Registration Dossier
Registration Dossier
Data platform availability banner - registered substances factsheets
Please be aware that this old REACH registration data factsheet is no longer maintained; it remains frozen as of 19th May 2023.
The new ECHA CHEM database has been released by ECHA, and it now contains all REACH registration data. There are more details on the transition of ECHA's published data to ECHA CHEM here.
Diss Factsheets
Use of this information is subject to copyright laws and may require the permission of the owner of the information, as described in the ECHA Legal Notice.
EC number: 215-202-6 | CAS number: 1313-13-9
- Life Cycle description
- Uses advised against
- Endpoint summary
- Appearance / physical state / colour
- Melting point / freezing point
- Boiling point
- Density
- Particle size distribution (Granulometry)
- Vapour pressure
- Partition coefficient
- Water solubility
- Solubility in organic solvents / fat solubility
- Surface tension
- Flash point
- Auto flammability
- Flammability
- Explosiveness
- Oxidising properties
- Oxidation reduction potential
- Stability in organic solvents and identity of relevant degradation products
- Storage stability and reactivity towards container material
- Stability: thermal, sunlight, metals
- pH
- Dissociation constant
- Viscosity
- Additional physico-chemical information
- Additional physico-chemical properties of nanomaterials
- Nanomaterial agglomeration / aggregation
- Nanomaterial crystalline phase
- Nanomaterial crystallite and grain size
- Nanomaterial aspect ratio / shape
- Nanomaterial specific surface area
- Nanomaterial Zeta potential
- Nanomaterial surface chemistry
- Nanomaterial dustiness
- Nanomaterial porosity
- Nanomaterial pour density
- Nanomaterial photocatalytic activity
- Nanomaterial radical formation potential
- Nanomaterial catalytic activity
- Endpoint summary
- Stability
- Biodegradation
- Bioaccumulation
- Transport and distribution
- Environmental data
- Additional information on environmental fate and behaviour
- Ecotoxicological Summary
- Aquatic toxicity
- Endpoint summary
- Short-term toxicity to fish
- Long-term toxicity to fish
- Short-term toxicity to aquatic invertebrates
- Long-term toxicity to aquatic invertebrates
- Toxicity to aquatic algae and cyanobacteria
- Toxicity to aquatic plants other than algae
- Toxicity to microorganisms
- Endocrine disrupter testing in aquatic vertebrates – in vivo
- Toxicity to other aquatic organisms
- Sediment toxicity
- Terrestrial toxicity
- Biological effects monitoring
- Biotransformation and kinetics
- Additional ecotoxological information
- Toxicological Summary
- Toxicokinetics, metabolism and distribution
- Acute Toxicity
- Irritation / corrosion
- Sensitisation
- Repeated dose toxicity
- Genetic toxicity
- Carcinogenicity
- Toxicity to reproduction
- Specific investigations
- Exposure related observations in humans
- Toxic effects on livestock and pets
- Additional toxicological data

Endpoint summary
Administrative data
Link to relevant study record(s)
- Endpoint:
- basic toxicokinetics in vivo
- Adequacy of study:
- disregarded due to major methodological deficiencies
- Reliability:
- 3 (not reliable)
- Rationale for reliability incl. deficiencies:
- other: see 'Remark'
- Remarks:
- The methodology lacks details necessary to assess the scientific integrity. The authors had no explanations for the absence of behavioural or neurological signs in the treated monkeys even though they recorded 60-80% increases in manganese concentrations in the brain. This publication was very unclear as to the composition of the administered dose, for example, the methods refer to manganese dust, the table titles manganese dioxide and within the tables MnO. The study design also appears to have been severely lacking in the basic blood manganese monitoring during the study, particularly as there was no other apparent mechanism in place to confirm the dose delivery. The manganese analysis that was performed on the brains took place a year after the study termination, no reason given. Overall, there appears to have been a lack of attention to the actual dose composition, the effectiveness of the administration and any measurement of whether or how much dose was actually absorbed.
- Objective of study:
- other: distribution (brain)... (see attached file)
- Qualifier:
- no guideline followed
- Principles of method if other than guideline:
- Four female Rhesus monkeys were exposed to Mn dust for 6 hours per day, five days a week, for two years. After two years, the animals were sacrificed and the levels of Mn and dopamine in various regions of the brains were compared to controls.
- GLP compliance:
- no
- Species:
- monkey
- Strain:
- other: Rhesus (Macaca Mullata)
- Sex:
- female
- Details on test animals or test system and environmental conditions:
- TEST ANIMALS
- Weight at study initiation: 2 - 3 kg
- Housing: animals were placed individually in exposure chambers during exposure and returned to regular cages (in groups of two) after each six hour exposure period
- Diet: normal diet - Route of administration:
- inhalation: dust
- Details on exposure:
- TYPE OF INHALATION EXPOSURE: whole body
GENERATION OF TEST ATMOSPHERE / CHAMPER DESCRIPTION
- Exposure apparatus: inhalation chambers (52 cu. ft)
Mn dust was fed into the inhalation chamber by a Wright dust feed mechanism and then circulated throughout the chamber by a special exhaust blower providing 15 changes of air per hour.
Chambers were washed down each day after exposure, and the outlet filter removed and changed. - Duration and frequency of treatment / exposure:
- 6 hour exposure, 5 days a week, for 2 years
- Remarks:
- Doses / Concentrations:
30 mg/m³ - No. of animals per sex per dose / concentration:
- 4
- Control animals:
- yes
- Details on dosing and sampling:
- PHARMACOKINETIC STUDY (Absorption, distribution, excretion)
- Tissues sampled: brain (caudate, putamen, globus pallidus and substantia nigra)
- Time and frequency of sampling: at the end of the exposure period (2 years)
TERMINAL PROCEDURES
After two years of exposure, all animals were lightly anethetised with pentobarbital and sacrificed by guillotine. The brains were removed immediately and placed on chipped ice for disection. The caudate, putamen, globus pallidus and substantia nigra were removed, and each area was finely chopped, mixed, and divided into two equal aliquots. One aliquot from each area was frozen immediately for subsequent dopamine assay, and the other aliquot was dried in a desiccator for Mn measurement. The frozen specimens were assayed two weeks later. Mn was assayed by destructive neutron activation analysis one year later. - Metabolites identified:
- no
- Conclusions:
- Interpretation of results: bioaccumulation potential cannot be judged based on study results
Due to the uncertain test material identity, no conclusions can be drawn. - Executive summary:
Four female Rhesus monkeys were exposed to Mn dust for 6 hours per day, five days a week, for two years. After two years, the animals were sacrificed and the levels of Mn and dopamine in various regions of the brains were compared to controls.
Monkeys exposed to Mn dust did not produce any signs of abnormal behaviour or abnormal neurological signs. There were no unscheduled deaths. Analysis of the brains of the monkeys revealed significant decreases in Dopamine (DA) concentration in caudate and globus pallidus, and a 60-80% increase in Mn concentration in the basal ganglia of the brain.
The methodology lacks details necessary to assess the scientific integrity. The authors had no explanations for the absence of behavioural or neurological signs in the treated monkeys even though they recorded 60-80% increases in manganese concentrations in the brain. This publication was very unclear as to the composition of the administered dose, for example, the methods refer to manganese dust, the table titles manganese dioxide and within the tables MnO. The study design also appears to have been severely lacking in the basic blood manganese monitoring during the study, particularly as there was no other apparent mechanism in place to confirm the dose delivery.
The manganese analysis that was performed on the brains took place a year after the study termination, no reason given. Overall, there appears to have been a lack of attention to the actual dose composition, the effectiveness of the administration and any measurement of whether or how much dose was actually absorbed. Due to the uncertain test material identity, no conclusions can be drawn and the report is disregarded.
- Endpoint:
- basic toxicokinetics in vivo
- Type of information:
- experimental study
- Adequacy of study:
- disregarded due to major methodological deficiencies
- Reliability:
- 3 (not reliable)
- Rationale for reliability incl. deficiencies:
- other: see 'Remark'
- Remarks:
- Very brief methodology. Even though the text stated that the lungs from treated rats retained as high as 12-fold the normal lung content of manganese, the table of results showed: control lungs 33.2±5.2 manganese/g and treated lungs 30.3±5.1 manganese/g which contradicts the text. Furthermore, the animals were dosed by intratracheal injection which is not relevant in relation to exposure by inhalation. As such, the reliability of the data is compromised.
- Objective of study:
- other: Absorption and distribution in organs and enzyme effects
- Qualifier:
- no guideline followed
- Principles of method if other than guideline:
- Adult male Wistar rats were intratracheally given a single dose of MnO2 dust in saline and sacrificed after 30 days. The lungs were removed, one part was used for histopathological studies, the other used for enzyme activity assays. Other tissues were removed and observed for manganese distribution.
- GLP compliance:
- no
- Radiolabelling:
- no
- Species:
- rat
- Strain:
- Wistar
- Sex:
- male
- Details on test animals or test system and environmental conditions:
- TEST ANIMALS
- Weight at study initiation: 150±5g
- Housing: Air conditioned rooms.
- Diet: Standard pellet diet
- Water: Ad libitum - Route of administration:
- intratracheal
- Vehicle:
- physiological saline
- Details on exposure:
- PREPARATION OF DOSING SOLUTIONS:
50 mg of dust suspension in 1 mL saline. Control animals received 1 mL saline only. - Duration and frequency of treatment / exposure:
- A single dose, animals were exposed for 30 days (the end of which they were sacrificed for analysis)
- Remarks:
- Doses / Concentrations:
50 mg dust suspension of manganese dioxide in 1 mL saline. - No. of animals per sex per dose / concentration:
- 10 animals inoculated with test material, and ten control rats.
- Control animals:
- yes, concurrent vehicle
- Details on dosing and sampling:
- PHARMACOKINETIC STUDY
- Tissues and body fluids sampled : Brain, blood, liver, kidney, lung, pancreas, testis - Conclusions:
- Interpretation of results: bioaccumulation potential cannot be judged based on study results
The activities of enzymes isolated from the lungs of rats dosed (it) for 30 days with manganese dioxide dust were not significantly altered, even though tissues remote from the lungs showed significant increases in manganese content compared to controls. - Executive summary:
Adult male Wistar rats were intratracheally given a single dose of MnO2 dust in saline and sacrificed after 30 days. The lungs were removed, one part was used for histopathological studies, the other used for enzyme activity assays. Other tissues were removed and observed for manganese distribution.
Histologically the lungs of most of the animals in the experimental group revealed normal structure of the pulmonary tissue with negligible amount of dust discernible. In some animals, small deposits of dusts persisted and cellular nodules composed of mononuclear cells, predominantly macrophages and thin reticulin fibres developed. The control animals did not reveal any comparable histologic change.
