mississippi silt loam
- % Org. carbon:
- 2.1
- Sample No.:
- #4
- Type:
- other: K
- Value:
- 0.78 dimensionless
- pH:
- 6.6
- Matrix:
- Hagerstown silty clay loam
- % Org. carbon:
- 2.5
- Transformation products:
- not measured
- Details on results (Batch equilibrium method):
- The test material was classified as very mobile in Plainfield sand, Mississippi silt loam, and Fox sandy loam, and as mobile in Hagerstown silty clay loam.
Mean frontal Rf values:
- Plainfield sand: 1.0
- Fox sandy loam: 0.93
- Mississippi silt loam: 0.95
- Hagerstown silty clay loam: 0.74 - Validity criteria fulfilled:
- not applicable
- Conclusions:
- Under the conditions of this study, the test material was classified as very mobile in Plainfield sand, Mississippi silt loam, and Fox sandy loam, and as mobile in Hagerstown silty clay loam. The sorption coefficient calculated from relative mobility was 0.4 for Plainfield sand, 0.481 for Fox sandy loam, 0.47 for Mississippi silt loam and 0.780 for Hagerstown silty clay loam.
- Executive summary:
The mobility of the test material in four soils using soil thin layer chromatography was investigated in compliance with GLP.
Glass plates were coated with a thin layer of one of four different soils. Radiolabelled test material was applied, and water was allowed to migrate up the plates. The distribution of radioactivity on each soil was then determined using a linear analyser. The frontal relative migration (Rf) value of each was measured and the mobility determined.
Under the conditions of this study, the test material was classified as very mobile in Plainfield sand, Mississippi silt loam, and Fox sandy loam, and as mobile in Hagerstown silty clay loam. The sorption coefficient calculated from relative mobility was 0.4 for Plainfield sand, 0.481 for Fox sandy loam, 0.47 for Mississippi silt loam and 0.780 for Hagerstown silty clay loam.
- Endpoint:
- adsorption / desorption: screening
- Type of information:
- experimental study
- Adequacy of study:
- supporting study
- Reliability:
- 2 (reliable with restrictions)
- Rationale for reliability incl. deficiencies:
- study well documented, meets generally accepted scientific principles, acceptable for assessment
- Qualifier:
- no guideline followed
- Principles of method if other than guideline:
- The impact of the herbicide concentration (0.10 - 10 000 mg kg^-1) and addition of organic and inorganic nutrients on the test material mineralisation in aquifer and soil samples was studied in laboratory experiments.
- GLP compliance:
- not specified
- Remarks:
- Publication
- Details on sampling:
- Surface soil, aquifer sediment and groundwater were collected from a Danish site located near Fladerne Bæk in Jutland. This area is moorland plain with melt-water sediments dominated by coarse sand (Jacobsen et al., 1998). The area is used for farming and has previously been treated with the test material.
- Details on matrix:
- Details and descriptions of the characteristics of the site have been published previously (Jacobsen et al., 1998; Larsen et al., 2000; Sørensen et al., 2006). Aquifer sediment was collected as cores of approximately 1 m length with a 5.5 cm diameter stainless steel piston sampler as described by Larsen et al. (2000). Cores were collected in the saturated zone approximately 3 m below surface and stored at 4 °C until partitioning. Upon the return to the laboratory, 2 cm at the end of each core and the outermost 0.5 cm were aseptically removed using a paring device and discarded. Groundwater was sampled 3 m below surface, using a peristaltic pump, through a Teflon tube that was lowered into a 2 cm diameter well. Electric conductivity, pH and the oxygen concentration were measured passing freshly pumped groundwater through a flow cell equipped with electrodes (EC 320, pH 320 and Oxi 320, WTW, Germany). Surface soil (5 - 20 cm) was collected by digging, sieved through a 4 mm mesh and finally stored at 4 °C. The total carbon content of dry sediment and soil samples was determined upon return to the laboratory by combustion using a LECO® CHN-2 000 carbon, hydrogen and nitrogen analyser (LECO Corporation, St. Joseph, MI). Both sediment and soil samples were homogenised thoroughly by hand using a sterile spatula prior to set-up of laboratory experiments.
- Details on test conditions:
- Herbicide mineralisation
Soil and sediment corresponding to 15.0 g (dry weight) were added to 100 mL airtight glass flasks. Then, 15 mL of groundwater was added to sediment, while to soil was added sterile Milli-Q water in order to obtain a water content of 20 %. Subsequently [ring-U-14C]test material (Izotop, Budapest, Hungary; radiochemical purity > 95 %), [ring-U-14C] was added, corresponding to radioactivities of 17 - 2 923 Bq and different herbicide concentrations. For concentrations above 50 mg kg^-1, unlabelled test material (Pestanal, Riedel-de Haen, Seelze, Germany; > 99 %) was also added. All experiments were performed with herbicides amended in concentrations of 1.00, 100 and 10 000 mg kg^-1, except for one, where the herbicide mineralisation in aquifer sediment was tested with nine concentrations ranging from 0.10 to 10 000 mg kg^-1. Two sets of experiments were used to evaluate the effect of nutrient amendment on the herbicide mineralisation. In the first experiment, either 100 mg kg^-1 casamino acids (Difco Laboratories, Detroit, MI) or 5 % vol. of a minimal medium containing Cl-, PO4 2-, SO4 2-, Na+, K+, NH4+, Mg2+, Mn2+, Fe2+ and Zn2+ (de Lipthay et al., 1999) was applied. In the second experiment, four different amendments were tested: (i) 100 mg kg^-1 glucose, (ii) 100 mg kg^-1 catechol, (iii) 1.29 mM PO4 2- (high), or (iv) 0.13 mM PO4 2- (low) to flasks with aquifer sediment. Casamino acids and glucose was chosen as representatives of either a complex or a defined source of carbon. Catechol was included based on the hypothesis that it might stimulate the mineralisation of the test material as it is an intermediate in the degradation of aromatic compounds. The addition of the minimal medium was aimed at adding an array of different inorganic nutrients, and PO4 2- was added alone to test whether this nutrient specifically had an effect. Glass flasks were equipped with 5 mL test tubes containing 2 mL of 0.5 M NaOH to trap the 14CO2 produced from 14C-herbicide mineralisation. Triplicate flasks of each treatment were included. Abiotic controls were included with sediment and soil autoclaved for 3 x 20 min with 24 h between each cycle. Flasks were incubated in the dark at 10 °C. Selected herbicide concentrations were also tested at 20 °C. At each sampling the NaOH was changed in a laminar flow bench and the flasks were left open for 5 min in order to replenish oxygen. The NaOH was mixed with 10 mL of OptiPhase HiSafe scintillation fluid (Wallac, United Kingdom) and counted for 10 min on a Wallac 1409 liquid scintillation counter (LSC). The degradation kinetics was divided into either mineralisation without growth or growth-related mineralisation by plotting the mineralisation rate versus time on a semi-logarithmic scale as described by Reffstrup et al. (1998). If the slope of the line was zero- or first-order kinetics indicating mineralisation without growth. A slope with a value >0 was used as an indication of growth-related kinetics (Alexander, 1999; Reffstrup et al., 1998). The time needed for mineralisation of 50 % of 14C-labelled herbicides to 14CO2 (indicated in the text as the mineralisation half-life) was either calculated by fitting data to the respective kinetics (zero-order and first-order) or determined directly from the mineralisation curves in the case of growth-related mineralisation.
Distribution of 14C
At the termination of the mineralisation experiments the distribution of 14C into five fractions were estimated: (i) 14CO2, (ii) 14C-labelled microbial biomass, (iii) water-extractable 14C, (iv) base-extracted 14C, and (v) non-extractable 14C. The 14C mass balance was only determined for the mineralisation experiments with aquifer sediment added herbicide concentrations of 1, 100 and 10 000 mg kg^-1 without nutrient amendment. The water-, base- and non-extractable 14C were determined as described by Sørensen et al. (2006). The incorporation of 14C into biomass was determined by a modification of the chloroform fumigation method (Madsen and Kristensen, 1997; Jenkinson and Powlson, 1976). One millilitre of alcohol-free chloroform was added to 1 mL of groundwater in closed 20-mL glass vials and shaken at 200 rpm overnight to lyse 14C-labelled biomass. After evaporation of the chloroform, 1.0 g fresh sediment was added and a test tube with 1 mL of 0.5 M NaOH was inserted. The flasks were then incubated at 20 °C for 1 month. The 14C released from biomass and subsequently metabolised by the added sediment microorganisms were measured as NaOH-trapped 14CO2 as described previously. In the sediment fraction, the 14C incorporated into biomass was determined by a similar protocol.
Bacterial densities
Bacterial densities were estimated by analysing triplicate sub-samples of each sediment and soil. One gram of sediment or soil was diluted in 9.0 mL sterile NaCl (0.9 % w/v), vortexed for 1 min and 10-fold dilutions were prepared. The number of culturable heterotrophic bacteria was estimated by plating on water agar (15.0 g L^-1 Bitek agar [Difco] in Milli-Q water) and 1/10 strength LB agar medium (LB10) (Sambrook et al., 1989). All agar media were supplemented with nystatin (50 mg mL^-1 ) to prevent fungal growth. Each sub-sample was plated in triplicate and incubated at 20 °C for 7 days (LB10) or 21 days (water agar) before enumeration of colonies (CFU). The number of test material-degraders was estimated by a most-probable-number (MPN) technique relying on detection of herbicide mineralisation. Two millilitres of a minimal medium (de Lipthay et al., 1999) containing 2.5 mg L^-1 herbicide + 4.50 nCi [U-14C]herbicide was added to 20 mL glass vials. To trap the evolved 14CO2, the vials were equipped with test tubes containing 1 mL of 0.5 M NaOH. The MPN vials were then supplied with 200 mL aliquots of each dilution (10^-1 - 10^-7) and incubated at 20 °C for 3 months. The MPN estimation was performed in triplicates.
