Registration Dossier
Registration Dossier
Data platform availability banner - registered substances factsheets
Please be aware that this old REACH registration data factsheet is no longer maintained; it remains frozen as of 19th May 2023.
The new ECHA CHEM database has been released by ECHA, and it now contains all REACH registration data. There are more details on the transition of ECHA's published data to ECHA CHEM here.
Diss Factsheets
Use of this information is subject to copyright laws and may require the permission of the owner of the information, as described in the ECHA Legal Notice.
EC number: 289-339-5 | CAS number: 87741-01-3
- Life Cycle description
- Uses advised against
- Endpoint summary
- Appearance / physical state / colour
- Melting point / freezing point
- Boiling point
- Density
- Particle size distribution (Granulometry)
- Vapour pressure
- Partition coefficient
- Water solubility
- Solubility in organic solvents / fat solubility
- Surface tension
- Flash point
- Auto flammability
- Flammability
- Explosiveness
- Oxidising properties
- Oxidation reduction potential
- Stability in organic solvents and identity of relevant degradation products
- Storage stability and reactivity towards container material
- Stability: thermal, sunlight, metals
- pH
- Dissociation constant
- Viscosity
- Additional physico-chemical information
- Additional physico-chemical properties of nanomaterials
- Nanomaterial agglomeration / aggregation
- Nanomaterial crystalline phase
- Nanomaterial crystallite and grain size
- Nanomaterial aspect ratio / shape
- Nanomaterial specific surface area
- Nanomaterial Zeta potential
- Nanomaterial surface chemistry
- Nanomaterial dustiness
- Nanomaterial porosity
- Nanomaterial pour density
- Nanomaterial photocatalytic activity
- Nanomaterial radical formation potential
- Nanomaterial catalytic activity
- Endpoint summary
- Stability
- Biodegradation
- Bioaccumulation
- Transport and distribution
- Environmental data
- Additional information on environmental fate and behaviour
- Ecotoxicological Summary
- Aquatic toxicity
- Endpoint summary
- Short-term toxicity to fish
- Long-term toxicity to fish
- Short-term toxicity to aquatic invertebrates
- Long-term toxicity to aquatic invertebrates
- Toxicity to aquatic algae and cyanobacteria
- Toxicity to aquatic plants other than algae
- Toxicity to microorganisms
- Endocrine disrupter testing in aquatic vertebrates – in vivo
- Toxicity to other aquatic organisms
- Sediment toxicity
- Terrestrial toxicity
- Biological effects monitoring
- Biotransformation and kinetics
- Additional ecotoxological information
- Toxicological Summary
- Toxicokinetics, metabolism and distribution
- Acute Toxicity
- Irritation / corrosion
- Sensitisation
- Repeated dose toxicity
- Genetic toxicity
- Carcinogenicity
- Toxicity to reproduction
- Specific investigations
- Exposure related observations in humans
- Toxic effects on livestock and pets
- Additional toxicological data

Basic toxicokinetics
Administrative data
- Endpoint:
- basic toxicokinetics in vivo
- Type of information:
- other: EU Risk Assessment
- Adequacy of study:
- other information
- Reliability:
- other:
Data source
Reference
- Reference Type:
- other: EU Risk Assessment
- Title:
- European Union Risk Assessment Report 1,3-BUTADIENE, CAS No: 106-99-0, EINECS No:203-450-8
- Author:
- [ECB] European Chemicals Bureau
- Year:
- 2 002
Materials and methods
- Objective of study:
- toxicokinetics
- Principles of method if other than guideline:
- EU Risk Assessment on the major component of C4 Hydrocarbons: 1,3-Butadiene
(extracted data from endpoint summary) - GLP compliance:
- not specified
Test material
- Reference substance name:
- Buta-1,3-diene
- EC Number:
- 203-450-8
- EC Name:
- Buta-1,3-diene
- Cas Number:
- 106-99-0
- Molecular formula:
- C4H6
- IUPAC Name:
- buta-1,3-diene
- Details on test material:
- - Name of test material (as cited in study report): 1,3-butadiene
- CAS number: 106-99-0
- EINECS number: 203-450-8
Constituent 1
Results and discussion
Toxicokinetic / pharmacokinetic studies
- Details on absorption:
- Studies in rodents and non-human primates have shown that butadiene is absorbed via the lungs. In rodents, uptake and metabolism of butadiene obeys simple first order kinetics at concentrations up to about 1,500 ppm, above which saturation of the process appears to occur.
There are no data on the toxicokinetics of butadiene following oral or dermal exposure, and although the possibility of uptake via these routes cannot be entirely discounted, their contribution to uptake and metabolism of butadiene is anticipated to be negligible. In addition, there is no evidence of any significant potential for dermal uptake from a comparison of the results of whole-body inhalation exposure studies compared with those in which exposure was nose-only. - Details on distribution in tissues:
- Butadiene is widely distributed throughout the body.
There is no evidence for bioaccumulation of butadiene.
- Details on excretion:
- The main route of elimination of butadiene and its metabolites in rodents and primates is urinary excretion or exhalation in the breath.
Minor faecal excretion also occurs.