Even though the text stated that the lungs from treated rats retained as high as 12-fold the normal lung content of manganese, the table of results showed: control lungs 33.2±5.2 manganese/g and treated lungs 30.3±5.1 manganese/g which contradicts the text. Furthermore, the animals were dosed by intratracheal injection which is not relevant in relation to exposure by inhalation. As such, the reliability of the data is compromised and the data were disregarded.
- Endpoint:
- basic toxicokinetics in vivo
- Adequacy of study:
- disregarded due to major methodological deficiencies
- Reliability:
- 3 (not reliable)
- Rationale for reliability incl. deficiencies:
- other: see 'Remark'
- Remarks:
- Whilst small-particle MnO2 exposure resulted in an elevation in olfactory bulb manganese concentration, the effect was highly variable. Other results did not reach statistical significance and there was no evidence of olfactory nerve MnO2 uptake in rats receiving the large particle exposure. As such, the data from this study are not considered to significantly contribute to the toxicokinetic review of manganese.
- Objective of study:
- absorption
- distribution
- Qualifier:
- no guideline followed
- Principles of method if other than guideline:
- Male rats were exposed in nose only exposure chambers to manganese dioxide aerosols of 1.3 and 18 µm MMAD for 15 days (5 days a week for 3 weeks); rats were dosed at t 3 mg Mn/m³. Following exposure the rats were sacrificed and tissues harvested for manganese determination.
- GLP compliance:
- not specified
- Radiolabelling:
- no
- Species:
- rat
- Strain:
- Long-Evans
- Sex:
- male
- Details on test animals or test system and environmental conditions:
- TEST ANIMALS
- Age at study initiation: 90 days
- Diet: ad libitum (except during exposure)
- Water: ad libitum (except during exposure)
ENVIRONMENTAL CONDITIONS
- Temperature (°C): 21 ± 1 °C
- Photoperiod (hrs dark / hrs light): 12 hours dark / 12 hours light - Route of administration:
- inhalation: aerosol
- Details on exposure:
- TYPE OF INHALATION EXPOSURE: nose only
GENERATION OF TEST ATMOSPHERE / CHAMPER DESCRIPTION
- Exposure apparatus: Jaeger-NYU nose-only exposure apratus
TEST ATMOSPHERE
- MMAD (Mass median aerodynamic diameter) / GSD (Geometric st. dev.):
> large-particle aerosol: 18 µm MMAD (GSD: 1.6)
> small-particle aerosol: 1.3 µm MMAD (GSD: 1.6) - Duration and frequency of treatment / exposure:
- 3 weeks (5 days per week)
- Remarks:
- Doses / Concentrations:
4.75 mg/m³ as manganese dioxide (MnO2); 3 mg/m³ as manganese (MMADs of 1.3 and 18 µm per dose groups, repectively). Control animals received clean air only. - No. of animals per sex per dose / concentration:
- 6 males per group
- Control animals:
- yes, sham-exposed
- Details on dosing and sampling:
- TERMINAL PROCEDURES
At the conclusion of exposure, the rats were anaesthetised and euthanised. Blood, lung, and liver were harvested to provide an index of manganese uptake via non-olfactory routes. The brain was harvested and dissected into the following regions: olfactory bulb, cortex, BG, cerebellum, diencephalon, and brainstem.
Measurement of manganese concentration in tissues was by graphite furnace atomic absorption spectrometry. Blood samples were analysed using the method of Parsons and Slavin. - Conclusions:
- Interpretation of results: bioaccumulation potential cannot be judged based on study results
The current data do suggest that for large particles which are likely to deposit in the nasopharynx that olfactory nerve transport to the brain is not likely to account for significant transport. - Executive summary:
Male rats were exposed in nose only exposure chambers to manganese dioxide aerosols of 1.3 and 18 µm MMAD for 15 days (5 days a week for 3 weeks); rats were dosed at t 3 mg Mn/m³. Following exposure the rats were sacrificed and tissues harvested for manganese determination.
Small-particle MnO2 exposure resulted in an elevation in olfactory bulb manganese concentration, presumably through uptake by the olfactory nerve, but the effect was highly variable. While small increases in cortical and neostriatal manganese levels were also observed in these rats, they did not reach statistical significance. By contrast, there was no evidence of olfactory nerve MnO2 uptake in rats receiving the large-particle exposure.
The current data do suggest that for large particles which are likely to deposit in the nasopharynx that olfactory nerve transport to the brain is not likely to account for significant transport.
- Endpoint:
- basic toxicokinetics in vivo
- Type of information:
- experimental study
- Adequacy of study:
- weight of evidence
- Reliability:
- 2 (reliable with restrictions)
- Rationale for reliability incl. deficiencies:
- other: see 'Remark'
- Remarks:
- A very well designed and documented study. A clear hypothesis is tested and the results thoroughly analysed and discussed. Restrictions - no claims that the study had been conducted and reported according to internationally accepted guidelines or in compliance with the principles of GLP.
- Objective of study:
- absorption
- distribution
- Qualifier:
- no guideline followed
- Principles of method if other than guideline:
- Different groups of adult male rats received either MnCl2.4H2O or MnO2 once a week for 4 weeks at a dose of 24.3 mg Mn/kg body wt. (b.w.) by oral gavage (g.) or 1.22 mg Mn/kg b.w. by intraperitoneal injection (i.p.) or intratracheal instillation (i.t.). Four days after the last administration the rats were killed and the concentration of manganese measured in blood, hepatic and cerebral tissues (cortex, cerebellum, and striatum).
- GLP compliance:
- not specified
- Radiolabelling:
- no
- Species:
- rat
- Strain:
- Sprague-Dawley
- Sex:
- male
- Details on test animals or test system and environmental conditions:
- TEST ANIMALS
- Age at study initiation: 3 months old
- Weight at study initiation: body wt. 350±450 g
- Housing: housed in an air-conditioned room
- Diet: Fed ad libitum a conventional rat chow diet
- Water: Free access to tap water
ENVIRONMENTAL CONDITIONS
- Temperature (°C): 22 ±2 °C,
- Humidity (%): relative humidity 50 ± 10%
- Photoperiod (hrs dark / hrs light): 12:12 h light/dark cycle - Route of administration:
- other: oral gavage, intraperitoneal injection and intratracheal instillation
- Vehicle:
- physiological saline
- Details on exposure:
- PREPARATION OF DOSING SOLUTIONS:
The MnCl2 solutions and MnO2 suspensions were prepared in 0.9% saline and the pH adjusted to between 6.0 and 6.5 with 0.1 M NaOH. Immediately before use, the preparations were autoclaved and sonicated. During administration, the MnO2 preparations were permanently sonicated and stirred which enabled a reproducible sampling of the aliquots to be administered. - Duration and frequency of treatment / exposure:
- once a week for 4 weeks
- Remarks:
- Doses / Concentrations:
MnCl2, 4H2O or MnO2 at doses of 24.3 mg Mn/kg body wt. (b.w.) by oral gavage (g.) or 1.22 mg Mn/kg b.w. for intraperitoneal injection (i.p.) and intratracheal instillation (i.t.) - No. of animals per sex per dose / concentration:
- 6 rats per dose group
- Control animals:
- yes, concurrent vehicle
- Details on study design:
- - Dose selection rationale: The dose groups were selected on the basis of preliminary experiments which demonstrated the absence of organ toxicity induced by the treatments that might have influenced the absorption rate.
- Details on dosing and sampling:
- PHARMACOKINETIC STUDY
- Tissues and body fluids sampled: blood, hepatic and cerebral tissues (cortex, cerebellum, and striatum).
- Time and frequency of sampling: Four days after the last administration the rats were killed and the tissues were sampled. - Statistics:
- Student's t-test was used for comparison of the means of unpaired data. For multiple comparison ANOVA complemented with Dunnett's or Duncan's tests was performed. The level of statistical significance was taken as P < 0.05.
- Preliminary studies:
- A four week observation for signs of toxicity was used as a preliminary study to determine dose selection.
- Details on absorption:
- MnO2 given orally did not significantly increase blood and cerebral tissue Mn concentrations; the low bioavailability is most likely due to the lack of intestinal resorption. Administration of MnO2 i.p. and i.t., however, led to significant increases of Mn concentrations in blood and cerebral tissues. These increments were not significantly different from those measured after MnCl2 administration, except for striatal Mn after i.t. which was markedly less (48%) after MnO2 administration. The liver Mn concentration was not affected by any of the administered treatments. Rats treated i.t. with MnCl2 showed an elective increase of the striatal Mn concentration (205%).
- Details on distribution in tissues:
- Mn concentrations were significantly increased in the cerebellum (31%), striatum (48%) and cortex (34%) following repeated i.t. installation of MnO2, however no increases in Mn concentration in brain tissues was seen after repeated administration by gavage. A comparison of the blood Mn kinetics immediately after g. and i.t. treatment with MnCl2 or MnO2 indicated that the higher elevation of blood Mn concentration ( > 2000 ng Mn/100 ml) after i.t. administration of MnCl2 could account for the elective uptake of Mn in the striatum observed in repeated dosing experiments. Administration of MnCl2 by g., i.p., and i.t. routes produced equivalent steady-state blood Mn concentrations (about 1000 ng Mn/100 ml), representing increases of 68, 59, and 68% compared with controls, respectively.
It is concluded that the modulation of Mn distribution in brain regions according to the route of administration and the chemical form of the Mn substance may be explained on the basis of different blood Mn kinetics and regional anatomic specificities of the striatal region. - Metabolites identified:
- not measured
- Conclusions:
- Interpretation of results: low bioaccumulation potential based on study results
It is concluded that the modulation of Mn distribution in brain regions according to the route of administration and the chemical form of the Mn compound may be explained on the basis of different blood Mn kinetics and regional anatomic specificities of the striatal region. - Executive summary:
Different groups of adult male rats received either MnCl2.4H2O or MnO2 once a week for 4 weeks at a dose of 24.3 mg Mn/kg body wt. (b.w.) by oral gavage (g.) or 1.22 mg Mn/kg b.w. by intraperitoneal injection (i.p.) or intratracheal instillation (i.t.). Four days after the last administration the rats were killed and the concentration of manganese measured in blood, hepatic and cerebral tissues (cortex, cerebellum, and striatum).
MnO2 given orally did not significantly increase blood and cerebral tissue Mn concentrations; the low bioavailability is most likely due to the lack of intestinal resorption. Administration of MnO2 i.p. and i.t., however, led to significant increases of Mn concentrations in blood and cerebral tissues. These increments were not significantly different from those measured after MnCl2 administration, except for striatal Mn after i.t. which was markedly less (48%) after MnO2 administration. The liver Mn concentration was not affected by any of the administered treatments. Rats treated i.t. with MnCl2 showed an elective increase of the striatal Mn concentration (205%).