Herbicide sorption
Sorption of 100 mg kg^-1 test material was determined at 10 °C according to the OECD guidelines 106 (OECD, 1997). Five grams dry weight sediment or soil and 4.5 mL of 0.01 M CaCl2 was pre-equilibrated by rotation for 24 h in 10 mL glass tubes. Additionally, in selected experiments organic and inorganic nutrients were added to the CaCl2 equal to the concentrations used in the mineralisation experiments. After pre-equilibration, 14C-labelled (5 000 dpm) and unlabelled herbicides were added in a final 0.5 mL volume. The samples were then mixed by rotation for another 96 h and following centrifuged (15 min, 750 x g) 1 mL of the supernatants were counted by LSC. Two types of controls were included, one with sediment or soil in order to correct for background radioactivity and another with only herbicide in order to correct for sorption to the glass surface. Sorption was described by the linear equation Cs = Kd x Cw where Kd denotes the distribution coefficient, Cs the concentration of the compound sorbed to the soil, and Cw the aqueous equilibrium concentration of the compound. - Details on results (Batch equilibrium method):
- Effect of concentration on the herbicide mineralisation in aquifer samples
In sediment from Fladerne Bæk, mineralisation of 2,4-D showed growth-linked kinetics and mineralisation half-lives of 37 - 84 days for herbicide concentrations ranging from 0.50 to 10 000 mg kg^-1 were apparent. In contrast, the lowest 2,4-D concentration of 0.10 mg kg^-1 revealed a mineralisation half-life of approximately 300 days, which could be explained by zero-order kinetics without growth of the degradative population. Mineralisation of 2,4-D was also tested at 20 °C with herbicide concentrations of 1.00, 100 and 10 000 mg kg^-1. Increasing the temperature with 10 °C decreased the mineralisation half-life 1.2 - 3.0 times, and the highest effect was observed with the highest herbicide concentrations. Comparable mineralisation patterns were measured with the test material. Growth-linked mineralisation kinetics was observed for herbicide concentrations of 50 - 10 000 mg kg^-1, with mineralisation half-lives of approximately 266 - 700 days. Some of the highest herbicide concentrations may have inhibited the degradative activity, as longer lag phases were apparent here. Test material concentrations in the range of 0.10 - 10 mg kg^-1 could be described by zero-order kinetics with mineralisation half-lives of > 4 years. Mineralisation half-lives decreased by a factor of 2.2 (100 mg kg^-1) and 3.3 (10 000 mg kg^-1) when increasing the temperature to 20 °C. Within the experimental period of 196 days, minor amounts of 14C-labelled 2,4,5-T were mineralised to 14CO2. The radiochemical purity of the [ring-U-14C]2,4,5-T used as a tracer in the mineralisation experiments was 98 % and 2,4,5-T mineralisation could therefore only be accounted for with herbicide concentrations in the range of 1.00 - 100 mg kg^-1 . Only zero-order kinetics was detected, with mineralisation half-lives of approximately 4.3 - 11.7 years. Increasing the temperature with 10 °C caused an increase in mineralisation rates by 2.6 - 3.6 times. No mineralisation was detected in any of the sterile controls.
Effect of concentration on the herbicide mineralisation in soil samples
In the soil samples 1.00 mg kg-1 2,4-D was mineralised by first-order kinetics. This pattern was detected at 10 °C as well as 20 °C, with mineralisation half-lives of 486 and 343 days, respectively. For higher concentrations, growth-linked kinetics was observed with mineralisation half-lives at 10 °C of 30 days (10 000 mg kg^-1) and 142 days (100 mg kg^-1). Increasing the temperature to 20 °C decreased the mineralisation half-life with a factor of 2.0 - 2.8. First-order kinetics was observed for the test material concentrations of 1.00 and 100 mg kg^-1. At 10 °C, mineralisation half-lives were 662 days (1.00 mg kg^-1) and 191 days (100 mg kg^-1, and these decreased by a factor of 2.9 and 1.7, respectively, when increasing the temperature to 20 °C. Growth-related mineralisation kinetics was apparent with a test material concentration of 10 000 mg kg^-1, giving mineralisation half-lives of 78 days at 10 °C and 33 days at 20 °C. No mineralisation was detected in any of the sterile controls.
Effect of nutrient amendments on the herbicide mineralisation in aquifer samples
Amendments of casamino acids and mineral medium imposed different effects on the mineralisation of phenoxyalkanoic acids depending on the herbicide concentration. No significant effects on the mineralisation rate were detected with the lowest test material concentration of 1 mg kg^-1 (P > 0.05). For herbicide concentrations of 100 mg kg^-1 minor but significant (P < 0.05) effects were observed resulting in increased lag periods before accelerated mineralisation with casamino acids and decreased lag periods following amendments with minimal medium. With the highest herbicide concentration (10 000 mg kg^-1), the amendment of both minimal medium and casamino acids resulted in decreased lag periods of approximately 30 days for the test material.
In the second experimental set-up, the authors examined the effect of addition of glucose or catechol as well as two concentrations of phosphate on the mineralisation of the test material. With herbicide concentrations of 1.00 mg kg^-1, addition of glucose and high phosphate concentrations significantly (P < 0.05) increased the mineralisation extent within the experiment. In contrast, catechol had a negative effect (P < 0.05) on the amount of 14C-labelled herbicide mineralised to 14CO2, while the amendment of low phosphate concentrations had no effect. The addition of glucose and catechol resulted in prolonged lag phases for the mineralisation of 100 mg kg-1 2,4-D, but phosphate amendment had no effect. Similar results were obtained with the test material. For the highest herbicide concentration of 10 000 mg kg^-1, addition of glucose and catechol extended the length of the lag phase by 150 days before initiation of test material mineralisation. With the test material, only the high phosphate concentration decreased the lag phase before mineralisation. Double S-shaped curves for the growth-linked mineralisation of the test material were apparent in both aquifer and soil samples with and without nutrient amendments
Distribution of 14C following herbicide mineralisation
The authors recovered 82 – 120 % of initially added 14C-labelled herbicides following the mineralisation experiments. After the 2,4-D mineralisation experiments, 14CO2 constituted the largest fraction (70 – 78 %). The biomass fraction accounted for 2 – 17 %, while 2.9 - 5.2 % was extracted with NaOH. Minor amounts (0.5 - 4.8 %) were found in the water-extractable fraction and only < 0.1 % remained as non-extractable residues detected by incineration. With the test material, the largest part of 14C was found in the water fraction (25 – 67 %) and as 14CO2 (11 – 49 %). A substantial part was extracted with NaOH (15 – 20 %) and 5.6 – 20 % was found in the biomass fraction. Again, < 0.1 % was recovered from incineration.
Densities of different bacterial populations
The heterotrophic bacterial density was 10^6 - 10^7 CFU g-1. No significant differences were found between sediment and soil (P > 0.05). On the contrary, significantly higher numbers of phenoxy acid degraders were measured in soil compared to sediment. The effect of herbicide-exposure on bacterial density was determined after termination of the mineralisation experiments. In contrast, significantly higher numbers of test material degraders were found in aquifer sediment and surface soil amended with 10 000 mg kg^-1 herbicide corresponding to the detection of growth-linked mineralisation. In sediment, there was also a tendency of increased numbers of test material- degraders for experiments amended with 100 mg kg^-1 herbicide. Nutrient amendments significantly (P < 0.05) increased the general heterotrophic bacterial density with a factor of 5 - 10). However, no effect on the density of specific phenoxy acid degraders was detected compared to un-amended samples.
Herbicide sorption
All three herbicides showed significantly higher sorption to soil compared to aquifer sediment. With sediment, Kd values of 0.113 - 0.296 L kg^-1 were measured which is 12 - 68 times lower than values detected with soil. With sediment, the test material had the highest Kd value. In contrast, the test material had the lowest Kd in sorption experiments with soil. Presence of nutrients had a significant impact on the sorption of the test material to aquifer sediment (P < 0.05). Sorption of the test material was differently affected and Kd values solely increased by the amendment of catechol. Glucose had no effect while all other nutrients resulted in decreased sorption. - Validity criteria fulfilled:
- not applicable
- Conclusions:
- The impact of the herbicide concentration (0.10 -10 000 mg kg^-1) and addition of organic and inorganic nutrients on the test material mineralisation in aquifer and soil samples was studied in laboratory experiments. A shift from non-growth to growth-linked mineralisation kinetics was observed in aquifer sediment with test material concentrations >10.0 mg kg^-1. The shift was apparent at higher herbicide concentrations in soil coinciding with a lower bioavailable fraction and a higher herbicide sorption to soil. Herbicide addition did not affect the bacterial density, although test material applied at 10 000 mg kg^-1 stimulated growth of specific degraders. Generally, nutrient amendments did not stimulate mineralisation at the lowest herbicide concentrations. In contrast, the mineralisation rate of higher herbicide concentrations was significantly stimulated by the amendment of inorganic nutrients.
- Executive summary:
Under the conditions of the study, the test material was mineralised in the tested samples of aquifer sediment and soil, but the rate and extent were highly influenced by herbicide structure, incubation temperature, herbicide concentration and availability of additional nutrients. Shifts from non-growth-linked to growth-linked mineralisation kinetics were detected at different herbicide concentrations depending on the type of herbicide and the origin of the sample. The higher concentration thresholds in the soil samples compared to aquifer samples were probably due to higher sorption. Furthermore, the higher density of indigenous herbicide degraders in soil compared to the aquifer sediment is very likely a key factor. Attempts to stimulate indigenous degraders by addition of organic and inorganic nutrients suggested that the mineralisation of phenoxyalkanoic acid herbicides in soil and aquifer sediment contaminated with high concentrations may benefit from the amendment of inorganic nutrients. In contrast minor mineralisation was apparent with low herbicide concentrations and no stimulation was possible by supplying either organic or inorganic nutrients.
- Endpoint:
- adsorption / desorption, other
- Type of information:
- experimental study
- Adequacy of study:
- key study
- Reliability:
- 2 (reliable with restrictions)
- Rationale for reliability incl. deficiencies:
- comparable to guideline study with acceptable restrictions
- Reason / purpose for cross-reference:
- reference to same study
- Qualifier:
- according to guideline
- Guideline:
- other: OECD Test guideline 'Adsorption-Desorption'.
- Deviations:
- not specified
- GLP compliance:
- no
- Type of method:
- other: Not specified
- Media:
- soil
- Radiolabelling:
- yes
- Test temperature:
- 20 °C
- Analytical monitoring:
- no
- Matrix no.:
- #1
- Matrix type:
- sandy loam
- % Clay:
- 15.8
- % Silt:
- 18.4
- % Sand:
- 63.4
- pH:
- 6.9
- Matrix no.:
- #2
- Matrix type:
- sandy loam
- % Clay:
- 13.2
- % Silt:
- 28.6
- % Sand:
- 55.5
- pH:
- 6.7
- Matrix no.:
- #3
- Matrix type:
- other: Coarse sandy soil: 0 - 25 cm depth
- % Clay:
- 4
- % Silt:
- 6.6
- % Sand:
- 86.9
- pH:
- 6.6
- Details on matrix:
- COLLECTION AND STORAGE
- Geographic location: Soils were from three Danish Experimental Farms (#1 Flakkebjerg, #2 Roskilde and #3 Jyndevad, providing sandy loam, sandy loam and coarse sandy soil, respectively). The soils were grown with rotational crops including cereals.
