Registration Dossier
Registration Dossier
Data platform availability banner - registered substances factsheets
Please be aware that this old REACH registration data factsheet is no longer maintained; it remains frozen as of 19th May 2023.
The new ECHA CHEM database has been released by ECHA, and it now contains all REACH registration data. There are more details on the transition of ECHA's published data to ECHA CHEM here.
Diss Factsheets
Use of this information is subject to copyright laws and may require the permission of the owner of the information, as described in the ECHA Legal Notice.
EC number: 266-966-2 | CAS number: 67711-90-4 Solids recovered from exhaust gas streams found in furnaces, flues and settling chambers as a result of roasting, smelting and converting operations. Constituents found as end products are dependent upon the materials used during various operations.
- Life Cycle description
- Uses advised against
- Endpoint summary
- Appearance / physical state / colour
- Melting point / freezing point
- Boiling point
- Density
- Particle size distribution (Granulometry)
- Vapour pressure
- Partition coefficient
- Water solubility
- Solubility in organic solvents / fat solubility
- Surface tension
- Flash point
- Auto flammability
- Flammability
- Explosiveness
- Oxidising properties
- Oxidation reduction potential
- Stability in organic solvents and identity of relevant degradation products
- Storage stability and reactivity towards container material
- Stability: thermal, sunlight, metals
- pH
- Dissociation constant
- Viscosity
- Additional physico-chemical information
- Additional physico-chemical properties of nanomaterials
- Nanomaterial agglomeration / aggregation
- Nanomaterial crystalline phase
- Nanomaterial crystallite and grain size
- Nanomaterial aspect ratio / shape
- Nanomaterial specific surface area
- Nanomaterial Zeta potential
- Nanomaterial surface chemistry
- Nanomaterial dustiness
- Nanomaterial porosity
- Nanomaterial pour density
- Nanomaterial photocatalytic activity
- Nanomaterial radical formation potential
- Nanomaterial catalytic activity
- Endpoint summary
- Stability
- Biodegradation
- Bioaccumulation
- Transport and distribution
- Environmental data
- Additional information on environmental fate and behaviour
- Ecotoxicological Summary
- Aquatic toxicity
- Endpoint summary
- Short-term toxicity to fish
- Long-term toxicity to fish
- Short-term toxicity to aquatic invertebrates
- Long-term toxicity to aquatic invertebrates
- Toxicity to aquatic algae and cyanobacteria
- Toxicity to aquatic plants other than algae
- Toxicity to microorganisms
- Endocrine disrupter testing in aquatic vertebrates – in vivo
- Toxicity to other aquatic organisms
- Sediment toxicity
- Terrestrial toxicity
- Biological effects monitoring
- Biotransformation and kinetics
- Additional ecotoxological information
- Toxicological Summary
- Toxicokinetics, metabolism and distribution
- Acute Toxicity
- Irritation / corrosion
- Sensitisation
- Repeated dose toxicity
- Genetic toxicity
- Carcinogenicity
- Toxicity to reproduction
- Specific investigations
- Exposure related observations in humans
- Toxic effects on livestock and pets
- Additional toxicological data

Endpoint summary
Administrative data
Description of key information
Additional information
- 1.15 µg Cu/mm2after7 days T/D tests at pH 6
- 0.19 µg Cu/mm2after7 days T/D tests at pH 7
- 0.13 µg Cu/mm2after7 days T/D tests at pH 8
- 4.2 µg Cu/mm2after 28 days T/D test at pH 6 (excluding one outlier)
- At pH 6 after 7 days: 1.5 µg Cu/mm2* 0.67 mm2/mg * 1 mg/L = 1.0 µg dissolved Cu/L released
- At pH 7 after 7 days: 0.6 µg Cu/mm2* 0.67 mm2/mg * 1 mg/L = 0.4 µg dissolved Cu/L released