Mn concentrations were significantly increased in the cerebellum (31%), striatum (48%) and cortex (34%) following repeated i.t. installation of MnO2, however no increases in Mn concentration in brain tissues was seen after repeated administration by gavage. A comparison of the blood Mn kinetics immediately after g. and i.t. treatment with MnCl2 or MnO2 indicated that the higher elevation of blood Mn concentration ( > 2000 ng Mn/100 ml) after i.t. administration of MnCl2 could account for the elective uptake of Mn in the striatum observed in repeated dosing experiments. Administration of MnCl2 by g., i.p., and i.t. routes produced equivalent steady-state blood Mn concentrations (about 1000 ng Mn/100 ml), representing increases of 68, 59, and 68% compared with controls, respectively.
It is concluded that the modulation of Mn distribution in brain regions according to the route of administration and the chemical form of the Mn substance may be explained on the basis of different blood Mn kinetics and regional anatomic specificities of the striatal region.
- Endpoint:
- basic toxicokinetics in vivo
- Type of information:
- experimental study
- Adequacy of study:
- weight of evidence
- Reliability:
- 2 (reliable with restrictions)
- Rationale for reliability incl. deficiencies:
- other: see 'Remark'
- Remarks:
- Appears to be well designed, described and discussed. Restrictions – no claims that the study had been conducted and reported according to internationally accepted guidelines or in compliance with the principles of GLP. The test material was only one of several manganese substances used in the production plant, as such specific measurements of the actual test material concentrations (dose or biomonitoring) were not undertaken. Only total manganese levels were measured which means these cannot be directly related to the test material levels.
- Objective of study:
- absorption
- excretion
- Principles of method if other than guideline:
- The concentration of airborne manganese in a manganese oxides and salts producing plant were determined via personal sampling. Blood and urine samples from exposed workers were examined for Mn content and compared to non-exposed workers.
- GLP compliance:
- not specified
- Radiolabelling:
- no
- Species:
- human
- Sex:
- male
- Details on test animals or test system and environmental conditions:
- 141 Mn-exposed male workers were an average of 34.3 years old (range: 19-59 range)
104 male workers (who were not exposed to Mn) were an average of 38.4 years old (range: 19-58) - Route of administration:
- other: Inhalation, potential dermal and incidental oral
- Vehicle:
- unchanged (no vehicle)
- Duration and frequency of treatment / exposure:
- Workers were exposed for a mean 7.1 years with a range of 1-19 years
- Remarks:
- Doses / Concentrations:
The airborne manganese levels ranged from 0.07 to 8.61 mg/m³ with an overall mean ± SD and median values of 1.33 ± 0.14 and 0.97 mg/m³ respectively. The geometric mean values amounted to 0.94 mg/m³ and the 95th percentile was 3.30 mg/m³. In the control group, a total number of 22 whole shift air samples were taken at the representative workplaces showing that Mn-air levels ranged from 2 to 52 µg/m³ with mean and median values of 12 and 7 µg/m³ respectively (95th percentile: 29 µg/m³). - No. of animals per sex per dose / concentration:
- 141 exposed workers, 104 unexposed controls
- Control animals:
- yes, concurrent no treatment
- Positive control reference chemical:
- no
- Details on dosing and sampling:
- PHARMACOKINETIC STUDY (Absorption, distribution, excretion)
- Tissues and body fluids sampled : urine, blood
- Time and frequency of sampling: Blood and spot urine tests were taken after workers had a shower at the end of their shift. Twenty nine exposed workers gave blood samples before and after shifts (either morning or afternoon shifts). 13 workers gave urine samples collected at the end and the beginning of a working week, giving 3 days without exposure. - Statistics:
- Students t-test
- Metabolites identified:
- not measured
- Conclusions:
- Interpretation of results: bioaccumulation potential cannot be judged based on study results
In summary, the present study indicates that on an individual basis, the determination of Mn-B and Mn-U is of limited value for the biological monitoring of workers exposed to Mn dust. Nevertheless, on a group basis, the risk of excessive accumulation of Mn may be ascertained by measuring Mn-B.
Likewise recent uptake of Mn is likely to lead to a rapid increase in Mn-U, but the quantitative relationship between these parameters cannot yet be established. - Executive summary:
The concentration of airborne manganese in a manganese oxides and salts producing plant were determined via personal sampling. Blood and urine samples from exposed workers were examined for Mn content and compared to non-exposed workers.
On an individual basis, the determination of Mn-B and Mn-U is of limited value for the biological monitoring of workers exposed to Mn dust. Nevertheless, on a group basis, the risk of excessive accumulation of Mn may be ascertained by measuring Mn-B.
Likewise recent uptake of Mn is likely to lead to a rapid increase in Mn-U, but the quantitative relationship between these parameters cannot yet be established.
- Endpoint:
- basic toxicokinetics in vivo
- Type of information:
- experimental study
- Adequacy of study:
- weight of evidence
- Reliability:
- 2 (reliable with restrictions)
- Rationale for reliability incl. deficiencies:
- other: see 'Remark'
- Remarks:
- Well documented and discussed. Restrictions - no claims that the study had been conducted and reported according to internationally accepted guidelines or in compliance with the principles of GLP. The test material was only one of several manganese substances used in the production plant, as such specific measurements of the actual test material concentrations (dose or biomonitoring) were not undertaken. Only total manganese levels were measured which means these cannot be directly related to the test material levels. Similarly, effects on the lungs, central nervous system, and other biological systems cannot be directly related exclusively to the test material.
- Objective of study:
- toxicokinetics
- Qualifier:
- no guideline followed
- Principles of method if other than guideline:
- A cross-sectional epidemiological study of 141 male subjects exposed to inorganic manganese (Mn) in a manganese oxide and salt producing plant compared to a matched control group of 104 subjects. Their physical and neurological health was examined, along with their medical history. Some biological indices were also investigated.
- GLP compliance:
- not specified
- Radiolabelling:
- no
- Species:
- human
- Sex:
- male
- Route of administration:
- other: Inhalation, potential dermal and incidental oral exposure
- Vehicle:
- unchanged (no vehicle)
- Control animals:
- yes, concurrent no treatment
- Conclusions:
- Interpretation of results: bioaccumulation potential cannot be judged based on study results
In summary, this study demonstrates that time- weighted average exposure to manganese dust ( total dust) of about 1 mg/m³ may still lead to the occurrence of preclinical adverse effects in the lungs and the central nervous system in some workers exposed for less than 20 years.
The current average exposure level of 1 mg Mn/m³ is most likely an overestimation for the exposure intensity in the past, since the production capacity of the plant gradually increased over the last 15 years. Therefore, the critical exposure level of airborne Mn (total dust) might be much lower than 1 mg/m³. The results do not reveal a clear-cut biological limit value for manganese in blood, but since values exceeding those normally found in nonexposed population (95th percentile = 1 µg/100 mL) may still be associated with a slightly increased prevalence of abnormal findings, we are inclined to suggest of 1 µg/ 100 mL as a tentative guide for Mn-B. - Executive summary:
A cross-sectional epidemiological study of 141 male subjects exposed to inorganic manganese (Mn) in a manganese oxide and salt producing plant compared to a matched control group of 104 subjects. Their physical and neurological health was examined, along with their medical history. Some biological indices were also investigated.
The intensity of Mn exposure was moderate as reflected by the airborne Mn levels and the concentrations of Mn in blood (Mn-B) and in urine (Mn-U). A significantly higher prevalence of cough in cold season, dyspnea during exercise, and recent episodes of acute bronchitis was found in the Mn group. Lung ventilatory parameters (forced vital capacity, FVC; forced expiratory volume in one second, FEV1; peak expiratory flow rate, PEFR) were only mildly altered in the Mn group (smokers) and the intensity and the prevalence of these changes were not related to Mn-B, Mn-U, or duration of exposure. There was no synergistic effect between Mn exposure and smoking on the spirometric parameters. Except for a few nonspecific symptoms (fatigue, tinnitus, trembling of fingers, increased irritability), the prevalence of the other subjective complaints did not differ significantly between the control and Mn groups. Psychomotor tests were more sensitive than the standardized neurological examination for the early detection of adverse effects of Mn on the central nervous system (CNS). Significant alterations were found in simple reaction time (visual), audioverbal short term memory capacity, and hand tremor (eye-hand coordination, hand steadiness). A slight increase in the number of circulating neutrophils and in the values of several serum parameters (ie, calcium, ceruloplasmin, copper, and ferritin) was also found in the Mn group. There were no clear-cut dose-response relationships between Mn-U or duration of Mn exposure and the prevalence of abnormal CNS or biological findings. The prevalence's of disturbances in hand tremor and that of increased levels of serum calcium were related to Mn-B. The response to the eye-hand coordination test suggests the existence of a Mn-B threshold at about 1 microgram Mn/100 mL of whole blood. This study demonstrates that a time-weighted average exposure to airborne Mn dust (total dust) of about 1 mg/m³ for less than 20 years may present preclinical signs of intoxication.
In summary, this study demonstrates that time- weighted average exposure to manganese dust ( total dust) of about 1 mg/m³ may still lead to the occurrence of preclinical adverse effects in the lungs and the central nervous system in some workers exposed for less than 20 years.
The current average exposure level of 1 mg Mn/m³ is most likely an overestimation for the exposure intensity in the past, since the production capacity of the plant gradually increased over the last 15 years. Therefore, the critical exposure level of airborne Mn (total dust) might be much lower than 1 mg/m³. The results do not reveal a clear-cut biological limit value for manganese in blood, but since values exceeding those normally found in nonexposed population (95th percentile = 1 µg/100 mL) may still be associated with a slightly increased prevalence of abnormal findings, we are inclined to suggest of 1 µg/ 100 mL as a tentative guide for Mn-B.
- Endpoint:
- basic toxicokinetics in vivo
- Type of information:
- experimental study
- Adequacy of study:
- weight of evidence
- Reliability:
- 2 (reliable with restrictions)
- Rationale for reliability incl. deficiencies:
- other: see 'Remark'
- Remarks:
- Relatively good detailed methodology for an old study. Restrictions – no claims that the study had been conducted and reported according to internationally accepted guidelines or in compliance with the principles of GLP. Limited group sizes (2 or 3) meant no statistical evaluation. No estimates of absorption or elimination of dose performed.
- Objective of study:
- distribution
- Qualifier:
- no guideline followed
- Principles of method if other than guideline:
- Rhesus monkeys were exposed to submicron manganese dioxide dusts at manganese concentrations of 3 mg/m³ and 0.7 mg/m³ for 22 hours a day for 10 months. Body weight, external signs, serum calcium and manganese levels, serum enzyme activities and general blood examinations were performed on the test animals.