- Sampling depth: Subsoil was from undisturbed cores of coarse sandy soil sampled 33 - 66 and 66 - 99 cm below soil surface (Jyndevad). Adsorption coefficients were calculated for the soil at a depth of 0 - 25 cm. The depth for both Flakkebjerg and Roskilde was 0 - 30 cm
- Storage conditions: Stored at 5 °C with about 25 % of total water holding capacity (WHC) until use. Subsoil was kept in stainless steel tubes at 5 °C.
- Storage length: Storage for less than one month.
- Soil preparation: After sampling, the surface soils were sieved (< 2 mm).
PROPERTIES OF THE SOILS (in addition to defined fields)
- WHC (Water holding capacity, % of wet soil):
Flakkebjerg: 31
Roskilde: 31
Jyndevad: 29
- Humus (%C x 1.72):
Flakkebjerg: 2.4
Roskilde: 2.6
Jyndevad: 2.4 - Details on test conditions:
- Adsorption (Kd) of the test material was determined by shaking 5 g of soil with 25 mL 0.01 M CaCl2 added to 14C-test material (5 mg L^-1) for 16 h. To avoid degradation the soils were sterilised by irradiation. The soil was precipitated by centrifugation and 14C was counted in aliquots of the supernatant to calculate concentrations of the test material in soil and water. Koc was calculated from Kd and the organic C contents of the soils.
- Sample No.:
- #1
- Duration:
- 16 h
- Initial conc. measured:
- 5 other: mg L^-1
- pH:
- 6.9
- Temp.:
- 20 °C
- Sample No.:
- #2
- Duration:
- 16 h
- Initial conc. measured:
- 5 other: mg L^-1
- pH:
- 6.7
- Temp.:
- 20 °C
- Sample No.:
- #3
- Duration:
- 16 h
- Initial conc. measured:
- 5 other: mg L^-1
- pH:
- 6.6
- Temp.:
- 20 °C
- Sample No.:
- #1
- Type:
- Kd
- Value:
- 0.12 dimensionless
- pH:
- 6.9
- Temp.:
- 20 °C
- Matrix:
- Flakkebjerg soil 0 - 30 cm depth
- % Org. carbon:
- 2.4
- Sample No.:
- #2
- Type:
- Kd
- Value:
- 0.2 dimensionless
- pH:
- 6.7
- Temp.:
- 20 °C
- Matrix:
- Roskilde soil 0 - 30 cm depth
- % Org. carbon:
- 2.6
- Sample No.:
- #3
- Type:
- Kd
- Value:
- 0.07 dimensionless
- pH:
- 6.6
- Temp.:
- 20 °C
- Matrix:
- Jyndevad soil 0 - 25 cm depth
- % Org. carbon:
- 2.4
- Sample No.:
- #1
- Type:
- Koc
- Value:
- 8.4 dimensionless
- pH:
- 6.9
- Temp.:
- 20 °C
- Matrix:
- Flakkebjerg soil 0 - 30 cm depth
- % Org. carbon:
- 2.4
- Sample No.:
- #2
- Type:
- Koc
- Value:
- 13.3 dimensionless
- pH:
- 6.7
- Temp.:
- 20 °C
- Matrix:
- Roskilde soil 0 - 30 cm depth
- % Org. carbon:
- 2.6
- Sample No.:
- #3
- Type:
- Koc
- Value:
- 5.3 dimensionless
- pH:
- 6.6
- Temp.:
- 20 °C
- Matrix:
- Jyndevad soil 0 - 25 cm depth
- % Org. carbon:
- 2.4
- Sample No.:
- #1
- Remarks on result:
- not measured/tested
- Adsorption and desorption constants:
- The adsorption is low with Kd values between 0.07 and 0.2 and Koc values of 8, 13 and 5, respectively in the three soils. With such low adsorption values the test material is likely to leach if not decomposed in the surface soil.
- Transformation products:
- not measured
- Validity criteria fulfilled:
- not specified
- Conclusions:
- Under the conditions of the study the adsorption of the test material was low with Kd values of 0.07 - 0.2 and Koc values of 8.4, 13.3 and 5.3 in the three soil types.
- Executive summary:
Adsorption of 14C-ring-labelled test material was determined according to the OECD Test Guideline Adsorption - Desorption in three different soil types.
Soils were from three Danish Experimental Farms (#1 Flakkebjerg, #2 Roskilde and #3 Jyndevad, providing sandy loam, sandy loam and coarse sandy soil, respectively).
Adsorption (Kd) of the test material was determined at 20 °C by shaking 5 g of soil with 25 mL 0.01 M CaCl2 added to 14C-test material (5 mg L^-1) for 16 h. To avoid degradation the soils were sterilised by irradiation. The soil was precipitated by centrifugation and 14C was counted in aliquots of the supernatant to calculate concentrations of the test material in soil and water. Koc was calculated from Kd and the organic C contents of the soils.
The adsorption of the test material was low with Kd values of 0.07 - 0.2 and Koc values of 8.4, 13.3 and 5.3 in the three soil types (Flakkebjerg, Roskilde and Jyndevad, respectively). With such low adsorption values the test material is likely to leach if not decomposed in the surface soil.
- Endpoint:
- adsorption / desorption, other
- Type of information:
- experimental study
- Adequacy of study:
- supporting study
- Reliability:
- 2 (reliable with restrictions)
- Rationale for reliability incl. deficiencies:
- study well documented, meets generally accepted scientific principles, acceptable for assessment
- Qualifier:
- no guideline followed
- Principles of method if other than guideline:
- The study reports the results of Koc and soil DT50 experiments used for the definition of the leaching potential through the GUS Index.
- GLP compliance:
- not specified
- Remarks:
- Published literature
- Type of method:
- HPLC estimation method
- Details on study design: HPLC method:
- DETERMINATION OF DT50
- The degradation experiments were conducted on fresh soil, not previously treated with pesticides which was collected in an agricultural field representative for corn production of the province of Bergamo (Lombardia Region, Italy). This soil type was usually employed in other degradation experiments conducted in the testing laboratory.
- The degradation experiment was performed on two soil replicates (each in duplicate).
- The application dose was 5 mg/kg.
- An initial recovery of the test material of 70 % from soil was extracted.
- The HPLC conditions were: Acetonitrile-water 50:50.
- The degradation behaviour of the test material was represented by first order kinetics (0.99 < r^2 < 0.94).
- The soil DT50 values were calculated with the following equation:
DT50 = (ln 2) / m
Where:
m represents the slope of the degradation lines.
- The abundance of the soil microbial community, which was quantified throughout the degradation experiments by epifluorescence direct count in order to assess the soil viability, was not affected by the presence of pesticide metabolites with respect to the original fresh soil.
- Experimental Conditions for Soil DT50:
Incubation temperature: 21 °C
Length of test: Up to DT90
Reference compound: Atrazine
Extraction solvent: CH3CHO
Analytical equipment: RP-HPLC/DAD
Wavelength (nm): 205 - 210 nm
Column: 25 cm length x Ø 4.6 mm, 5 µm particle size RP-amide C16
Flow rate: 1 mL/min
Mobile phase: CH3CN/H2O
DETERMINATION OF KOC
- The Koc values of metabolites were determined by HPLC screening (OECD). The experimental capacity factors (k’) of seven reference compounds were plotted against their corresponding Koc official values. The resulting regression line (r^2 = 0.88 was: log Koc = 8.8697 log k’ + 1.0701. This equation was used to calculate the Koc value for the test material by substituting the experimental k’.
- Experimental Conditions for Koc:
Analytical equipment: RP-HPLC/DAD
Wavelength: 210 nm
Column: Supelcosil LC-CN
Mobile phase: CH3OH/H2O (55:45)
Elution: isocratic
Reference compounds: acetanilide, atrazine, dichlofop-methyl, isoproturon, linuron, methiocarb, phenol
DETERMINATION OF GUS INDEX
- The GUS index was calculated with the following equation:
GUS = log (soil DT50) x (4 – log Koc)
By substituting the correspondent experimental values of soil DT50 and Koc. In accordance with this classification, a GUS >2.8 shows the substance to be a potential leacher (L), those with GUS between 1.8 and 2.8 have transient properties (T) and those with GUS <1.8 are non-leachers (NL). - Matrix no.:
- #1
- Matrix type:
- other: Soil used for DT50 experiment
- % Clay:
- 11.5
- % Silt:
- 47
- % Sand:
- 41.5
- % Org. carbon:
- 1.4
- pH:
- 8
- CEC:
- 14.95 meq/100 g soil d.w.
- Details on matrix:
- PROPERTIES
- Soil taxonomic classification: USDA F
- Moisture content: 22 %
- Water holding capacity max: 34 % - Type:
- Koc
- Value:
- 93 dimensionless
- Details on results (HPLC method):
- The degradation cureves versus time of the examined metabolites fitted with first order kinetics.
Soil DT50 was < 3 days for the test material. The DT50 was determined from two defined soil replicates, each in duplicate.
- Koc ± S.D. from three HPLC determinations = 93 ± 1
- DT50 (days) ± S.D. = 0.4 ± 0.1
- GUS = -0.88
- The test material is a non-leacher. - Transformation products:
- not measured
- Validity criteria fulfilled:
- not applicable
- Conclusions:
- Under the conditions of this study the Koc was 93 ± 1 and the DT50 was 0.4 days. The test material was considered a non-leacher.
- Executive summary:
The study investigated the leaching potential of the test material using the results of Koc and soil DT50 experiments through the GUS Index.
From the degradation curve versus time of the examined metabolite fitted with first order kinetics the test material degraded very quickly (0.4 days). The Koc of the test material was 93 and the GUS value calculated from the equation was -0.88. The test material had a Koc value which was typical of a mobile compound.
Under the conditions of this study the Koc was 93 ± 1 and the DT50 was 0.4 days. The test material was considered a non-leacher.