- At pH 8 after 7 days: <0.3 µg Cu/mm2* 0.67 mm2/mg * 1 mg/L = <0.2 µg dissolved Cu/L released
- At pH 6 after 28 days: 5.0 µg Cu/mm2* 0.67 mm2/mg * 1 mg/L = 3.4 µg dissolved Cu/L released
- At pH 7 after 28 days: 1.2 µg Cu/mm2* 0.67 mm2/mg * 1 mg/L = 0.8 µg dissolved Cu/L released
- At pH 8 after 28 days: 0.9 µg Cu/mm2* 0.67 mm2/mg * 1 mg/L = 0.6 µg dissolved Cu/L released
- At pH 6, after 7 days: 0.41 µg Cu/mm2* 67 mm2/mg * 1 mg/L = 27.5 µg Cu/L released
- At pH 7, after 7 days: 0.19 µg Cu/mm2* 67 mm2/mg * 1 mg/L = 12.7 µg Cu/L released
- At pH 8, after 7 days: 0.13 µg Cu/mm2* 67 mm2/mg * 1 mg/L = 8.7 µg Cu/L released
- At pH 6, after 28 days: 27.5 µg/L * 4 = 110 µg Cu/L released
- At pH 7, after 28 days: 12.7 µg/L * 4 = 50.8 µg Cu/L released
- At pH 8, after 28 days: 8.7 µg/L * 4 = 34.8 µg Cu/L released
- At pH 6, after 7 days: 1.3 µg Cu/mm2* 9.1 mm2/mg * 1 mg/L = 11.8 µg Cu/L released
- At pH 7, after 7 days: 0.88 µg Cu/mm2* 9.1 mm2/mg * 1 mg/L = 8.0 µg Cu/L released
- At pH 8, after 7 days: 0.45 µg Cu/mm2* 9.1 mm2/mg * 1 mg/L = 4.1 µg Cu/L released
- At pH 6, after 28 days: 5.0 µg Cu/mm2* 9.1 mm2/mg * 1 mg/L = 45.5 µg Cu/L released
- At pH 7, after 28 days: 3.3 µg Cu/mm2* 9.1 mm2/mg * 1 mg/L = 30.0 µg Cu/L released
- At pH 8, after 28 days: 1.9 µg Cu/mm2* 9.1 mm2/mg * 1 mg/L = 17.3 µg Cu/L released
- From an experimental freshwater mesocosm study (Schaefers et al, 2003), carried out with a range of copper loadings, the measured data indicate rapid removal of copper (i.e. 70% in 2 to 8 days);
- For the whole-lake spike addition studies (Lake Courtille), the measured data indicate rapid removal of copper (i.e. 70% in 15 to 17 days) (Van Hullebusch et al., 2002 and 2003);
- For the whole-lake spike addition studies (Saint Germain les Belles Reservoir), the measured data indicate rapid removal of copper (i.e. 70% in 2 to 7 days) (Van Hullebusch et al., 2003);
- For the Hoover Reservoir experiment (Button and Hostetter,1977), the measured data indicate rapid removal of copper (i.e. 70% in 1 hour)
- In a continuous addition experiment (MELIMEX field experiment in a limno coral lake, Switzerland, Gachter and Geiger,1979), copper removal is also observed. Indirect model calculations indicated a half-time of approximately 76 days. The MELIMEX System was characterised by a settling velocity that is 10 times lower than that used in the EUSES system (the model system used in the Copper Voluntary Risk Assessment, 2008) (0.2 versus 2.5 m/d) and a suspended solids concentration that is almost 3 times lower than the EUSES system (5.9 versus 16 mg/L). It is therefore concluded that the MELIMEX study was carried out under extreme conditions, not relevant to classification purposes. It is worth noting that no measurements were made following termination of the copper loading and therefore the assessment is based on modelling.
- For a standard lake environment, consisting of the EUSES model parameters and the Kdderived in the Copper Voluntary Risk Aassessment (2008), copper removal from the water column was 70% in 1 -3 days;
- For a standard lake environment, consisting of the EUSES model parameters but with pH varying between 6 and 8 (Kdestimated from the model), copper removal from the water column satisfies the criterion of rapid removal of 70% in 28 days with maximum depth of 47 m. Also, additional sensitivity analyses, with varying DOC between 2 to 15 mg/L and settling velocities between 0.2 and 2.5 m/d, demonstrate that copper removal from the water column satisfies the criterion of rapid removal of 70% dissolved copper removal in 28 days.
- In one worst case assessment, reducing the settling velocity to 0.2 m/day and using the standard empirical Kd, indicated 70% removal within a period of 33 to 47 days.