- GLP compliance:
- not specified
- Radiolabelling:
- no
- Species:
- monkey
- Strain:
- other: Macaca Mullata
- Sex:
- female
- Details on test animals or test system and environmental conditions:
- TEST ANIMALS
- Source: Of Indian origin
- Weight at study initiation: 3-4 kg
- Housing: Cages within inhalation chamber
- Diet : Commercially available pellet food, bananas, apples, sweet potatoes and cabbage as required
- Acclimation period: 1 month - Route of administration:
- inhalation: dust
- Vehicle:
- unchanged (no vehicle)
- Details on exposure:
- TYPE OF INHALATION EXPOSURE: whole body
GENERATION OF TEST ATMOSPHERE / CHAMBER DESCRIPTION
- Exposure apparatus: Two large test chamber
- Source and rate of air: Stated as fresh air compressed in a pump to create a jet stream
- System of generating particulates/aerosols: Manganese dioxide dust added to a dust generator, stirred with a jet stream in a tower where the finest particles reach the top and are delivered to the exposure chambers
- Method of particle size determination: Microscopy
- Treatment of exhaust air: Venturi scrubber
TEST ATMOSPHERE (if not tabulated)
- Particle size distribution: Almost all particles were less than 2 µm in diameter, 80% no larger than 1 µm in diameter - Duration and frequency of treatment / exposure:
- 22 hours a day for 10 months.
- Remarks:
- Doses / Concentrations:
Group A 3 mg/m³, Group B 0.7 mg/m³ and Group C (control) 0 mg/m³ - No. of animals per sex per dose / concentration:
- 3 animals in the highest dose group, and 2 animals in the control group and the lowest dose group.
- Control animals:
- yes, concurrent no treatment
- Details on dosing and sampling:
- PHARMACOKINETIC STUDY Distribution
- Tissues and body fluids sampled : blood, plasma, serum, brain, bile, organs, tissues and glands
- Time and frequency of sampling: Blood samples were taken once a month, with all other tissues taken at the end of the ten month period - Metabolites identified:
- not measured
- Conclusions:
- Interpretation of results: high bioaccumulation potential based on study results
Apart from accumulation in the sercretory organs, manganese accumulation in the brain was particularly high in the cerebral basal nuclei which is critical in manganese exposure. The critical organ concentration was estimates as 17-20 µg/g (on the dry weight basis) - Executive summary:
Rhesus monkeys were exposed to submicron manganese dioxide dusts at manganese concentrations of 3 mg/m³ and 0.7 mg/m³ for 22 hours a day for 10 months. Body weight, external signs, serum calcium and manganese levels, serum enzyme activities and general blood examinations were performed on the test animals.
There was little or no difference in body weight between groups. Two animals of group A began to exhibit neurologic signs such as mild tremor of the fingers, loss in pinching force and a loss in dexterity of the upper limbs after 3-4 moths. These symptoms did not prove to be progressive.
In the general blood examinations, red blood cells, white blood cells, leukocyte differentials, haemoglobin level, hematocrit value, serum protein and its fractions showed no marked variations. In the serum GOT, GPT activities were increased with a marked increase in the activity of MAO. Serum calcium and magnesium were both slightly increased.
In the organs, serum MAO and adenosine deaminase activities increased slightly. MAO and adenosine deaminase activities were also increased in the liver, adrenal glands, cerebellum and mesencephalon. Adenosine deaminase activity was also increased in each of the areas of the brain.
Manganese levels in almost all the organs and tissues were increased in proportion to the exposure amounts. The levels are expected to be highest in the lungs and lymph nodes close to the pulmonary hilus. Manganese dioxide in quite insoluble, however some is absorbed by the lungs and accumulated in the secretory glands such as the pituitary gland, adrenal glands, submaxillary glands, parotid glands and thyroid glands. Concentrations of manganese was found to be higher in these glands compared to pancreas, liver and kidneys. The accumulation of manganese in the areas of the brain was high in proportion to the exposure amounts, the accumulation in the basal nuclei of the brain (caudate nucleus, pallidum, putamen) being most striking.
Apart from accumulation in the sercretory organs, manganese accumulation in the brain was particularly high in the cerebral basal nuclei which is critical in manganese exposure. The critical organ concentration was estimates as 17-20 µg/g (on the dry weight basis)
- Endpoint:
- basic toxicokinetics in vivo
- Adequacy of study:
- disregarded due to major methodological deficiencies
- Reliability:
- 3 (not reliable)
- Rationale for reliability incl. deficiencies:
- other: see 'Remark'
- Remarks:
- After 8 hours inhalation exposure to MnO2 dust the mean amount of manganese in the GI tract of mice was 5.127 mg. This value was then used to calculate the level of manganese to incorporate into diet (1 mg Mn/g diet) in order to provide comparable levels of manganese exposure to a second group of mice for comparative purposes (behaviour etc.). This calculation appears flawed, as it totally rules out any possibility of uptake from the lungs directly or even if mucocilliary transport of inhaled particles took place whether these would have the same bioavailability as dietary manganese.
- Objective of study:
- toxicokinetics
- Qualifier:
- no guideline followed
- Principles of method if other than guideline:
- Sub-chronic oral neurotoxicity and toxicokinetics (weight of evidence/expert report) Inhalation: Mouse (M) dosed by inhalation (whole-body) to ca. 49 mg Mn/m³ for 12 weeks and then to ca. 85 mg Mn/m³ from wk 13 to 32 (7h/d, 5d/wk). 6 wk post-exposure period. Sacrifices at wk 16, 20, 24, 28 and 32 for tissue Mn concs. Behavioural exams every 2 wks after first 16 wks exposure.
Feeding: male mice received Mn-supplemented, or control diet, for 16 to 30 weeks. Behavioural exams every 2 wks after first 16 wks exposure. - GLP compliance:
- no
- Radiolabelling:
- no
- Species:
- mouse
- Strain:
- Swiss
- Sex:
- male
- Details on test animals or test system and environmental conditions:
- TEST ANIMALS
- Source: West Seneca Laboratories, WEst Seneca, NY, USA
- Age at study initiation: approximately 3 months
- Housing: individually in suspended wire cages
- Diet: Charles River RHM-1000 Maintenance Diet (Agway Inc., Syracuse, NY, USA), ad libitum
- Water: tap water, ad libitum
ENVIRONMENTAL CONDITIONS
- Temperature (°C): 21 ± 1°C
- Photoperiod (hrs dark / hrs light): 12 hours dark / 12 hours light - Route of administration:
- other: Study I: inhalation (whole body); Study II: oral (diet)
- Details on exposure:
- Study I:
TYPE OF INHALATION EXPOSURE: whole body
GENERATION OF TEST ATMOSPHERE / CHAMPER DESCRIPTION
- Exposure apparatus: 0.78 m³ "Rochester-type" inhalation chambers. In the cham,bers the mice were heoused in 10 cm (diameter) x 15 cm cylindrical wire mesh cages
TEST ATMOSPHERE (if not tabulated)
- MMAD (Mass median aerodynamic diameter): 1.5 µm
Study II:
DIET PREPARATION
MnO2 was incorporated into a powdered diet (RMH-3200). The MnO2 was mixed with diet in 5 kg batches in a commercial food mixer (1 mg MnO2 was added per gram of diet) - Duration and frequency of treatment / exposure:
- Study I:
7 hours per day for 5 days a week for 16 to 32 weeks
Study II:
Dietary exposure for 16 to 30 weeks - Remarks:
- Doses / Concentrations:
Study I:
ca. 49 mg Mn/m³ for 12 weeks and then to ca. 85 mg Mn/m³ from wk 13 to 32 (7h/d, 5d/wk)
Study II:
5 mg MnO2/day for 16 to 30 weeks - No. of animals per sex per dose / concentration:
- Study I:
96 animals exposed to test material; 96 animals exposed to clean air
Study II:
64 animals received Mn-supplemented diets; 64 animals received plain diet - Control animals:
- yes
- Positive control reference chemical:
- no
- Metabolites identified:
- not measured
- Conclusions:
- Interpretation of results: bioaccumulation potential cannot be judged based on study results
While no general relationship was obtained between tissue Mn levels and behaviour, selected behavioural measures did correlate with tissue Mn levels. Animals exposed via feeding to comparable Mn levels across the same length of exposure employed in the inhalation study did not demonstrate any significant behavioural alterations. - Executive summary:
In study I, male mice were dosed by inhalation (whole-body) to ca. 49 mg Mn/m³ for 12 weeks and then to ca. 85 mg Mn/m³ from weeks 13 to 32 (7h/d, 5d/wk). Animals surviving to week 32 were exposed to control conditions for a further 6 weeks during which biweekly sampling continued. Animals were sacrificed at week 16, 20, 24, 28 and 32 for analysis of Mn tissue concentrations. Behavioural examinations were conducted every 2 weeks after the first 16 weeks of exposure.
In study II, male mice received Mn-supplemented, or control diet, for 16 to 30 weeks. Behavioural examinations were conducted every 2 weeks after the first 16 weeks of exposure.
In the inhalation study, Mn-exposed animals had significantly higher blood, liver, kidney, lung, cerebrum, cerebellum plus brainstem, and testis Mn levels than control animals. With the exception of the liver, these levels declined with increasing exposure time. No histopathologic effects attributable to Mn-exposure were observed. However, significant overall effects on growth and behaviour were obtained. Specifically, Mn-exposed subjects weighed more, executed more rearings in the open-field, and tended to exhibit longer latencies to enter the open-field. When the post-exposure data were analyzed separately, no significant effects were obtained.
In the feeding study, there was no significant effect of treatment, nor was a significant time by treatment interaction found. Since no significant behavioural differences involving treatment were found, the study was terminated after 30 weeks of exposure.
While no general relationship was obtained between tissue Mn levels and behaviour, selected behavioural measures did correlate with tissue Mn levels. Animals exposed via feeding to comparable Mn levels across the same length of exposure employed in the inhalation study did not demonstrate any significant behavioural alterations.
- Endpoint:
- basic toxicokinetics
- Type of information:
- other: Review article
- Adequacy of study:
- weight of evidence
- Reliability:
- other: 5
- Rationale for reliability incl. deficiencies:
- other: Review article. Publication reviews multiple manganese substances and not just the test material
- Qualifier:
- no guideline required
- Principles of method if other than guideline:
- A review of existing data concerning the differences in manganese dosimetry and the implications in neurotoxicity.