- Endpoint:
- adsorption / desorption, other
- Type of information:
- read-across from supporting substance (structural analogue or surrogate)
- Adequacy of study:
- supporting study
- Justification for type of information:
- Read-across to structurally similar supporting substance.
- Reason / purpose for cross-reference:
- read-across source
- Type:
- Koc
- Value:
- 93 dimensionless
- Details on results (HPLC method):
- The degradation cureves versus time of the examined metabolites fitted with first order kinetics.
Soil DT50 was < 3 days for the test material. The DT50 was determined from two defined soil replicates, each in duplicate.
- Koc ± S.D. from three HPLC determinations = 93 ± 1
- DT50 (days) ± S.D. = 0.4 ± 0.1
- GUS = -0.88
- The test material is a non-leacher. - Endpoint:
- adsorption / desorption: screening
- Type of information:
- experimental study
- Adequacy of study:
- supporting study
- Study period:
- 25 June 1986 to 01 July 1986
- Reliability:
- 2 (reliable with restrictions)
- Rationale for reliability incl. deficiencies:
- study well documented, meets generally accepted scientific principles, acceptable for assessment
- Qualifier:
- according to guideline
- Guideline:
- other: Pesticide Assessment Guidelines of the Environmental Protection Agency (EPA), Subdivision N, Section 163-1.
- Deviations:
- no
- GLP compliance:
- not specified
- Type of method:
- batch equilibrium method
- Media:
- soil
- Radiolabelling:
- yes
- Test temperature:
- 25 °C
- Matrix no.:
- #1
- Matrix type:
- sand
- % Clay:
- 2
- % Silt:
- 8
- % Sand:
- 90
- % Org. carbon:
- 0.8
- pH:
- 5.6
- CEC:
- 1 meq/100 g soil d.w.
- Bulk density (g/cm³):
- 1.62
- Matrix no.:
- #2
- Matrix type:
- sandy loam
- % Clay:
- 10
- % Silt:
- 34
- % Sand:
- 56
- % Org. carbon:
- 2.3
- pH:
- 7.6
- CEC:
- 9 meq/100 g soil d.w.
- Bulk density (g/cm³):
- 1.22
- Matrix no.:
- #3
- Matrix type:
- silt loam
- % Clay:
- 20
- % Silt:
- 74
- % Sand:
- 6
- % Org. carbon:
- 5.9
- pH:
- 6.8
- CEC:
- 13 meq/100 g soil d.w.
- Bulk density (g/cm³):
- 1.27
- Matrix no.:
- #4
- Matrix type:
- silty clay loam
- % Clay:
- 34
- % Silt:
- 42
- % Sand:
- 24
- % Org. carbon:
- 2.5
- pH:
- 6.6
- CEC:
- 14.7 meq/100 g soil d.w.
- Bulk density (g/cm³):
- 1.21
- Details on matrix:
- - The soils used in the study were chosen because they have diverse physical characteristics. The four soils used were Plainfield sand (#1), Fox sandy loam (#2), Plano silt loam (#3), and Hagerstown silty clay loam (#4).
- Each soil was oven dried at 90 °C overnight and sieved through a 2-mm screen.
- Fox sandy loam is from a farm in Dane County, Wisconsin. Plainfield sand was collected near the south shore of Petenwell Lake in Adams County, Wisconsin. Plano silt loam was collected at the University of Wisconsin's·Arlington farm, Columbia County, Wisconsin. The Hagerstown soil is from near Lime Kiln in Frederick County, Maryland. - Details on test conditions:
- TEST EQUIPMENT
- Oubnoff metabolic incubating shaker, GCA Precision Scientific
- Pyrex disposable screw-top culture tubes, 20 mm x 150 mm, with Teflon-lined caps
- TLC linear analyer, Model LB2842, Berthold
- Scintillation spectrometers, Packard 4640 and 460 CD
- Precoated TLC plates, 0.25 mm x 5 mm x 20 mm, Silica Gel 60 F-254
- Solvent used in the study was 0.01 M calcium nitrate
PROCEDURES
Preliminary Studies
- Before definitive study initiation, preliminary studies were undertaken to confirm the solubility of the test material, to determine its adsorption to glassware, and to evaluate its stability in solution under the conditions proposed for the study. A preliminary adsorption study was also carried out to select an appropriate water/soil ratio and equilibrium time for the definitive study.
- Preparation of Solutions: An aqueous stock solution of the test material was prepared for all of the studies by combining C-labelled test material and nonradioactive test material. This solution contained 113 ppm test material in 0.1 M calcium nitrate. The specific activity (A) of the isotopically diluted test material was calculated, to be 3 640 dpm/µg.
- A dilution of this solution in water (1:10) was used as the high concentration solution in the preliminary studies. The concentration of this solution was determined by liquid scintillation counting (LSC) to be 11.6 ppm test material. This high concentration solution was further diluted with 0.01 M calcium nitrate to provide the various other solutions used in these studies.
- Solubility: Solubility of the test material was verified by diluting aliquots of the 11.6 ppm solution with 0.01 M calcium nitrate to yield concentrations of 5.82, 1.16, and 0.582 ppm test material based on the dilution volumes. Duplicate aliquots of each solution were then counted by LSC.
- Adsorption to Glass: Two culture tubes, each containing 10 mL of the 0.582 ppm test material solution but containing no soil, were shaken in the Dubnoff incubator at 25 °C for 24 hours. From each test tube, duplicate 100 µL aliquots were taken for radiocarbon level determination by LSC.
- Equilibrium. For each soil, two culture tubes, each containing approximately 1 g of soil and 10 mL of the 11.6 ppm test material solution, were capped, placed in the incubator, and shaken for their designated time (1, 2, 5, or 24 hours). At each designated time, the culture tubes were removed from the incubator and centrifuged until the soil was completely packed at the bottom of the culture tubes. Duplicate 100 µL aliquots were removed for LSC to detect a possible decrease in the concentration of test material in solution. Two culture tubes, each containing 10 mL of water at 25 °C, were centrifuged for the same time and at the same rotor speed as the samples. The temperature of the water was then determined to be 25 °C, demonstrating that the centrifugation process caused neither heating nor cooling of the samples.
- Stability: Stability of the test material was confirmed by TLC analysis of the 113 ppm stock solution of aqueous radiolabelled test material at the beginning of the studies and after it had been standing in room light and at room temperature for 6 days.
DEFINITIVE STUDY
- A fresh 1:10 dilution was prepared similarly for the definitive study; the concentration of this solution was determined by LSC to be 12.0 ppm test material. The radioactivity of this solution and its three dilutions was determined by duplicate LSC analysis.
- Adsorption: Duplicate soil-solution samples were prepared for each of the four soil types specified in the protocol at each of four concentrations (0.606, 1.24, 5.92, and 12.0 ppm as determined by LSC) by adding 10 mL of the test material solutions to 3 g aliquots of the previously dried and sieved soils. Thus, a total of 32 culture tubes were prepared. The culture tubes were capped, shaken vigorously, and placed in the Dubnoff metabolic incubating shaker. After 1 hour of equilibration at 25 °C and after approximately 10 minutes of centrifugation or until the soil was packed at the bottom of the culture tubes, duplicate 1 mL aliquots were removed from each culture tube, and the radiocarbon level was determined by LSC.
- Desorption: After removing an additional 5 mL of solution, 7 mL of 0.01 M calcium nitrate solution was added to each culture tube to re-establish a 10 mL volume. The culture tubes were shaken vigorously on a vortex to break up the soil packed on the bottom. Following 1 hour of shaking at 25 °C, the tubes were centrifuged to pack the soil at the bottom of the culture tubes, duplicate 1 mL aliquots were removed, and the radiocarbon levels were determined by LSC. - Sample No.:
- #1
- Type:
- Kd
- Value:
- 0.199 dimensionless
- Matrix:
- Plainfiled Sand
- Sample No.:
- #2
- Type:
- Kd
- Value:
- 0.298 dimensionless
- Matrix:
- Fox Sandy Loam
- Sample No.:
- #3
- Type:
- Kd
- Value:
- 0.686 dimensionless
- Matrix:
- Plano Silt Loam
- Sample No.:
- #4
- Type:
- Kd
- Value:
- 0.428 dimensionless
- Matrix:
- Hagerstown Silty Clay Loam
- Adsorption and desorption constants:
- PRELIMINARY STUDIES
- Solubility: On the basis of LSC analyses of the 11.6 ppm test material stock solution and a series of dilutions over a 20-fold range of that solution, solubility was found to be constant. That is, the correlation between the concentrations as determined by the dilution volumes and the radioactivity as determined by LSC was 0.999994.
- Adsorption to Containers: Adsorption of aqueous test material to the culture tubes was negligible.
- Equilibrium Time and Approximate Equilibrium Constant: The change in concentration of an aqueous test material solution after various equilibration times was determined for each soil. Adsorption equilibrium for all soils was reached in essentially 1 hour because no consistent change was observed in the solution concentrations between a 1- and a 24-hour equilibration time. Based on these results, a 1-hour equilibration time was chosen. A soil: water ratio of 3:10 was selected for all the soils because very little test material was adsorbed by any of the soils in the preliminary study.
- Stability: TLC analysis indicated that no degradation occurred within this time period, which exceeded the time required to complete the definitive study.
DEFINITIVE STUDY
- The amount of test material desorbed was usually in the range of 25 to 50 % of the amount absorbed. The amount of test material desorbed showed some suspect values due to the small amount of test material adsorbed by the soils which made it difficult to accurately measure the amount desorbed. - Transformation products:
- not measured
- Validity criteria fulfilled:
- not applicable
- Conclusions:
- Aqueous test material adsorbed to some degree in all soils studied. At 25 °C, this adsorption followed a Freundlich isotherm. Freundlich constant (Kd) values ranged from 0.20 to 0.69. Desorption of adsorbed test material was usually in the range of 25 to 50 % of the amount adsorbed, with some scattered values due to the small amount of test material adsorbed by the soil. Under the conditions of this study: Kd (Fox - sandy loam) = 0.298, Kd (Plano - silt loam) = 0.686, Kd (Hagerstown silty - clay loam) = 0.428 and Kd (Plainfiled sand) = 0.199.
- Executive summary:
The adsorption/desorption of the test material on representative agricultural soils was investigated in a study according to Pesticide Assessment Guidelines of the Environmental Protection Agency (EPA), Subdivision N, Section 163-1.