In rodents, urinary excretion takes place in two phases with 77-99% of the inhaled dose excreted with a half-life of a few hours in rodents, while
the remainder is excreted with a half-life of several days. There is no evidence for bioaccumulation of butadiene.
Metabolite characterisation studies
- Metabolites identified:
- yes
- Details on metabolites:
- The first step in the metabolic pathway is the formation of epoxybutene, catalysed by mixed function oxygenases. The further metabolism of epoxybutene can proceed by a number of different pathways. There is some conjugation with glutathione. A second possible pathway is hydrolysis to butenediol, catalysed by epoxide hydrolase. Another possibility is further epoxidation to diepoxybutane. Further epoxidation and/or hydrolysis reactions can then occur, which ultimately lead to erythritol formation. It is not clear at which stage or stages in the pathway CO2 is formed.
Any other information on results incl. tables
Toxicokinetics:
There are quantitative species differences in the toxicokinetics of butadiene. In comparison with the rat, the mouse absorbs and retains approximately 4-7 fold higher concentrations of butadiene per kg bodyweight. The mouse also produces approximately 2-20 fold higher concentrations of the metabolite, epoxybutene, than does the rat, for equivalent exposures. Very low concentrations of the diepoxide metabolite have been detected in the blood and various tissues of rats and mice; this metabolite has been tentatively identified in the blood of monkeys, in vivo. Again, where measurements are available, tissue levels of diepoxybutane are generally higher in mice compared with rats, by up to 163-fold. In vitro studies indicate that in the mouse, lung and liver tissue have similar capacity for butadiene metabolism while in rats and humans, liver tissue has a greater capacity for metabolism than does lung tissue, although some metabolism does take place in lung tissue. Detoxification pathways are kinetically favoured over activation pathways in rodent and human tissue, although the ratio of activation:detoxification is highest in mouse tissue compared with rat or human tissue. In mouse liver and lung tissue, detoxification of epoxybutene appears to be mainly by conjugation with glutathione, with hydrolysis to butenediol a relatively minor pathway. In comparison, in human liver and lung, detoxification of epoxybutene is primarily by hydrolysis, with only some glutathione conjugation; this finding from in vitro studies supports the in vivo human metabolism data. Formation of the diepoxide has been demonstrated in mouse liver tissue exposed to butadiene in vitro, but not in rat or human tissue, although formation of diepoxybutane has been demonstrated cDNA-expressed human liver microsomes exposed to epoxybutene. From the limited comparative information available from in vitro and in vivo studies, it appears that in relation to the formation of epoxide metabolites, the metabolism of butadiene in humans is quantitatively more similar to that in the rat, rather than the mouse. However, in vitro studies have demonstrated considerable inter-individual variability in the oxidative metabolism of butadiene. A number of PBPK models have been developed to try to characterise the internal tissue levels of butadiene and its epoxide metabolites. In general, whilst these models are useful and aid understanding of the kinetics of butadiene and its epoxide metabolites between species, they do not yet provide any clearer understanding of the basis for the marked species differences in susceptibility. Although the known quantitative species differences in the metabolism of butadiene to its epoxide metabolites, demonstrated in vitro, in vivo and in PBPK modelling, may explain in part the very marked difference in toxicity of butadiene between rats and mice, it is not possible, on the basis of the currently available evidence, to attribute the differences in toxicity between the rat and mouse solely to these quantitative differences in metabolism. Nor is it possible, on the basis of the available in vitro data in rodents and humans which indicate considerable inter-individual variability in human tissue metabolic capacity, to exclude the possibility that some humans could be similar to mice in their capacity for metabolism of butadiene to more active intermediates.
Genotoxicity of metabolites:
Epoxybutene and diepoxybutane have been studied in a number of in vitro and in vivo studies. Positive results have been obtained
for epoxybutene and diepoxybutane in the Ames test, in the absence of metabolic activation and for diepoxybutane which has also been tested in the presence of metabolic activation.
Epoxybutene, epoxybutanediol and diepoxybutane induced an increase in mutation frequency at the tk and hprt locus in TK6 human lymphoblastoid cells, with diepoxybutane producing a response at concentrations ~40 to 100-fold lower than either epoxybutene or epoxybutanediol. There was no evidence that epoxybutene or diepoxybutane could induce UDS in rat or mouse hepatocytes in vitro. Both epoxybutene and diepoxybutane are genotoxic agents in somatic cells in vivo in studies in the mouse and/or hamster and in the somatic and germ cells of mice and rats in vivo.
Applicant's summary and conclusion
- Executive summary:
The main route for the absorption of 1,3 -butadiene is via the lungs. Oral and dermal absorption are negligble.
The main metabolic pathway for 1,3 -butadiene is described.
Information on Registered Substances comes from registration dossiers which have been assigned a registration number. The assignment of a registration number does however not guarantee that the information in the dossier is correct or that the dossier is compliant with Regulation (EC) No 1907/2006 (the REACH Regulation). This information has not been reviewed or verified by the Agency or any other authority. The content is subject to change without prior notice.
Reproduction or further distribution of this information may be subject to copyright protection. Use of the information without obtaining the permission from the owner(s) of the respective information might violate the rights of the owner.
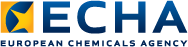