- GLP compliance:
- no
- Conclusions:
- Interpretation of results: bioaccumulation potential cannot be judged based on study results
These studies have dramatically improved our understanding of the health risks posed by manganese by determining exposure conditions that lead to increased concentrations of this metal within the CNS and other target organs. Most individuals are exposed to manganese by the oral and inhalation routes of exposure; however, parental injections and other routes of exposure are important. Interactions between manganese and iron and other divalent elements occur and impact the toxic the toxicokinetic of manganese, especially following oral exposure. The oxidation state and solubility of manganese also influence the absorption, distribution, metabolism, and elimination of manganese. Manganese disposition is influenced by the route of exposure. Rodent inhalation studies have shown that manganese deposited within the nose can undergo direct transport to the brain along the olfactory nerve. Species differences in manganese toxicokinetics and response are recognised with nonhuman primates replicating CNS effects observed in humans while rodents do not. Potentially susceptible populations, such as foetuses, neonates, individuals with compromised hepatic function, individuals with suboptimal manganese or iron intake, and those with other medical states (e.g., pre-parkinsonian state, ageing),may have altered manganese metabolism and could be at greater risk of manganese toxicity. However, the publication does not specifically focus on the test material, but considers manganese from a variety of manganese substances. - Endpoint:
- basic toxicokinetics
- Type of information:
- other: Review article
- Adequacy of study:
- weight of evidence
- Reliability:
- other: 5
- Rationale for reliability incl. deficiencies:
- other: Review article
- Objective of study:
- toxicokinetics
- Qualifier:
- no guideline followed
- Principles of method if other than guideline:
- A review of existing inhalation data of manganese and its risk.
- GLP compliance:
- not specified
- Conclusions:
- Interpretation of results: bioaccumulation potential cannot be judged based on study results
The analyses of the data presented in this manuscript provide some assurance that potentially susceptible subpopulations that inhale manganese particles develop qualitatively similar end-of-exposure brain manganese concentrations when compared with young adult male rats. Thus, an UF of 3 to account for intra-species variations in manganese pharmacokinetics may be sufficient to protect potentially susceptible subpopulations - Endpoint:
- basic toxicokinetics in vivo
- Type of information:
- experimental study
- Adequacy of study:
- weight of evidence
- Reliability:
- other: 5
- Rationale for reliability incl. deficiencies:
- other: Non-pivotal.
- Qualifier:
- no guideline followed
- Principles of method if other than guideline:
- Manganese dioxide subcutaneously injected into monkeys to determine if manganese induces changes in receptors in the brain.
The density of four different receptors and one marker of dopamine uptake sites were analyzed in monkey brains after manganese exposure (0.1 g manganese per month during 26 months, a dose comparable to that workers might inhale in dusty environments) by means of quantitative receptor autoradiography. - GLP compliance:
- not specified
- Radiolabelling:
- no
- Species:
- monkey
- Strain:
- Macaca fascicularis
- Sex:
- male
- Details on test animals or test system and environmental conditions:
- TEST ANIMALS
- Weight at study initiation: 4.5 - 6.0 kg - Route of administration:
- subcutaneous
- Vehicle:
- olive oil
- Remarks:
- Doses / Concentrations:
At each occassion 0.2 g Mn was administered. - No. of animals per sex per dose / concentration:
- 3 animals were treated with test material, 3 animals served as a control
- Control animals:
- yes
- Details on study design:
- - Dose selection rationale: animals received subcutaneous administration of test material at a dose coparable to that workers might inhale in dusty environments.
- Conclusions:
- Interpretation of results: bioaccumulation potential cannot be judged based on study results
The major target cells for the cholinergic interneurons as well as for the GABAergic neurons projecting to the striatum appear to be unaffected by the manganese treatment as ligand binding to muscarinic acetylcholine receptors and GABAA receptors were unaffected. This is a good indication for either a high neuronal selectivity or non-uniform effects on affected cells in the action of manganese on the postsynaptic cells. - Executive summary:
The density of four different receptors and one marker of dopamine uptake sites were analyzed in monkey brains after manganese exposure (0.1 g manganese per month during 26 months, a dose comparable to that workers might inhale in dusty environments) by means of quantitative receptor autoradiography.
The binding of 3H-mazindol to the dopamine uptake sites was reduced by 75% in both the head of the caudate nucleus and putamen, while it remained unchanged in the other regions analyzed. The binding of the D1 receptor ligand 3H-SCH 23,390 was reduced about 45% in the same areas as mazindol binding, while the density of D2 receptors was unaffected. The muscarinic acetylcholine receptors as well as GABAA receptors remained also unchanged in all brain areas analyzed after manganese exposure. Thus the dopaminergic neurons must be considered to be vulnerable to manganese concentrations attainable in the work environment. The results also indicate that postsynaptic structures containing D1 receptors are sensitive while cells containing D2 receptors are either spared or compensated for by up-regulation of the number of receptors on remaining sites.
Thus, the major target cells for the cholinergic interneurons as well as for the GABAergic neurons projecting to the striatum appear to be unaffected by the manganese treatment as ligand binding to muscarinic acetylcholine receptors and GABAA receptors were unaffected. This is a good indication for either a high neuronal selectivity or non-uniform effects on affected cells in the action of manganese on the postsynaptic cells.
- Endpoint:
- basic toxicokinetics in vivo
- Type of information:
- experimental study
- Adequacy of study:
- weight of evidence
- Reliability:
- other: 5
- Rationale for reliability incl. deficiencies:
- other: Non-pivotal
- Qualifier:
- no guideline followed
- Principles of method if other than guideline:
- The neurochemical, histological and neuropsychological effects of manganese oxide were assessed in monkeys that were repetitively injected intraperitoneally with a manganese (IV) oxide suspension in olive oil. The monkeys were left for 1 week to 6 months before they were sacrificed.
- GLP compliance:
- no
- Radiolabelling:
- no
- Species:
- monkey
- Strain:
- Macaca fascicularis
- Sex:
- male
- Details on test animals or test system and environmental conditions:
- TEST ANIMALS
- Weight at study initiation: 3.5 - 4.5 kg
- Diet: pellets (ad libitum) and fruit (every day)
- Water: ad libitum - Route of administration:
- intraperitoneal
- Vehicle:
- olive oil
- Control animals:
- yes, concurrent vehicle
- Metabolites identified:
- not measured
- Conclusions:
- Interpretation of results: bioaccumulation potential cannot be judged based on study results
In conclusion, all the observed effects of the manganese intoxication follow the manganese content in the different regions. It is, however, not possible to definitely rule out the chance that this is circumstantial. The effects were not entirely homogenous in the sense that the measured parameters were equally affected in all the analysed brain regions. Thus, the toxic actions of manganese show a selectivity towards the dopaminergic system and the neurotensin containing neurons. Minor effects on the cholinergic and serotonin systems were observed while the GABA-ergic system appears unaffected, which was supported both by a lack of effect on GAD activity had on GABA content. - Executive summary:
The neurochemical, histological and neuropsychological effects of manganese oxide were assessed in monkeys that were repetitively injected intraperitoneally with a manganese (IV) oxide suspension in olive oil. The monkeys were left for 1 week to 6 months before they were sacrificed for analysis.
Under the conditions of the study all animals developed hyperactive behaviour after about 2 months. About 5 months after the start of the exposure the animals became hypoactive with an unsteady gait, and subsequently an action tremor appeared in some of the animals. The animals lost power in both upper and lower limbs and the movements of the hands and feet were very clumsy. The serum content of manganese rose 10- 40 times during the exposure time and the content in brain was generally increased more than 10 times, with the highest content found in globus pallidus and putamen. The observed neurochemical effects were also largest in globus pallidus and putamen. In these regions there was a considerable depletion of dopamine and 3,4-dihydroxyphenylacetic acid, while the homovanillic acid content remained almost unchanged. A severe neuronal cell loss was observed in globus pallidus but not in other regions. This is in accordance with results from a neuropathological study of a human suffering from chronic manganese poisoning where globus pallidus was devoid of neuronal cells while the content of pigmented cells in substantia nigra was normal. These data suggest a reduction in number of dopaminergic nerve terminals, as the activity of the dopamine synthesizing enzyme DOPA-decarboxylase was also lowered.
In conclusion, all the observed effects of the manganese intoxication follow the manganese content in the different regions. It is, however, not possible to definitely rule out the chance that this is circumstantial. The effects were not entirely homogenous in the sense that the measured parameters were equally affected in all the analysed brain regions. Thus, the toxic actions of manganese show a selectivity towards the dopaminergic system and the neurotensin containing neurons. Minor effects on the cholinergic and serotonin systems were observed while the GABA-ergic system appears unaffected, which was supported both by a lack of effect on GAD activity had on GABA content.
- Endpoint:
- basic toxicokinetics in vivo
- Type of information:
- experimental study
- Adequacy of study:
- weight of evidence
- Reliability:
- 4 (not assignable)
- Rationale for reliability incl. deficiencies:
- other: Methodology lacking, no data on timings of blood and urine sampling for example. However, this is an important study as it was using real human occupational exposures.
- Objective of study:
- toxicokinetics
- Qualifier:
- no guideline available
- Principles of method if other than guideline:
- A study of a cohort of workers from five different plants, either milling manganese ore or preparing manganese dioxide for dry cell batteries to determine a threshold value for manganese exposure.
- GLP compliance:
- no
- Species:
- human
- Sex:
- male
- Route of administration:
- inhalation: dust
- Vehicle:
- unchanged (no vehicle)
- Control animals:
- yes
- Conclusions:
- Interpretation of results: bioaccumulation potential cannot be judged based on study results
Manganese concentrations in air correlated significantly with manganese concentrations in urine (r = 0.77) and blood (r = 0.69). At the TLV of 5 mg/m³ the corresponding blood and urine manganese concentrations were both around 30 µg/Litre. - Executive summary:
Workers from two manganese ore milling plants and three dry-cell battery manufacturing plants were studied to determined the extent of absorption and exposure of workers to manganese dioxide. An attempt was made to determine the usefulness of blood and urine estimations as biological indicators of exposure.
The highest manganese exposures were in the manganese mills. Fifteen of the twenty-eight samples collected exceeded the Threshold Limit Value (TLV). The battery factories had significantly lower exposure levels.
No cases of manganese poisoning (manganism) were observed. However four workers from the manganese mills had urine manganese concentrations exceeding the biological TLV of 50 µg/Litre. The blood manganese concentration of all the workers were below the biological TLV of 300 µg/Litre with an average (meang) of 22.59 µg/Litre.
Manganese concentrations in air correlated significantly with manganese concentrations in urine (r = 0.77) and blood (r = 0.69). At the TLV of 5 mg/m³, the corresponding blood and urine manganese concentrations were both around 30 µg/Litre.
- Endpoint:
- basic toxicokinetics
- Adequacy of study:
- weight of evidence
- Reliability:
- 4 (not assignable)
- Rationale for reliability incl. deficiencies:
- other: Very old study, however does provide estimated clearance data of 54Mn from the lower respiratory tract of dogs following 54MnO2 aerosol exposure. No information on particle size provided.
- Qualifier:
- no guideline followed
- Principles of method if other than guideline:
- Beagle dogs were exposed to aerosols of 54-MnO2 for 30 to 90 minutes and their urine and faeces collected for up to 4 weeks post-exposure. Blood samples were also taken during the first post-exposure day. These samples were analysed by gamma ray radiation in a sodium iodide gamma well counter designed to indicate the degree of absorption or in vivo solubility. Immediately after exposure, and at various times later, measurements were made on the radioactivity remaining in the thorax to evaluate the rate of lower respiratory tract clearance.