Four diverse soils were each equilibrated with aqueous solutions of the test material. The resulting equilibrium concentration of the test material in water was measured, and the soil equilibrium concentration was calculated. Four aqueous concentrations were used to construct a Freundlich isotherm of adsorption at 25 °C for each soil.
Aqueous test material adsorbed to some degree in all soils studied. At 25 °C, this adsorption followed a Freundlich isotherm. Freundlich constant (Kd) values ranged from 0.20 to 0.69. Desorption of adsorbed test material was usually in the range of 25 to 50 % of the amount adsorbed, with some scattered values due to the small amount of test material adsorbed by the soil.
Under the conditions of this study: Kd (Fox - sandy loam) = 0.298, Kd (Plano - silt loam) = 0.686, Kd (Hagerstown silty - clay loam) = 0.428 and Kd (Plainfiled sand) = 0.199.
- Endpoint:
- adsorption / desorption: screening
- Type of information:
- experimental study
- Adequacy of study:
- supporting study
- Study period:
- 13 May 1986 to 16 June 1986
- Reliability:
- 2 (reliable with restrictions)
- Rationale for reliability incl. deficiencies:
- study well documented, meets generally accepted scientific principles, acceptable for assessment
- Qualifier:
- according to guideline
- Guideline:
- other: Section 163-1, Subdivision N of Environmental Protection Agency (EPA) Pesticide Assessment Guidelines.
- Deviations:
- no
- GLP compliance:
- not specified
- Type of method:
- other: soil mobility study
- Media:
- soil
- Radiolabelling:
- yes
- Remarks:
- It was labelled with a specific activity of 39 mCi/mmol.
- Matrix no.:
- #1
- Matrix type:
- sandy loam
- % Clay:
- 10
- % Silt:
- 34
- % Sand:
- 56
- % Org. carbon:
- 2.3
- pH:
- 7.6
- CEC:
- 9 meq/100 g soil d.w.
- Details on matrix:
- COLLECTION AND STORAGE
- Geographic location: A farm in De Forest in Dane County, Wisconsin.
PROPERTIES
- The field moisture capacity of the Fox soil was determined to be 15.2 %. - Details on test conditions:
- MATERIALS AND EQUIPMENT
- All water used was processed through a Milli-Q water purification system, Millipore Corporation.
- The soil used in this study was Fox sandy loam. The physical properties of the soil were determined by the University of Wisconsin-Extension Soil and Forage Laboratory. The microbial characterisation and field moisture capacity (FMC) were performed by HLA.
- Biometer flask, No. 2556, Bellco Glass, Inc.
- TLC linear analyser, Model LB 2842, Berthold.
- 14C-2,4-D and 14C-Paraquat reference standards were supplied by HLA.
- Glass plate, 20 cm x 20 cm.
EXPERIMENTAL PROCEDURES
- FMC: Soil FMC was determined by saturating a portion of soil with water in a Buchner funnel, attaching the funnel to a vacuum filtration flask, and drawing a pressure of 0.3 atm. The wet soft was then weighed, dried in a 103 °C oven for 24 hours, and weighed again. Weight loss divided by dry weight is equal to the FMC.
- Soil Fortification: Because the soil moisture was approximately 7 %, no additional air-drying was necessary before the soil was sieved through a 2-mm mesh screen to ensure uniformity. A 50.0 g sample of sieved soft (46.5 g on a dry-soil basis) was placed in a biometer flask. An acetone solution was prepared that contained radiolabelled and non-radiolabelled test material. The soil was fortified by adding 0.44 mL of the acetone solution (a soil: solution ratio of 106:1), which contained 6.28 x 10^7 dpm (156 µg) of radiolabelled test material and 345 µg of non-radiolabelled test material for a total of 501 µg test material. This gave a concentration of 10.8 ppm test material in soil (dry-weight basis). The solvent was evaporated with a stream of nitrogen over the soil. After the solvent had evaporated, the soil was stirred and mixed with a spatula in the flask for approximately 10 minutes.
- Aerobic Incubation: Water was added to the fortified soil to bring the moisture content to 78 % of the FMC. Approximately 10 mL of the 10 % sodium hydroxide (NaOH) solution was added to the side arm of the biometer flask. The flask was sealed with a rubber stopper and incubated in a dark environment at 25 ± 1 °C. At Days 6, 20. and 30, the NaOH trap was assayed for radioactivity.
- Radio analysis of the NaOH Trap: The radioactivity of the NaOH solution was determined by removing duplicate aliquots from the trap and measuring activity via liquid scintillation counting. The trap was rinsed, and fresh NaOH solution was placed in the side-arm trap at every sampling point. Activity found in the NaOH trap was indicative of 14C-test material being metabolised.
- TLC Plate Preparation: Soil was sieved through a 1.18-mm screen. A tape strip (approximately 2.5 cm wide) was placed approximately 17.5 cm from one edge of the 20 x 20 cm glass plate to create an area approximately 17.5 x 20.0 cm. A slurry of soil and water was prepared, coated on a 20 x 17.5 cm area of the plate, and dried. Channels were cut in the soil to separate the plate into seven strips, each approximately 17.5 cm long and 2.5 cm wide.
- Sample Analysis: A zone of soil was scraped from the origin of three of the soil TLC plate strips and replaced by a subsample of the 14C-test material-fortified soil that had been aged 30 days. To the soil subsample, a small quantity of water was added to form a uniform slurry. The approximate quantity of 14C applied to each soil TLC strip as aged 14C-test material-fortified soil was 0.13 μCi. Of the remaining four strips, one was spotted with a toluene solution of 14C-2,4-D, one with a methanol solution of 14C-Paraquat, and the last two with an acetone solution of nonsoil-aged test material. The approximate quantity of 14C applied to the remaining four strips was 0.02 to 0.03 μCi. After the solvent had evaporated and the soil slurry had dried, the soil TLC plate was developed in an unsaturated tank of water at room temperature until the solvent front reached the top of one of the soil strips. The soil plate was air-dried, and the leading edge of radioactivity in each strip was determined using the linear analyser. From the linear analyser scans, relative mobility (frontal Rf) values were calculated by measuring the distance from the origin to the leading edge of radioactivity and dividing by the distance from the origin to the solvent front. A sorption coefficient, as required by Subdivision N, was calculated. - Transformation products:
- not measured
- Details on results (Batch equilibrium method):
- RESULTS AND DISCUSSION
- By Day 30, 39.3 % of the applied activity had been trapped in NaOH during aerobic incubation of 14C-test material in Fox sandy loam soil.
- Triplicate mobility determinations were conducted for the soil-aged test material, while nonsoil-aged test material determinations were done in duplicate. Reference standards of 2,4-D and Paraquat were single determinations.
- Most of the applied radioactivity was detected at the origin for soil-aged residues of the test material, while nonsoil-aged test material migrated almost with the solvent front. From the linear analyser scans of the soil-aged test material, only a slight amount of the radioactivity was detected near the Rf as for nonsoil-aged test material.
- The frontal Rf values, mobility classes, and sorption coefficients for each pesticide were determined. - Validity criteria fulfilled:
- not applicable
- Conclusions:
- During aerobic incubation of 14C-test material in Fox sandy loam soil, approximately 39 % of the applied radioactivity was trapped in NaOH by Day 30. The soil-aged residues of the test material were much less mobile (lower Rf value) on soil TLC than nonsoil-aged test material. Soil-aged test material and 14C-Paraquat were classified as immobile, while 14C-2,4-D was classified as having an intermediate mobility and nonsoil-aged test material was classified as mobile. Since little mobility of soil-aged test material was observed on a sandy loam soil, no other soil types were tested.
- Executive summary:
During aerobic incubation of 14C-test material in Fox sandy loam soil, approximately 39 % of the applied radioactivity was trapped in NaOH by Day 30. A subsample of the soil that contained the aged test material residues was then used in a soil mobility study.
A 20 x 20 cm glass plate was coated with a thin layer of Fox sandy loam soil. Radiolabelled, soil-aged test material; nonsoil-aged test material; 2,4-D; and Paraquat were each applied to the soil, and water was allowed to migrate up the plate. The distribution of radioactivity for each sample was then determined with a linear analyser. The frontal relative migration (Rf) value of each was measured, the mobility class was determined, and the sorption coefficient was calculated.
Soil-aged residues of the test material were much less mobile (lower Rf value) on soft thin layer chromatography than non-soil ages test material.
- Endpoint:
- adsorption / desorption: screening
- Type of information:
- experimental study
- Adequacy of study:
- supporting study
- Reliability:
- 2 (reliable with restrictions)
- Rationale for reliability incl. deficiencies:
- study well documented, meets generally accepted scientific principles, acceptable for assessment
- Qualifier:
- equivalent or similar to guideline
- Guideline:
- OECD Guideline 106 (Adsorption - Desorption Using a Batch Equilibrium Method)
- Principles of method if other than guideline:
- Literature review of phenoxyalkanoic acid herbicides to compare the extent of their adsorption in soils and degradation rates to assess their potential for groundwater contamination.
- GLP compliance:
- not specified
- Remarks:
- Published literature.
- Radiolabelling:
- not specified
- Type:
- Kd
- Value:
- 0.48 other: mL/g
- pH:
- 7
- % Org. carbon:
- 1.4
- Remarks on result:
- other: (0.34-0.63)
- Type:
- Koc
- Value:
- 35 other: mL/g
- pH:
- 7
- % Org. carbon:
- 1.4
- Remarks on result:
- other: (24-45)
- Type:
- other: DT50
- Value:
- 8.3 other: days
- Validity criteria fulfilled:
- not applicable
- Conclusions:
- For the test material the following values were reported, Kd = 0.48 mL/g, Koc = 35 mL/g and DT50 = 8.3 days.
- Executive summary:
A literature review of phenoxyalkanoic acid herbicides was performed to compare the extent of their adsorption in soils and degradation rates to assess their potential for groundwater contamination.
Comparison of published data concerning the adsorption of phenoxyalkanoic acid herbicides in soils found that, the adsorption of the test matreial is significantly inversely correlated with the logD values. Modeling of their adsorption, based on the negative relationship between adsorption of anions on organic matter and log D values, is possible.