Note: this section was copied from the Chemical Safety Report, section 4.0. The format was not maintained during copying, and graphs and tables could not be copied. Therefore, it is recommended that the reader consults the Chemical Safety Report. The transformation-dissolution data are also reported in more detail in the report “The environmental hazard classification of copper”, which is attached to the IUCLID endpoint summary “Ecotoxicological information”
4.0 General summary on environmental fate and pathways
Copper is a natural element and transition metal with more than one oxidation state. Copper in its metallic form (Cu°) is not available. Copper needs to be transformed to its ionic forms (copper ions) to become available for uptake by living organisms. This section summarizes available information relevant to the environmental fate of copper ions. Information is available on the release of soluble copper ions from metallic copper (Cu°) into environmental media (transformation-dissolution tests), on the speciation of copper ions in aqueous media, on removal processes that govern the fate of copper ions in the environment (water, sediments, and soils), on partitioning of copper to suspended particles, and on bioaccumulation.
4.0.1. Stability and Biodegradation
4.0.1.1 Transformation/dissolution of Cu°
Copper metal (Cu°) is insoluble and needs to be transformed to solubilised (dissolved) species in order to be available to the aquatic environment. Therefore, transformation-dissolution tests were conducted according to the GHS protocol (GHS, 6thedition, Annex 10) and are subsequently used for assessing the classification of copper for environmental hazard. The results of the transformation-dissolution tests are described in details in the IUCLID file, section “additional information on fate and pathways”, and are summarized below. The various studies and experiments have different pH (6, 7, or 8), duration (7 or 28 days), copper forms tested (massive copper, coarse powders, or fine powders), experimental set-ups (materials tested as such or embedded in epoxy resin), and loadings (expressed per unit mass or per unit exposed surface area). These studies are mainly used for the classification of the various copper forms for environmental hazards (see Section 7 of the CSR), but are discussed here because they are also supportive evidence for the environmental fate of copper.
Massive copper wires (Rodriguez et al., 2007)
Rodriguez et al. (2007) conducted transformation-dissolution tests using massive copper (pieces of copper wire, 99.9% purity) with varying diameters. The tests were carried out with various mass loadings (1-100 mg/L, corresponding to surface loadings 1.22-281 mm2/L), had durations of 7 and 28 days, and were conducted at pH 6, 7 and 8. These tests demonstrated that copper release from wires with different diameters is determined by the exposed surface area (Figure2). For this reason, the specific surface area approach was adopted for the environmental classification of copper, meaning that the release of copper in transformation-dissolution tests is expressed per unit exposed surface area. This approachavoids issues associated with the selection of a particle type, avoids preparation of particles with a certain size specifically for classification purposes (non-commercial particles), and allows easy and precise measurements of the surface area.
Rodriguez et al. (2007) furthermore found that copper ion releases during the transformation/dissolution tests depend on the stirring rate. At high stirring rates (>50 rpm), copper ion release sharply increases, and high intra- and inter-vessel variability in measured dissolved copper concentrations were observed (Figure3). Considering that this increased release and variability was related to particle abrasion, which should be prevented (GHS rev. 6, 2015, Annex 10, A10.5.1.9), only release rates from tests at 50 rpm are considered.
The copper release during transformation/dissolution tests is pH dependent (Rodriguez et al., 2007). The release is 6 times higher at pH 6 compared to pH 7 and 8 (Figure4). Copper releases during transformation/dissolution tests increase linearly with the surface loading (mm2/L) during the test (Figure4). From tests conducted at surface loadings between 1.2 and 281 mm2/L (corresponding to mass loadings between 1 and 100 mg/L), the average surface–specific copper release (µg dissolved Cu /mm2exposed) are:
Massive copper in epoxy resin (Rodriguez et al., 2011, 2012, and 2017)
In order to obtain better reproducibility, and to ensure consistency with the GHS (stirring at 100 rpm, without causing abrasion of particles, GHS rev. 6, 2015, Annex 10, A10.5.1.9), Rodriguez et al. (2011 and 2012)avoided abrasion by applying specific non-abrasion devices: copper wires mounted in polypropylene disks, and copper massive samples mounted in epoxy resin (Figure5). The exposed surface of the massive samples was polished prior to exposure. The highest copper release per unit surface, and the lowest coefficients of variation, were obtained for copper massive samples mounted in epoxy resin. These values are therefore a conservative choice and are reported below.