- GLP compliance:
- not specified
- Radiolabelling:
- yes
- Species:
- dog
- Strain:
- Beagle
- Sex:
- female
- Details on test animals or test system and environmental conditions:
- TEST ANIMALS
- Weight at study initiation: 10 ± 1 kg
- Housing: individually
- Individual metabolism cages: yes - Route of administration:
- inhalation: aerosol
- Details on exposure:
- TYPE OF INHALATION EXPOSURE: nose only
GENERATION OF TEST ATMOSPHERE / CHAMPER DESCRIPTION
The aerosol of test material was generated by liquid aspiration.
PREPARATION OF TEST MATERIAL SUSPENSION
The manganese dioxide suspension was made from manganese chloride by way of the hydroxide and oxidation of the sesquioxide. The suspension was dialysed 24-48 hours against distilled water to remove the extraneous and diffusable electrolytes. Before aerolosation, the suspension was placed in a homogeniser. - Duration and frequency of treatment / exposure:
- Dogs were given single exposures from 30 to 90 minutes in duration.
- Remarks:
- Doses / Concentrations:
0.5 ± 0.2 µg/L - No. of animals per sex per dose / concentration:
- 2 females were exposed to test material aerosols
- Control animals:
- no
- Details on dosing and sampling:
- CLEARANCE MEASUREMENTS - IN VIVO COUNTING
Dogs were positioned in a steel vault such that sodium iodide crystams were adjacent to the lateral chest walls; the output of these counters was fed into a multichannel analyser. Photopeak analyses of the 835 keV gamma of manganese-54 collected by the multichannel analyser, were performed and then linearised. - Metabolites identified:
- not measured
- Conclusions:
- Interpretation of results: bioaccumulation potential cannot be judged based on study results
The average biological half-time was determined to be around 38 days which would indicate an exponential form for the lower respiratory tract clearance of MnO2.
Analyses of the blood were inconclusive but the faecal and urinary elimination were consistent with the concept that the major elimination pathway from the lower respiratory tract was into the faeces via trachea and the gastro-intestinal tract. - Executive summary:
Beagle dogs were exposed to aerosols of 54-MnO2 for 30 to 90 minutes and their urine and faeces collected for up to 4 weeks post-exposure. Blood samples were also taken during the first post-exposure day. These samples were analysed by gamma ray radiation in a sodium iodide gamma well counter designed to indicate the degree of absorption or in vivo solubility. Immediately after exposure, and at various times later, measurements were made on the radioactivity remaining in the thorax to evaluate the rate of lower respiratory tract clearance. The experiment on the first dog exposed to 54-MnO2, was carried out for 30 post-exposure days, while the experiment on the second dog exposed to 54-MnO2 was considerably longer; the first stage lasted 60 days and after a 2 week break, it was re-instituted for an additional 8 weeks. Fewer measurements were made for the determination of the clearance half time in the fist experiment. the second experiment, on the other hand, was run for approximately 135 days.
Under the conditions of the study, the average biological half-time was determined to be around 38 days which would indicate an exponential form for the lower respiratory tract clearance of MnO2. Analyses of the blood were inconclusive but the faecal and urinary elimination were consistent with the concept that the major elimination pathway from the lower respiratory tract was into the faeces via trachea and the gastro-intestinal tract.
Referenceopen allclose all
Monkeys exposed to Mn dust did not produce any signs of abnormal behaviour or abnormal neurological signs. There were no unscheduled deaths.
Analysis of the brains of the monkeys revealed significant decreases in Dopamine (DA) concentration in caudate and globus pallidus, and a 60-80% increase in Mn concentration in the basal ganglia of the brain.
Distribution of protein in the lung of normal and MnO2 inoculated rats:
Changes in enzyme activity of the lung in normal and MnO2 inoculated rats:
aµmol/l bP< 0.01 cP< 0.001 dP< 0.05
Histopathological observations: Histologically the lungs of most of the animals in the experimental group revealed normal structure of the pulmonary tissue with negligible amount of dust discernible. In some animals, small deposits of dusts persisted and cellular nodules composed of mononuclear cells, predominantly macrophages and thin reticulin fibres developed. The control animals did not reveal any comparable histologic change.
Recovery of protein: The protein content estimated from the whole homogenate, mitochondrial and post mitochondrial supernatant fractions remained unchanged. |
Small-particle MnO2 exposure resulted in an elevation in olfactory bulb manganese concentration, presumably through uptake by the olfactory nerve, but the effect was highly variable. While small increases in cortical and neostriatal manganese levels were also observed in these rats, they did not reach statistical significance. By contrast, there was no evidence of olfactory nerve MnO2 uptake in rats receiving the large-particle exposure.
Four week administration experiment
No significant effect on body weight gain could be detected after treatment with MnCl2or MnO2by gavage, i.p. or i.t. administration. Neither lethality nor signs of toxicity were noted during these treatments. At the end of the 4-week treatment regimen, histological analyses performed on GI tract (gavage), liver (i.p.), and lung (i.t.) tissues did not show any significant alteration, except for some scattered inflammatory foci (macrophages and mononucleated cells) in the lung of rats dosed with MnO2but not MnCl2. During the preliminary experiments lung toxicity was checked by monitoring the biological parameters of bronchoalveolar lavages 1 day and 7 days after i.t. instillation. No significant effect of a single instillation of 1.22 mg Mn/kg b.w. either as MnCl2or MnO2could be detected on cellular parameters (i.e. total and differential cell counts). Mn concentrations in liver tissue (1500±2000 ng Mn/g tissue wet weight) were not affected after 4 weeks of treatment with MnCl2or MnO2.
MnCl2 cohort
An important finding was obtained at the end of the 4 weeks of treatment, viz., the three routes of administration produced similar steady-state increases of Mn in blood (gavage, 68%,P= 0.010; i.p., 59%,P= 0.089; i.t., 68%,P= 0.064). Treatment by oral gavage did not influence the Mn concentration in cerebellum and striatum, and slightly but significantly increased that in the cortex (22%,P= 0.033). Intraperitoneal injection did not change the Mn concentration in cerebellum, whereas that in striatum and cortex was increased by 34% (P= 0.177) and 36% (P< 0.001), respectively. Intratracheal instillation increased the Mn concentrations in the three subregions of the brain. The increases were significant in the cortex (48%,P= 0.027) and striatum (205%,P< 0.001) and approached the level of statistical significance in cerebellum (27%,P= 0.100). In order to take into account some differences noted between the values measured in the respective control groups (gavage, i.p., i.t.), an additional statistical analysis was performed. All control values were pooled to constitute a single overall control group (n= 3 ´ 6) and the increments of tissue Mn concentration in the MnCl2treated groups were calculated relative to this overall control group. An ANOVA complemented by Dunnett test performed on the increments confirmed the results obtained by the Student's t-test. The effect of the route of administration on the actual concentrations of Mn in cerebral tissues and blood of treated rats was also examined by ANOVA. The multiple range test showed that administration of MnCl2via oral gavage, i.p. injection, or i.t. instillation produced more or less evenly increased concentrations of Mn in cortex or blood, whatever the route of administration. Only rats treated by i.t. instillation of MnCl2showed a selectively increased Mn concentration in the striatum. The Mn concentrations in the cerebellum were higher after i.p. or i.t. administration when compared to oral gavage (P< 0.05).
MnO2 cohort
After 4 weeks, the concentration of Mn in blood was significantly increased when administered by the i.p. and i.t. routes, i.e. 79% (P= 0.001) and 41% (P= 0.030), respectively. Administration by gavage did not increase either blood or brain tissue Mn concentrations. Significantly increased Mn concentrations were, however, found in the three brain subregions after i.p. injection (cerebellum, 40%,P= 0.002; striatum, 124%,P< 0.001; cortex, 67%,P< 0.001) and i.t. instillation (cerebellum, 31%,P< 0.001; striatum, 48%,P= 0.001; cortex, 34%,P= 0.013). To take into account differences between the values measured in the respective control groups (gavage, i.p. and i.t.), the same additional statistical approach as mentioned above for the MnCl2 cohort was applied. The ANOVA and Dunnett test performed on the increments again confirmed the results obtained by the Student's t-test. The effect of the route of administration was examined by ANOVA complemented with the multiple range test. The actual Mn concentrations in the cerebral tissues and blood of the treated rats were found not to be significantly increased when MnO2 was given by gavage. The i.p. and i.t. routes produced similar Mn concentrations in both the cerebellum and the cortex, whereas i.t. administration produced a higher striatal Mn concentration (P= 0.059) compared to the two other routes of administration. The Mn concentration in blood was significantly higher after i.p. compared to i.t. administration.
Comparison between MnCl2 and MnO2 administrations
The effect of the Mn chemical form was studied for each route of administration separately. The comparison (Student's t-test) was performed on the differences between the tissue Mn concentrations in rats (n= 6) treated with either MnCl2or MnO2and their respective control mean values (either gavage, i.p., or i.t.). When compared to MnO2groups, significantly higher increments in cortex and blood Mn concentrations were found after gavage with MnCl2, while i.t. instillation of MnCl2produced a significantly greater increment in the striatum. Intraperitoneal injection of MnCl2did not produce significantly higher increments of Mn concentrations in blood and brain tissues compared to MnO2.
Blood Mn kinetics after a single administration
In animals treated with MnCl2, i.t. instillation of 1.22 mg Mn/kg b.w. was very rapidly followed by a transient rise of the blood Mn concentration. The highest concentration (7050 ng Mn/100 ml) coincided with the first blood sample taken 30 min after dosing with Mn. Although the true peak concentration might occur between 0 and 30 min, it was impossible to sample blood earlier because of experimental constraints. Subsequently, the blood Mn concentrations declined gradually but remained higher than control values (about 500 to 600 ng Mn/100 ml) for at least up to 24 h. By contrast, oral administration of MnCl2(24.3 mg Mn/kg b.w.) produced a transient rise in blood Mn values which was about five times lower compared with the i.t. route of administration. A peak value of 1660 ng Mn/100 ml was reached after 1 h of dosing followed by a rapid return to control values within 12 h. In animals treated with MnO2the rise of blood Mn concentrations was strikingly delayed compared to the observations made with MnCl2 and the amplitude of the response was considerably less pronounced. Similarly to MnCl2, i.t. instillation of MnO2(1.22 mg Mn/kg b.w.) produced higher blood Mn concentrations than application by oral gavage (24.3 mg Mn/kg b.w.). The onset of increased blood Mn concentration occurred 48 to 72 h after i.t. dosing with MnO2and after 168 h a peak value of 1760 ng Mn/100 ml (200% increase) was achieved. A more significant delay (96 to 120 h) was observed after gavage administration of MnO2and the rise in blood Mn concentration after 144 h was only 27% (900 vs 710 ng Mn/100 ml at time 0,P< 0.02). The blood Mn concentration was still slightly increased (830 ng Mn/100 ml) 10 days after dosing but did not significantly differ (P> 0.05) from the control or the t= 0 value. The time course of blood Mn after oral administration of MnO2most likely reflects a slow and limited transfer of Mn2+ from the GI tract into the circulation.