Microbial degradation is the major process that controls the rate of the disappearance of phenoxyalkanoic acid herbicides in soils. Although fungi can also degrade phenoxyalkanoic acid herbicides, this degradation mechanism is much less important in typical arable soils. Also, the contribution of the photodegradation mechanism is much lower than that of microbial degradation. However, photodegradation may be the predominant process in dry soils. Additionally, the extent of volatilisation of phenoxyalkanoic acid herbicides from soils is limited. The microbial degradation of phenoxyalkanoic acid herbicides is usually a very fast process, and the degradation rates are dependent on the history of the use of herbicides in the soil. Frequent use of herbicides from this group increases the concentration of bacterial strains capable of degrading them and enables the adaptation of bacteria to utilize the compounds as a carbon and energy source.
A review of the studies of degradation by bacteria indicated that degradation mechanisms of the test material are relatively well recognised. From 45 to 70 % of phenoxyalkanoic acid herbicide added to soil is mineralised to CO2, and even up to 40 % of C from its phenolic rings may become a constituent of the biogenic residues of soil at the end of the degradation process. Therefore, the non-extractable residue for phenoxyalkanoic acid herbicides cannot be a threat to the environment.
For the test material the following values were reported, Kd = 0.48 mL/g, Koc = 35 mL/g and DT50 = 8.3 days.
In conclusion, the examined phenoxyalkanoic acids, similar to the majority of acidic herbicides, are poorly adsorbed in soils and rapidly degraded during the microbial degradation process.
Referenceopen allclose all
Physicochemical parameters and mean log Kd values
Compound | pKa | Log Kow | Mean Log Kd (L/kg) | SD Log Kd (L/kg) | Number of Samples |
2,4-D | 2.8 | 2.81 | 0.16 | 0.55 | 271 |
2,4-DB | 4.1 | 3.53 | 0.52 | 0.51 | 9 |
2,4-DP | 3 | 3.43 | 0.02 | 0.87 | 18 |
MCPA | 3.7 | 2.56 | -0.1 | 0.56 | 109 |
MCPB | 4.5 | 3.28 | 0.07 | 0.6 | 5 |
Test material | 3.1 | 3.13 | -0.38 | 0.56 | 57 |
pKa values from the IUPAC Footprint database (IUPAC 2011), log Kow are
from (Ruelle 2000), not corrected for ionization of the compounds, and
compatible with recommended values of the CODATA LOGKOW database
(Sangster 2012)
Sorbent–water distribution coefficients Kd for 2,4-D and Test material in 0.01 M CaCl2 solutions
Sorbent | Kd (L/kg) | Kd (L/m^2) | pH (-) | PZNC (-) | Temp (°C) | ||
2,4-D | Test Material | 2,4-D | Test Material | ||||
Quartz (Clausen et al. 2001) | approx. 0 | 0.025 | approx. 0 | 0.00003 | 6.7 | 2–3 | 10 |
Calcite (Clausen et al. 2001) | 0.046 | 0.116 | 0.000008 | 0.00002 | 8.8 | 8.6 | 10 |
α-Alumina (Clausen et al. 2001) | 0.338 | 0.54 | 0.000025 | 0.00004 | 7 | 8.7 | 10 |
α-Alumina (Goyne et al. 2004) | 02-Dec | na | 0.00005 | na | 5.6 - 6.4 | 6.5–6.9 | 20 |
Ferrihydrite (Celis et al. 1999) | 409 | na | 0.0013 | na | 5.4 | na | 20 |
Ferrihydrite (Clausen and Fabricius 2001) |
460 | 690 | 0.002 | 0.003 | 4.6 | 7.8–7.9 | 10 |
Goethite (Clausen and Fabricius 2001) |
15 | 30 | 0.00025 | 0.0005 | 4.6 | 7.5–9.2 | 10 |
Lepidocrocite (Clausen and Fabricius 2001) |
12 | 47 | 0.0001 | 0.0004 | 4.6 | 6.7–7.5 | 10 |
Kaolinite (Clausen et al. 2001) | 0.0425 | 0.238 | 0.000005 | 0.000028 | 5.9 | 4.5 | 10 |
Montmorillonite (Celis et al. 1999) | approx. 0 | na | na | na | 7.9 | na | 20 |
Soil humic acid (Celis et al. 1999) | 60 | na | na | na | 2.9 | na | 20 |
Fluka humic acid (Celis et al. 1999) | 14 (Pesticide anion) | na | na | na | 4.6 | na | 20 |
Peat (Tulp et al. 2009) | 10 (Pesticide anion) | 5 (Pesticide anion) | na | na | < 8 | na | 25 |
* With different mesoporosities: BET surface areas 37–242 m2 /g, aqueous
concentration 1 mg/L
na: not available
Table 1: Texture, pH, content of humus and WHC of the soils and adsorption of the test material described by Kd and Koc.
Depth (cm) |
Clay |
Silt |
Coarse Silt |
Fine Sand |
Coarse Sand |
Humus |
pH (H2O) |
WHC |
Adsorption |
|
Kd |
Koc |
|||||||||
Flakkebjerg soil |
||||||||||
0 - 30 |
15.8 |
18.4 |
|
44.2 |
19.2 |
2.4 |
6.9 |
31 |
0.12 |
8.4 |
Roskilde soil |
||||||||||
0 - 30 |
13.2 |
14.1 |
14.5 |
31.5 |
24.0 |
2.6 |
6.7 |
31 |
0.20 |
13.3 |
Jyndevad soil |
||||||||||
0 – 25 |
4.0 |
3.8 |
2.8 |
20.4 |
66.5 |
2.4 |
6.6 |
29 |
0.07 |
5.3 |
Clay: < 0.002 mm; silt: 0.002 - 0.02 mm; coarse silt: 0.02 - 0.063 mm; fine sand: 0.063 - 0.2 mm; coarse sand: 0.2 - 2 mm; humus: %C x 1.72.
WHC: Water holding capacity, % of wet soil.
Concentration of Test Material in a 0.582 ppm Solution After 24 Hours of Contact with a Culture Tube
Replicate |
Concentration (ppm) |
Recovery (%) |
A |
0.572 |
98.3 |
B |
0.571 |
98.1 |
The concentration was calculated from the radioactivity of the solution in dpm/mL divided by the specific activity of the test material, 3 641 dpm/μg.
Adsorption and Desorption Results for Aqueous Test Material and Fox Sandy Loam: Mean of Duplicate LSC Analyses of Each Replicate
Initial Solution Concentration (ppm) |
Replicate Set |
Adsorption |
Percent Desorbed (%) |
|
Solution Concentration (ppm) |
Soil Concentration (ppm) |
|||
11.965 |
A |
11.112 |
2.844 |
26.79 |
B |
11.050 |
3.050 |
26.59 |
|
5.923 |
A |
5.455 |
1.558 |
30.74 |
B |
5.503 |
1.400 |
29.14 |
|
1.239 |
A |
1.158 |
0.270 |
28.15 |
B |
1.135 |
0.347 |
38.04 |
|
0.606 |
A |
0.554 |
0.173 |
25.43 |
B |
0.547 |
0.194 |
25.26 |
Adsorption and Desorption Results for Aqueous Test Material and Plano Silt Loam: Mean of Duplicate LSC Analyses of Each Replicate
Initial Solution Concentration (ppm) |
Replicate Set |
Adsorption |
Percent Desorbed (%) |
|
Solution Concentration (ppm) |
Soil Concentration (ppm) |
|||
11.965 |
A |
10.092 |
6.243 |
27.47 |
B |
10.133 |
6.107 |
28.74 |
|
5.923 |
A |
4.927 |
3.318 |
28.93 |
B |
4.925 |
3.327 |
27.95 |
|
1.239 |
A |
1.014 |
0.749 |
29.37 |
B |
1.046 |
0.641 |
29.80 |
|
0.606 |
A |
0.495 |
0.367 |
28.07 |
B |
0.504 |
0.340 |
35.88 |
Adsorption and Desorption Results for Aqueous Test Material and Hagerstown Silty Clay Loam: Mean of Duplicate LSC Analyses of Each Replicate
Initial Solution Concentration (ppm) |
Replicate Set |
Adsorption |
Percent Desorbed (%) |
|
Solution Concentration (ppm) |
Soil Concentration (ppm) |
|||
11.965 |
A |
10.561 |
4.681 |
31.00 |
B |
10.621 |
4.481 |
30.62 |
|
5.923 |
A |
5.252 |
2.236 |
33.63 |
B |
5.208 |
2.383 |
183.80* |
|
1.239 |
A |
1.096 |
0.474 |
33.33 |
B |
1.086 |
0.509 |
33.99 |
|
0.606 |
A |
0.530 |
0.251 |
28.29 |
B |
0.548 |
0.192 |
44.27 |
* This result is recognised as being unrealistic, and is an example of the suspect values previously discussed
Adsorption and Desorption Results for Aqueous Test Material and Plainfield Sand: Mean of Duplicate LSC Analyses of Each Replicate
Initial Solution Concentration (ppm) |
Replicate Set |
Adsorption |
Percent Desorbed (%) |
|
Solution Concentration (ppm) |
Soil Concentration (ppm) |
|||
11.965 |
A |
11.207 |
2.527 |
36.72 |
B |
11.119 |
2.822 |
36.25 |
|
5.923 |
A |
5.571 |
1.174 |
35.86 |
B |
5.513 |
1.365 |
-3.59* |
|
1.239 |
A |
1.149 |
0.298 |
45.97 |
B |
1.147 |
0.306 |
37.58 |
|
0.606 |
A |
0.593 |
0.042 |
176.19* |
B |
0.551 |
0.182 |
51.65 |
* This result is recognised as being unrealistic, and is an example of the suspect values previously discussed
Linear Regression Analysis for Each Soil
Soil |
Freundlich Constant |
Organic Content (%) |
Correlation Coefficient |
|
Kd |
l/n |
|||
Fox sandy loam |
0.298 |
0.942 |
2.3 |
0.996 |
Plano silt loam |
0.686 |
0.962 |
5.9 |
0.999 |
Hagerstown silty clay loam |
0.428 |
1.012 |
2.5 |
0.997 |
Plainfield sand |
0.199 |
1.093 |
0.8 |
0.950 |
Cumulative Percent of Applied Radioactivity Trapped in NaOH Solution
Day |
Applied Radioactivity (%) |
Cumulative Applied Radioactivity (%) |
6 |
5.9 |
5.9 |
20 |
23.4 |
29.3 |
30 |
10.0 |
39.3 |
Relative Mobility (Frontal Rf Value) of Soil-Aged Test Material, Nonsoil-Aged Test Material, and Reference Standards in Fox Sandy Loam Soil
Compound |
Frontal Rf Value |
Mobility Class* |
K Value ** |
Nonsoil-Aged Test Material |
0.86+ |
(4) |
0.576 |
Soil-Aged Test Material |
0.08++ |
(1) |
12.8 |
2,4-D |
0.56 |
(3) |
1.25 |
Paraquat |
0.04 |
(1) |
26.3 |
*As defined by EPA Pesticide Assessment Guidelines, Subdivision N, Page 67 (1982):
(1) Immobile (Rf = 0.0 to 0.09)
(2) Low mobility (Rf = 0.1 to 0.34)
(3) = Intermediate mobility (Rf = 0.35 to 0.64)
(4) = Mobile (Rf = 0.65 to 0.89)
(5) = Very mobile (Rf = 0.90 to 1.0)
**K is the sorption coefficient calculated from relative mobility.
+ This value represents an average of two determinations.
++ This value represents an average of three determinations.
ADSORPTION AND DESORPTION
Factors affecting the extent of adsorption and desorption
- Adsorption and desorption of an acidic pesticide is a time-dependent process, dependent on both physical and chemical properties of the soil and adsorbed compound. Studies on the kinetics of adsorption and desorption of phenoxyalkanoic acid herbicides using the batch method demonstrated that these processes proceed rapidly, and equilibrium was most frequently reached within 4 h to 12 hours. It should be noted that, in contrast to laboratory batch sorption studies, the adsorption and degradation processes occur simultaneously under field conditions.
- Like other weak carboxylic acids and sulfonylureas, phenoxyalkanoic acid herbicides are poorly adsorbed in soils, and the extent of sorption is negatively correlated with soil pH and positively correlated with organic matter content. The organic carbon–normalised adsorption coefficient (KOC) most frequently has values within the range of 20 to 167 mL/g for the test material and its isomer The values of KOC increase with depth in the soil profiles, which indicates an increasing contribution of soil inorganic components to the overall sorption. Studies indicated that no significant differences were observed between the adsorption of the test susbtance and and its isomer in soils.
Mechanisms of Adsorption
- Studies on the adsorption of the test material on quartz demonstrated that quartz primarily adsorbs the neutral forms of these compounds. Because quartz and amorphous silica are the main components of the sand and silt fractions of soil, these fractions can also take part in the adsorption of phenoxyalkanoic acid herbicides. However, the conditions for adsorption include an acidic reaction of the soil and the coating of particles of sand and silt with organic matter in concentrations lower than 0.2 % to 0.4 %. In contrast, adsorption by the clay fraction appears to be negligible in most soils, as clay minerals are coated with a relatively thick layer of organic matter (the organic matter content in the clay fraction usually exceeds 4 %), which blocks the sorption sites capable of adsorbing phenoxyalkanoic acid herbicide. However, statistical analysis of results clearly indicates a certain contribution of clay minerals to the adsorption of 2,4-D. Thus, it seems that the adsorption of phenoxyalkanoic acid herbicides by minerals from the clay fraction is possible in certain types of soils.
-The finding that adsorption of the test material in soils is significantly inversely correlated with their log D values may have significant effects on advances in the prediction of adsorption of these compounds in soils.
Degradation
- Degradation of the test material by indigenous microbial communities in 15 soils from distinct locations on five continents indicated that the degradation was very well described by either the three-half-order kinetic model or the zero-order model.
- By-products of the first degradation step are pyruvate for the test material.
- The first step in the degradation pathway of the test material is very often initiated by the α-ketoglutarate–dependent dioxygenase enzyme encoded by the class III of tfdA genes. For this reason, an increase of bacteria harboring this class of tfdA genes was observed during the lag and steep phases of degradation, followed by a decrease at the end of the steep phase. However, the concentration of bacteria containing class III tfdA genes was much higher at the end of degradation than at the beginning. Therefore, during repeated application of phenoxyalkanoic acid herbicides, the degradation rate was higher and the lag phase was not observed or was very short.
- Additionally, specific enzymes, which most likely are subunits of Rieske nonheme iron oxygenase encoded by cadA genes harbored by α-proteobacteria, are able to initiate the first step of both the (R)-isomer and (S)-isomer degradation. According to Nielsen et al., products of cadB genes are also involved in the degradation of phenoxyalkanoic acids. It should be noted that cadA genes were also found in β-proteobacteria and γ-proteobacteria. According to Zakaria et al., this is evidence of lateral gene transfer during the adaptation of microorganisms by developing new catabolic degradation pathways.
- It was found that the first step in the degradation of the (R)-isomer was dependent on the enantiospecific Fe(II)-dependent and α-ketoglutarate-dependent dioxygenase enzyme encoded by the rdpA gene, whereas degradation of the (S)-enantiomer was dependent on the enzyme encoded by the sdpA gene. Moreover, Zakaria et al. suggested that the sdpA gene may be involved in both the (R)-enantiomer and (S)-enantiomer of test material degradation. Both the rdpA and sdpA genes were initially found in α-proteobacteria, but Zakaria et al. found that they are also involved in degradation by β-proteobacteria and γ-proteobacteria.
- Dioxygenases were also most likely capable of cleaving the ether linkage in the test material.
Photodegradation
- Romero et al. postulated that for the test material in moist soil, photodegradation may be an important process in the first two days of exposure to sunlight and may be the predominant process in dry soils.
INFLUENCE OF LIMING AND FERTILISERS ON LEACHING
- Liming is widely used in arable acidic soils to improve their properties, resulting in an increase in crop yield. The increase in the Ca2+ concentration causes a slight increase in the adsorption of anions of phenoxyalkanoic acid herbicides on organic matter, kaolinite, and quartz through a Ca-bridging mechanism. Despite this, an increase of pH in the plough layer of arable soil, which is the result of liming, decreases the adsorption of phenoxyalkanoic acid herbicides because the affinity of organic matter for the anionic form is at least 10 times less than that for the neutral forms of these compounds.
- The soil thin-layer chromatography studies of Horvat et al. indicated that the use of NPKs (multinutrient complex fertilisers containing nitrogen, phosphorus, and potassium) and CAN (a mixture of ammonium nitrate and calcium/ magnesium carbonate) increased the mobility of the test material. Application of fertilisers such as ammonium phosphate or NPK increases the concentration of phosphate anions in soils.
ESTIMATION OF THE LEACHING POTENTIAL
- Phenoxyalkanoic acid herbicides are able to penetrate through the soil profile, and a majority of pesticide databases provide indexes determining their potential for water contamination. The ability to predict water pollution based on physical and chemical properties of a pesticide is one of the principal goals of pesticide science. The data set collected in the present study facilitates determination of one of the most frequently used indexes to rank the leaching potential of pesticides, the Groundwater Ubiquity Score (GUS) index. According to Gustafson, the potential of a pesticide to translocate through the soil profile to groundwater can be estimated using the following equation:
GUS = log(DT50) x [4 – log (KOC)]
- This simple equation estimates the GUS index based on degradation and adsorption, 2 crucial processes affecting the fate of pesticides in soils. Therefore, predictions based on this index are often correlated with the results of groundwater monitoring studies. Pesticides with GUS < 1.8 are considered nonleachers, those with 1.8 < GUS < 2.8 are transient, and those with GUS > 2.8 are leachers.
- The obtained data indicate that, for typical soil properties, mecoprop is a transient leacher. Note that mecoprop-P, which is currently mostly used in commercial preparations, has lower degradation rates than the racemic mixtures, and the GUS indexes should be slightly higher than that for the test material.
Description of key information
Key Study: Helweg (1993)
Under the conditions of the study the adsorption of the test material was low with Kd values of 0.07 - 0.2 and Koc values of 8.4, 13.3 and 5.3 in the three soil types.
Supporting Study: de Lipthay et al. (2007)
The impact of the herbicide concentration (0.10 - 10 000 mg kg^-1) and addition of organic and inorganic nutrients on the test material mineralisation in aquifer and soil samples was studied in laboratory experiments. A shift from non-growth to growth-linked mineralisation kinetics was observed in aquifer sediment with test material concentrations >10.0 mg kg^-1. The shift was apparent at higher herbicide concentrations in soil coinciding with a lower bioavailable fraction and a higher herbicide sorption to soil. Herbicide addition did not affect the bacterial density, although test material applied at 10 000 mg kg^-1 stimulated growth of specific degraders. Generally, nutrient amendments did not stimulate mineralisation at the lowest herbicide concentrations. In contrast, the mineralisation rate of higher herbicide concentrations was significantly stimulated by the amendment of inorganic nutrients.
Supporting Study: Obrist (1986a)
Under the conditions of this study, the test material was classified as very mobile in Plainfield sand, Mississippi silt loam, and Fox sandy loam, and as mobile in Hagerstown silty clay loam. The sorption coefficient calculated from relative mobility was 0.4 for Plainfield sand, 0.481 for Fox sandy loam, 0.47 for Mississippi silt loam and 0.780 for Hagerstown silty clay loam.
Supporting Study: Werner et al. (2013)
The data review suggests that sorption of 2,4-D can be rationalised based on the soil parameters pH, foc, Oxalate Fe, and DCB Fe in combination with sorption coefficients measured independently for humic acids and ferrihydrite, and goethite. Soil organic matter and iron oxides appear to be the most relevant sorbents for phenoxy herbicides. The mean log Kd (L/kg) was found to be – 0.38 for the test material, the pKa and log Kow were also reported as 3.1 and 3.13.
Supporting Study: Paszko et al. (2015)
For the test material the following values were reported, Kd = 0.48 mL/g, Koc = 35 mL/g and DT50 = 8.3 days.
Read Across Supporting Study: Fava et al. (2005)
Under the conditions of this study the Koc was 93 ± 1 and the DT50 was 0.4 days. The test material was considered a non-leacher.
Supporting Study: Obrist (1986b)
During aerobic incubation of 14C-test material in Fox sandy loam soil, approximately 39 % of the applied radioactivity was trapped in NaOH by Day 30. The soil-aged residues of the test material were much less mobile (lower Rf value) on soil TLC than nonsoil-aged test material. Soil-aged test material and 14C-Paraquat were classified as immobile, while 14C-2,4-D was classified as having an intermediate mobility and nonsoil-aged test material was classified as mobile. Since little mobility of soil-aged test material was observed on a sandy loam soil, no other soil types were tested.