External Manganese Exposure
The airborne manganese levels ranged from 0.07 to 8.61 mg/m³ with overall mean ± SD and median values of 1.33 ± 0.14 and 0.97 mg/m³, respectively. The geometric mean value amounted to 0.94 mg/m³ and the 95thpercentile was 3.30 mg/m³. In the control group, a total number of 22 whole-shift air samples were taken at representative workplaces showing that Mn-air levels ranged from 2 to 52 µg/m³ with mean and median values of 12 and 7 µg/m³, respectively (95thpercentile: 29 µg/m³)
Internal Manganese Exposure:
The internal exposure to manganese substances was estimated by the determination of manganese in blood and in urine. There is a significant shift to higher values in the Mn group (x2 test, P < 0.001). The Mn-B levels ranged from 0.04 to 1.31 µg/100 mL in the control group and from 0.10 to 3.59 µg/100 mL in the Mn-exposed group. The arithmetic (SD) and geometric mean values for Mn-B were 0.57 (0.27) vs 1.36 (0.64) µg/100 mL and 0.49 vs 1.22 µg/100 mL, in the control and Mn-exposed groups, respectively. In the controls, the 95th percentile of Mn-B is 1.02 µg/100 mL. About 50% of the Mn-exposed workers had Mn-B levels above the highest value found in the control group. The Mn-U levels ranged from above the highest value found in the control group. The Mn-U levels ranged from 0.01 to 5.04 µg/g creatinine in the control group and from 0.06 to 140.6 µg/g creatinine in the Mn-exposed group. The arithmetic mean values for Mn-U amounted to 0.30 vs 4.76 µg/g creatinine whereas the geometric mean (SD) values were 0.15 (3.09) vs 1.59 (3.73) µg/g creatinine in the control and Mn-exposed groups respectively. In the controls, the 95th percentile of Mn-U is 0.85 µg/g creatinine. In summary, the average level of Mn-B was at least twice as high in the Mn-exposed workers than in the controls, while their average urinary excretion of manganese showed a tenfold increase over that of the control group.
Twenty-nine exposed workers gave blood samples before and after their workshift. They were either on a morning (n = 17) or afternoon (n = 12) The Mn-B levels ranged from 0.48 to 3.38 µg/100 mL in the preshift blood samples and from 0.40 to 3.70 µg/100 mL in the postshift blood samples, though this was not statistically significant.
From another group of 13 Mn-exposed workers, spot-urine samples were collected at the end on the work week, at which time Mn exposure ceased for 3 days. A second spot-urine sample was collected from the same workers of Tuesday morning just before they resumed work. The urinary excretion of Mn was markedly reduced in all subjects 73 hours after cessation of Mn exposure. The geometric mean of the Mn-U levels amounted to 7.2 µg/g creatinine at the end of the work week and dropped to 1.3 µg/g creatinine after 72 hours without Mn exposure. The biological half-life of Mn in urine is less than 30 hours.
Relationship Between Parameters of External and Internal Exposure to Manganese.
Individuals: The levels of Mn-B (pre- and postshift) and the levels of Mn-air measured with personal air samplers the same day were available for 24 Mn workers. There was no correlation between these parameters. The levels of Mn-U (during shift) together with current Mn-air levels were available for 34 Mn-exposed workers and again there was no correlation. Multiple correlations between Mn-B or Mn-U, on the one hand, and Mn-air and duration of Mn exposure, on the other, did not show and relationship. The total Mn-exposed group (n = 141), there was no correlation between Mn-U and Mn-B or between duration of exposure to Mn and Mn-U or Mn-B.
Groups: The Mn-exposed workers were classified according to three criteria: a) current Mn-exposure at the workplace; b) duration of Mn exposure; and c) subjective estimation of past integrated exposure by the chief foreman.
Workplaces
Eleven different workplaces were identified in the Mn plant. On a group basis there was no correlation between the current Mn pollution at the workplaces and the actual mean levels of Mn in blood, whereas a slight but statistically significant correlation was found with the actual mean levels of Mn in urine (mean Mn-Ug = 0.875 + 0.916 mean Mn-airg; n = 11, r = 0.62, P < 0.05).
Duration of Mn exposure
The group of 141 Mn-exposed workers was divided in four subgroups according to duration of exposure to Mn, ie, <5, 5-9, 10-14,≥15 years. The mean values of Mn-B (arithmetic) and Mn-U (geometric) of each subgroup were calculated. No statistically significant relationship was found between the parameters of internal Mn exposure and duration of exposure in the Mn plant.
Subjective estimation of past integrated exposure
Due to frequent job turnover between the 11 workplaces of the Mn plant, it was impossible to calculate each workers integrated Mn exposure (Mn-air x duration). However on the basis of the chief foreman’s estimation of past Mn exposure of the workers, it was possible to group the workers into six categories graded from 1+ (low exposure) to 6+ (heavily exposed). Among the 141 Mn-exposed workers, 91 had been engaged mainly at jobs belonging to one of the six major groups. There was a significant rank correlation (rs= 0.83, P < 0.05) between the subjective estimation of integrated Mn exposure and the levels of Mn-B (arithmetic mean) but not with Mn-U (geometric mean).
The intensity of Mn exposure was moderate as reflected by the airborne Mn levels and the concentrations of Mn in blood (Mn-B) and in urine (Mn-U). A significantly higher prevalence of cough in cold season, dyspnea during exercise, and recent episodes of acute bronchitis was found in the Mn group. Lung ventilatory parameters (forced vital capacity, FVC; forced expiratory volume in one second, FEV1; peak expiratory flow rate, PEFR) were only mildly altered in the Mn group (smokers) and the intensity and the prevalence of these changes were not related to Mn-B, Mn-U, or duration of exposure. There was no synergistic effect between Mn exposure and smoking on the spirometric parameters. Except for a few nonspecific symptoms (fatigue, tinnitus, trembling of fingers, increased irritability), the prevalence of the other subjective complaints did not differ significantly between the control and Mn groups. Psychomotor tests were more sensitive than the standardized neurological examination for the early detection of adverse effects of Mn on the central nervous system (CNS). Significant alterations were found in simple reaction time (visual), audioverbal short term memory capacity, and hand tremor (eye-hand coordination, hand steadiness). A slight increase in the number of circulating neutrophils and in the values of several serum parameters (ie, calcium, ceruloplasmin, copper, and ferritin) was also found in the Mn group. There were no clear-cut dose-response relationships between Mn-U or duration of Mn exposure and the prevalence of abnormal CNS or biological findings. The prevalence's of disturbances in hand tremor and that of increased levels of serum calcium were related to Mn-B. The response to the eye-hand coordination test suggests the existence of a Mn-B threshold at about 1 microgram Mn/100 mL of whole blood. This study demonstrates that a time-weighted average exposure to airborne Mn dust (total dust) of about 1 mg/m³ for less than 20 years may present preclinical signs of intoxication.
Prevalence of Respiratory Symptoms in Total Population
Influence of smoking and Mn Exposure on Spirometric Parameters*
Mean Reaction Time and Standard Deviation of Simple Reaction Time* (Visual) in Control and Mn-Exposed Workers
Scores of the Audioverbal Short-term Memory Test (15 Words of Rey) in Control and Mn-Exposed Workers.
|
There was little or no difference in body weight between groups. Two animals of group A began to exhibit neurologic signs such as mild tremor of the fingers, loss in pinching force and a loss in dexterity of the upper limbs after 3-4 moths. These symptoms did not prove to be progressive.
In the general blood examinations, red blood cells, white blood cells, leukocyte differentials, haemoglobin level, hematocrit value, serum protein and its fractions showed no marked variations. In the serum GOT, GPT activities were increased with a marked increase in the activity of MAO. Serum calcium and magnesium were both slightly increased.
In the organs, serum MAO and adenosine deaminase activities increased slightly. MAO and adenosine deaminase activities were also increased in the liver, adrenal glands, cerebellum and mesencephalon. Adenosine deaminase activity was also increased in each of the areas of the brain.
Manganese levels in almost all the organs and tissues were increased in proportion to the exposure amounts. The levels are expected to be highest in the lungs and lymph nodes close to the pulmonary hilus. Manganese dioxide in quite insoluble, however some is absorbed by the lungs and accumulated in the secretory glands such as the pituitary gland, adrenal glands, submaxillary glands, parotid glands and thyroid glands. Concentrations of manganese was found to be higher in these glands compared to pancreas, liver and kidneys. The accumulation of manganese in the areas of the brain was high in proportion to the exposure amounts, the accumulation in the basal nuclei of the brain (caudate nucleus, pallidum, putamen) being most striking.
|
Study I:
Mn-exposed animals had significantly higher blood, liver, kidney, lung,
cerebrum, cerebellum plus brainstem, and testis Mn levels than control
animals. With the exception of the liver, these levels declined with
increasing exposure time. No histopathologic effects attributable to
Mn-exposure were observed. However, significant overall effects on
growth and behaviour were obtained. Specifically, Mn-exposed subjects
weighed more, executed more rearings in the open-field, and tended to
exhibit longer latencies to enter the open-field. When the post-exposure
data were analyzed separately, no significant effects were obtained.
Study II:
There was no significant effect of treatment, nor was a significant time
by treatment interaction found. Since no significant behavioural
differences involving treatment were found, the study was terminated
after 30 weeks of exposure.
Most individuals are exposed to manganese by the oral and inhalation routes of exposure; however, parenteral injection and other routes of exposure are important. Interactions between manganese and iron and other divalent elements occur and impact the toxicokinetics of manganese, especially following oral exposure. The oxidation state and solubility of manganese also influence the absorption, distribution, metabolism, and elimination of manganese. Manganese disposition is influenced by the route of exposure. Rodent inhalation studies have shown that manganese deposited within the nose can undergo direct transport to the brain along the olfactory nerve. Species differences in manganese toxicokinetics and response are recognized with non-human primates replicating CNS effects observed in humans while rodents do not. Potentially susceptible populations, such as foetuses, neonates, individuals with compromised hepatic function, individuals with suboptimal manganese or iron intake, and those with other medical states (e.g., pre-parkinsonian state, aging), may have altered manganese metabolism and could be at greater risk for manganese toxicity.