Supporting Study: Obrist (1986/1987)
Under the conditions of this study: Kd (Fox - sandy loam) = 0.298, Kd (Plano - silt loam) = 0.686, Kd (Hagerstown silty - clay loam) = 0.428 and Kd (Plainfiled sand) = 0.199.
Key value for chemical safety assessment
Additional information
de Lipthay et al. (2007)
Under the conditions of the study, the test material was mineralised in the tested samples of aquifer sediment and soil, but the rate and extent were highly influenced by herbicide structure, incubation temperature, herbicide concentration and availability of additional nutrients. Shifts from non-growth-linked to growth-linked mineralisation kinetics were detected at different herbicide concentrations depending on the type of herbicide and the origin of the sample. The higher concentration thresholds in the soil samples compared to aquifer samples were probably due to higher sorption. Furthermore, the higher density of indigenous herbicide degraders in soil compared to the aquifer sediment is very likely a key factor. Attempts to stimulate indigenous degraders by addition of organic and inorganic nutrients suggested that the mineralisation of phenoxyalkanoic acid herbicides in soil and aquifer sediment contaminated with high concentrations may benefit from the amendment of inorganic nutrients. In contrast minor mineralisation was apparent with low herbicide concentrations and no stimulation was possible by supplying either organic or inorganic nutrients.
Obrist (1986a)
The mobility of the test material in four soils using soil thin layer chromatography was investigated in compliance with GLP. The study was awarded a reliability score of 2 in accordance with the criteria set forth by Klimisch et al. (1997).
Glass plates were coated with a thin layer of one of four different soils. Radiolabelled test material was applied, and water was allowed to migrate up the plates. The distribution of radioactivity on each soil was then determined using a linear analyser. The frontal relative migration (Rf) value of each was measured and the mobility determined.
Under the conditions of this study, the test material was classified as very mobile in Plainfield sand, Mississippi silt loam, and Fox sandy loam, and as mobile in Hagerstown silty clay loam. The sorption coefficient calculated from relative mobility was 0.4 for Plainfield sand, 0.481 for Fox sandy loam, 0.47 for Mississippi silt loam and 0.780 for Hagerstown silty clay loam.
Fava et al. (2005) Read Across to CMP (4-chloro-2-methylphenol)
The study investigated the leaching potential of the test material using the results of Koc and soil DT50 experiments through the GUS Index. The study was awarded a reliability score of 2 in accordance with the criteria set forth by Klimisch et al. (1997).
From the degradation curve versus time of the examined metabolite fitted with first order kinetics the test material degraded very quickly (0.4 days). The Koc of the test material was 93 and the GUS value calculated from the equation was -0.88. The test material had a Koc value which was typical of a mobile compound.
Under the conditions of this study the Koc was 93 ± 1 and the DT50 was 0.4 days. The test material was considered a non-leacher.
Werner et al. 2013
2,4-dichlorophenoxyacetic acid (2,4-D) and other phenoxy herbicide sorption experiments were reviewed. The study was awarded a reliability score of 2 in accordance with the criteria set forth by Klimisch et al. (1997).
A database with 469 soil–water distribution coefficients Kd (in litres per kilogram) was compiled: 271 coefficients are for the phenoxy herbicide 2,4-D, 9 for 4-(2,4- dichlorophenoxy)butyric acid, 18 for 2-(2,4-dichlorophenoxy)propanoic acid, 109 for 2-methyl-4-chlorophenoxyacetic acid, 5 for 4-(4-chloro-2-methylphenoxy)butanoic acid, and 57 for the test material. The following parameters characterising the soils, solutions, or experimental procedures used in the studies were also compiled if available: Solution CaCl2 concentration, pH, pre-equilibration time, temperature, soil organic carbon content (foc), percent sand, silt and clay, oxalate extractable aluminium, oxalate extractable iron (Oxalate Fe), dithionite–citrate–bicarbonate extractable aluminium, dithionite–citrate–bicarbonate extractable iron (DCB Fe), point of zero negative charge, anion exchange capacity, cation exchange capacity, soil type, soil horizon or depth of sampling, and geographic location. Kd data were also compiled characterising phenoxy herbicide sorption to the following well-defined sorbent materials: Quartz, calcite, α-alumina, kaolinite, ferrihydrite, goethite, lepidocrocite, soil humic acid, Fluka humic acid, and Pahokee peat.
The data review suggests that sorption of 2,4-D can be rationalised based on the soil parameters pH, foc, Oxalate Fe, and DCB Fe in combination with sorption coefficients measured independently for humic acids and ferrihydrite, and goethite.
Soil organic matter and iron oxides appear to be the most relevant sorbents for phenoxy herbicides.The mean log Kd (L/kg) was found to be – 0.38 for the test material, the pKa and log Kow were also reported as 3.1 and 3.13.
Helweg (1993)
Adsorption of 14C-ring-labelled test material was determined according to the OECD Test Guideline Adsorption - Desorption in three different soil types. The study was awarded a reliability score of 2 in accordance with the criteria set forth by Klimisch et al. (1997).
Soils were from three Danish Experimental Farms (#1 Flakkebjerg, #2 Roskilde and #3 Jyndevad, providing sandy loam, sandy loam and coarse sandy soil, respectively).
Adsorption (Kd) of the test material was determined at 20 °C by shaking 5 g of soil with 25 mL 0.01 M CaCl2 added to 14C-test material (5 mg L^-1) for 16 h. To avoid degradation the soils were sterilised by irradiation. The soil was precipitated by centrifugation and 14C was counted in aliquots of the supernatant to calculate concentrations of the test material in soil and water. Koc was calculated from Kd and the organic C contents of the soils.
The adsorption of the test material was low with Kd values of 0.07 - 0.2 and Koc values of 8.4, 13.3 and 5.3 in the three soil types (Flakkebjerg, Roskilde and Jyndevad, respectively). With such low adsorption values the test material is likely to leach if not decomposed in the surface soil.
Adsorption / Desorption. Obrist (1986/1987)
The adsorption/desorption of the test material on representative agricultural soils was investigated in a study according to Pesticide Assessment Guidelines of the Environmental Protection Agency (EPA), Subdivision N, Section 163-1. The study was awarded a reliability score of 2 in accordance with the criteria set forth by Klimisch et al. (1997).
Four diverse soils were each equilibrated with aqueous solutions of the test material. The resulting equilibrium concentration of the test material in water was measured, and the soil equilibrium concentration was calculated. Four aqueous concentrations were used to construct a Freundlich isotherm of adsorption at 25 °C for each soil.
Aqueous test material adsorbed to some degree in all soils studied. At 25 °C, this adsorption followed a Freundlich isotherm. Freundlich constant (Kd) values ranged from 0.20 to 0.69. Desorption of adsorbed test material was usually in the range of 25 to 50 % of the amount adsorbed, with some scattered values due to the small amount of test material adsorbed by the soil. Under the conditions of this study: Kd (Fox - sandy loam) = 0.298, Kd (Plano - silt loam) = 0.686, Kd (Hagerstown silty - clay loam) = 0.428 and Kd (Plainfiled sand) = 0.199.
Adsorption / desorption. Obrist (1986b)
During aerobic incubation of 14C-test material in Fox sandy loam soil, approximately 39 % of the applied radioactivity was trapped in NaOH by Day 30. A subsample of the soil that contained the aged test material residues was then used in a soil mobility study. The study was awarded a reliability score of 2 in accordance with the criteria set forth by Klimisch et al. (1997).
A 20 x 20 cm glass plate was coated with a thin layer of Fox sandy loam soil. Radiolabelled, soil-aged test material; nonsoil-aged test material; 2,4-D; and Paraquat were each applied to the soil, and water was allowed to migrate up the plate. The distribution of radioactivity for each sample was then determined with a linear analyser. The frontal relative migration (Rf) value of each was measured, the mobility class was determined, and the sorption coefficient was calculated.
Soil-aged residues of the test material were much less mobile (lower Rf value) on soft thin layer chromatography than non-soil ages test material.
Paszko et al. (2015)
A literature review of phenoxyalkanoic acid herbicides was performed to compare the extent of their adsorption in soils and degradation rates to assess their potential for groundwater contamination. The study was awarded a reliability score of 2 in accordance with the criteria set forth by Klimisch et al. (1997).
Comparison of published data concerning the adsorption of phenoxyalkanoic acid herbicides in soils found that, the adsorption of the test matreial is significantly inversely correlated with the logD values. Modeling of their adsorption, based on the negative relationship between adsorption of anions on organic matter and log D values, is possible.
Microbial degradation is the major process that controls the rate of the disappearance of phenoxyalkanoic acid herbicides in soils. Although fungi can also degrade phenoxyalkanoic acid herbicides, this degradation mechanism is much less important in typical arable soils. Also, the contribution of the photodegradation mechanism is much lower than that of microbial degradation. However, photodegradation may be the predominant process in dry soils. Additionally, the extent of volatilisation of phenoxyalkanoic acid herbicides from soils is limited. The microbial degradation of phenoxyalkanoic acid herbicides is usually a very fast process, and the degradation rates are dependent on the history of the use of herbicides in the soil. Frequent use of herbicides from this group increases the concentration of bacterial strains capable of degrading them and enables the adaptation of bacteria to utilize the compounds as a carbon and energy source.
A review of the studies of degradation by bacteria indicated that degradation mechanisms of the test material are relatively well recognised. From 45 to 70 % of phenoxyalkanoic acid herbicide added to soil is mineralised to CO2, and even up to 40 % of C from its phenolic rings may become a constituent of the biogenic residues of soil at the end of the degradation process. Therefore, the non-extractable residue for phenoxyalkanoic acid herbicides cannot be a threat to the environment.
For the test material the following values were reported, Kd = 0.48 mL/g, Koc = 35 mL/g and DT50 = 8.3 days.
In conclusion, the examined phenoxyalkanoic acids, similar to the majority of acidic herbicides, are poorly adsorbed in soils and rapidly degraded during the microbial degradation process.
Information on Registered Substances comes from registration dossiers which have been assigned a registration number. The assignment of a registration number does however not guarantee that the information in the dossier is correct or that the dossier is compliant with Regulation (EC) No 1907/2006 (the REACH Regulation). This information has not been reviewed or verified by the Agency or any other authority. The content is subject to change without prior notice.
Reproduction or further distribution of this information may be subject to copyright protection. Use of the information without obtaining the permission from the owner(s) of the respective information might violate the rights of the owner.
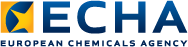