There is increased interest within the scientific community concerning the neurotoxicity of manganese owing in part to the use of methylcyclopentadienyl manganese tricarbonyl (MMT) as a gasoline fuel additive and an enhanced awareness that this essential metal may play a role in hepatic encephalopathy and other neurologic diseases. Neurotoxicity generally arises over a prolonged period of time and results when manganese intake exceeds its elimination leading to increases in brain manganese concentration. Neurotoxicity can occur following high dose oral, inhalation, or parenteral exposure or when hepatobiliary clearance of this metal is impaired. Studies completed during the past several years have substantially improved our understanding of the health risks posed by inhaled manganese by determining exposure conditions that lead to increased concentrations of manganese within the central nervous system and other target organs. Many of these studies focused on phosphates, sulphates, and oxides of manganese since these are formed and emitted following MMT combustion by an automobile. These studies have evaluated the role of direct nose-to brain transport of inhaled manganese and have examined differences in manganese toxicokinetics in potentially sensitive subpopulations (e.g., foetuses, neonates, individuals with compromised hepatic function or sub-optimal manganese intake, and the aged). This manuscript reviews the U.S. Environmental Protection Agency's current risk assessment for inhaled manganese, summarizes these contemporary pharmacokinetic studies, and considers how these data could inform future risk assessments of this metal following inhalation.
The binding of 3H-mazindol to the dopamine uptake sites was reduced by 75% in both the head of the caudate nucleus and putamen, while it remained unchanged in the other regions analyzed. The binding of the D1 receptor ligand 3H-SCH 23,390 was reduced about 45% in the same areas as mazindol binding, while the density of D2 receptors was unaffected. The muscarinic acetylcholine receptors as well as GABAA receptors remained also unchanged in all brain areas analyzed after manganese exposure. Thus the dopaminergic neurons must be considered to be vulnerable to manganese concentrations attainable in the work environment. The results also indicate that postsynaptic structures containing D1 receptors are sensitive while cells containing D2 receptors are either spared or compensated for by up-regulation of the number of receptors on remaining sites.
All animals developed hyperactive behaviour after about 2 months. About 5 months after the start of the exposure the animals became hypoactive with an unsteady gait, and subsequently an action tremor appeared in some of the animals. The animals lost power in both upper and lower limbs and the movements of the hands and feet were very clumsy. The serum content of manganese rose 10- 40 times during the exposure time and the content in brain was generally increased more than 10 times, with the highest content found in globus pallidus and putamen. The observed neurochemical effects were also largest in globus pallidus and putamen. In these regions there was a considerable depletion of dopamine and 3,4-dihydroxyphenylacetic acid, while the homovanillic acid content remained almost unchanged. A severe neuronal cell loss was observed in globus pallidus but not in other regions. This is in accordance with results from a neuropathological study of a human suffering from chronic manganese poisoning where globus pallidus was devoid of neuronal cells while the content of pigmented cells in substantia nigra was normal. These data suggest a reduction in number of dopaminergic nerve terminals, as the activity of the dopamine synthesizing enzyme DOPA-decarboxylase was also lowered.
The highest manganese exposures were in the manganese mills. Fifteen of the twenty-eight samples collected exceeded the Threshold Limit Value (TLV). The battery factories were found to have significantly lower exposure levels. No cases of manganese poisoning (manganism) were observed. However four workers from the manganese mills had urine manganese concentrations exceeding the biological TLV of 50 µg/Litre. The blood manganese concentration of all the workers were below the biological TLV of 300 µg/Litre with an average (meang) of 22.59 µg/Litre.
The average biological half-time was determined to be around 38 days which would indicate an exponential form for the lower respiratory tract clearance of MnO2.
Analyses of the blood were inconclusive but the faecal and urinary elimination were consistent with the concept that the major elimination pathway from the lower respiratory tract was into the faeces via trachea and the gastro-intestinal tract.
Description of key information
Key value for chemical safety assessment
- Bioaccumulation potential:
- no bioaccumulation potential
- Absorption rate - oral (%):
- 5
- Absorption rate - dermal (%):
- 1
- Absorption rate - inhalation (%):
- 5
Additional information
TOXICOKINETIC ASSESSMENT
TEST MATERIAL: Manganese Dioxide (MnO2); (EC Number 215-202-6, CAS Number 1313-13-9)
The test material, manganese dioxide, occurs naturally as the mineral pyrolusite which is the main ore of manganese. It is also present in manganese nodules. Pyrolusite is a soft darkish black/grey/blue amorphous appearing mineral, often with a granular, fibrous or columnar structure. Particle size analysis of manganese dioxide powder has shown that 35% of the particles were smaller than 1 µm diameter.
Absorption
The test material, manganese dioxide, has an exceedingly low water solubility of 4.6 x 10 -5 g/L of manganese in solution at 20.0 oC, which is equivalent to 7.3 x 10-5 g/L of the test material in solution at 20.0 oC based on the manganese content of the test material (Butler and O'Connor, 2009). Manganese dioxide also has an extremely low solubility (0.092 ± 0.016%) in artificial gastric juice (Anderson, 2009). As the oral absorption of even soluble manganese salts is still relatively low, typically less than 5%, this means that manganese dioxide has an exceedingly low potential for substantial oral absorption (<0.01%). In a study investigating the influence of the route of administration and chemical form of manganese in rats, it was concluded that a suspension of manganese dioxide powder had low bioavailability due to the lack of intestinal absorption (Roels, Meiers et al., 1997). The acute oral median lethal dose (LD50) of the test material in the rat was estimated to be greater than 3000 mg/kg bodyweight*. As such the test material has a very low potential for toxicity by oral absorption. As the test material has a very low solubility in water coupled with its physical inorganic nature (powder) means that it is unlikely to be readily absorbed through the skin.
The test material was so poorly solubility (<0.00005%) in artificial alveolar based upon the extractable manganese (Anderson, 2009) that it was below the detection limit for the procedure. Particle size analysis of a sample of manganese dioxide powder showed that approximately 80% of the particles of manganese dioxide powder were < 10 µm diameter (inhalable) and approximately 50% were < 2.5 µm diameter (respirable). As such, manganese dioxide powder has a very high potential to be inhaled and respired due to its particle size distribution. Since the test material is virtually insoluble in artificial alveolar fluid it would not be expected to be readily absorbed by the lungs and is likely to be slowly cleared from the lungs by the mucocilliary elevator into the gastrointestinal (GI) tract. However, animal studies have shown that significant amounts of manganese are still absorbed by the lungs following manganese dioxide exposure, albeit at a much slower rate than from a soluble form of manganese (Roels, Meiers et al. 1997). The absorption of manganese dioxide from the lungs was most probably mediated by peritoneal and alveolar macrophages through phagocytosis. As such, manganese dioxide powder poses a considerable hazard by inhalation due to its particle size distribution and its low solubility which is likely to hinder its clearance.
In conclusion, the test material has a low potential for any absorption by oral ingestion or dermal absorption. However, due to the small particle size, slow absorption and clearance from the lung, and uptake through phagocytosis means that the substance poses a hazard following exposure via the inhalation route.
With respect to absorption values to be used in risk and exposure assessments, those for the soluble manganese salt, manganese chloride, are adopted as a worst case, although the figure for inhalation absorption is adjusted downwards (absence of solubility in Anderson, 2009) to match oral absorption as a worst case (inhalation 5%, oral 5%, dermal 1%).
Metabolism, Distribution and Excretion
The majority of any test material that is ingested orally is likely to pass through the GI tract unchanged and be excreted in the faeces.
It is generally thought that manganese is metabolised by the body in the form of converting manganese from the Mn2+ valance state to the Mn3+ valance state, and that ingested manganese is absorbed as Mn2+ possibly bound to alpha 2-macroglobulin or albumin. In transversing the liver it is removed nearly quantitatively, but a small proportion is oxidised to the Mn3+ valance state, bound to transferrin and enters the circulation to be transported to tissues. The circulating amount of manganese will be controlled by the normal homeostatic mechanism provided by the liver that controls the manganese balance. However, manganese dioxide has manganese in the Mn4+ valance state and there is no specific information on the uptake and metabolism of Mn4+. In addition, test material that is inhaled can be taken up in the lungs, probably by phagocytosis, and thus will enter the blood stream initially bypassing the liver and its hepatic control of manganese homeostasis.
Manganese was widely distributed and evidence of manganese accumulation in the brain was seen following repeated exposure by inhalation (22 hours/day for 10 months) to manganese dioxide dust at a concentration of 3 mg/m3 manganese (Nishiyama, Suzuki et al. 1977). Mild, but definite neurologic signs were reported for 2 out of the 3 monkeys after 3-4 months of exposure.
As such, the test material, manganese dioxide, presents a hazard by inhalation of the powder, whereupon it can be absorbed and widely distributed throughout the body, including the brain, where evidence of neurotoxicity has been seen following repeated high dose exposures in both animals and man.
* The result of this study indicating an LD50 > 3480 mg/kg for MnO2 conflicts directly with the current classification of MnO2 which indicates acutely harmful by the oral route. Given the insoluble nature of MnO2 and low bioavailability due to this, it is easier to believe that MnO2 should not be classified as acutely harmful by the oral route and there is no published data which supports this classification. Although assignment of a reliability of 1 or 2 is not possible for this study, the conclusion from this study, that MnO2 is not acutely harmful by the oral route, is very believable.
Further information
A comprehensive toxicokinetic assessment has been made on manganese and its inorganic compounds, the full report is attached to this endpoint summary (Bounds 2009).
References
Anderson, K. A. (2009). Bioaccessibility of manganese from manganese Materials in Gastric and Lung (Alveolar) Biofluids, Oregon State University.
Bounds, S.V.J (2009). A toxicokinetic assessment for the Registration, Evaluation and Authorisation of Chemicals, Regulation (EC) No. 1907/2006 (REACH), Manganese and it inorganic compounds, Bounds Consulting Ltd.
Butler, R. E. and B. O'Connor (2009). MnO2 (Erachem): Determination of Water Solubility. Project Number :2702/0002, Harlan laboratories Ltd.
Nishiyama, K., Y. Suzuki, et al. (1977). "Biochemical changes and manganese distribution in monkeys exposed to manganese dioxide dust."Tokushima J Exp Med 24 (3-4): 137-45.
Roels, H., G. Meiers, et al. (1997). "Influence of the route of administration and the chemical form (MnCl2, MnO2) on the absorption and cerebral distribution of manganese in rats."Arch Toxicol 71 (4): 223-30.
Information on Registered Substances comes from registration dossiers which have been assigned a registration number. The assignment of a registration number does however not guarantee that the information in the dossier is correct or that the dossier is compliant with Regulation (EC) No 1907/2006 (the REACH Regulation). This information has not been reviewed or verified by the Agency or any other authority. The content is subject to change without prior notice.
Reproduction or further distribution of this information may be subject to copyright protection. Use of the information without obtaining the permission from the owner(s) of the respective information might violate the rights of the owner.
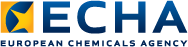