Thecopper release measured by Rodriguez et al. (2011) at a surface loading of 47 mm2/L was0.41 µg Cu/mm2after7 days T/D tests at pH 6. The copper release measured by Rodriguez et al. (2012, 2017) at a surface loading of 0.67 mm2/L is summarized inTable24.
From the above results at pH 6, the conclusions from the previous work (Rodriguez et al. 2007) should be slightly nuanced. There is a nearly linear relationship between Cu release and surface loading, but it is not fully linear when low loadings are compared to high loadings. The experiments conducted at high surface loading have a slightly lower copper release per unit surface. A 70-fold difference in surface loading results in a 4-fold difference in copper release per unit surface. A similar trend was also noted by Skeaff and Hardy (2005). For environmental classification, this shows that the most relevant choice is to use transformation-dissolution data obtained at a surface loading that is relevant for the copper form under consideration. Finally, it was noted that the release after 28 days is less than 4 times the copper release after 7 days, most likely indicating the formation of a low solubility copper oxide passivation layer.
Fine copper powder (Skeaff and Hardy, 2005)
Skeaff and Hardy (2005) performed 7-day transformation-dissolution tests on a fine copper powder sample. The specific surface area (SSA) was between 48 mm2/mg measured by sieving and 107 mm2/mg measured by BET. Tests were conducted at mass loadings of 1 and 100 mg/L and at pH 6. Assuming a SSA of 48 mm2/mg, this corresponds to surface loadings (Acalc) of 48 and 4800 mm2/L.
The measured release of copper was 82 µg/L for the mass loading of 1 mg/L (coefficient of variation 32%), and 1118 µg/L for the mass loading of 100 mg/L (coefficient of variation 34%). Skeaff & Hardy noted that the high variability may have been related to abrasion of the particles, which causes increased copper release to solution and should be avoided according to the transformation-dissolution protocol (GHS).
Assuming a SSA of 48 or 107 mm2/mg, the release per unit surface was 1.7 or 0.77 µg Cu/mm2in the experiment with 1 mg/L mass loading, and 0.23 or 0.10 µg Cu/mm2in the experiment with 100 mg/L mass loading. Despite the high uncertainty, it can be observed that the release per unit surface area is somewhat lower at high surface loadings. This agrees with the findings by Rodriguez et al. (2011, 2012) discussed earlier. Given the uncertainty on the SSA of the powder, the variability (high coefficients of variation), and the particle abrasion, these results are not directly for classification purposes, but are shown in order to support the weight of evidence.
Coarse copper powder in epoxy resin (ECTX, 2016)
ECTX (2016) conducted transformation-dissolution studies with a coarse copper powder with specific surface area 2.56 mm2/mg (BET measurement). In a first experiment, the material was tested as such, without anti-abrasion measures (report number FR X01-204). Only one powder particle was added to each vessel in order to attain the desired mass loading of 1 mg/L. This experiment showed a copper release of 1.4 µg/L after 7 days at pH 6 and at a mass loading of 1 mg/L, and 6.0 µg/L after 28 days at pH 6 (coefficients of variation 23 and 27%). Since only eight powder particles were added to each recipient, the exposed surface area was uncertain. This explains the high variability.
In order to decrease the variability, a second test (report number FR X01-203) was set up in which the copper powder particles were embedded in epoxy resin. This allowed to determine the exposed surface area more accurately, it avoids abrasion, and the surfaces were polished before exposure. Results from these experiments, which were conducted at surface loadings of 3.08—3.21 mm2/L, are summarized inTable25. These results had much higher reliability (coefficients of variation only 7—11%) and showed more Cu release than the first experiment. This may be due to the more accurate quantification of the exposed surface, and due to the surface polishing. The results from the second experiment are therefore a conservative choice for using in environmental hazard assessment, and they are preferred over the more variable results from the first experiment. Finally, it can be observed that the copper release after 28 days is approximately four times that after 7 days.
The copper release per unit surface measured by ECTX (2016), for a coarse powder and at surface loadings of 3.08—3.21 mm2/mg, are in good agreement with the results from Rodriguez et al. (2012) for a massive copper sample at surface loading of 0.67 mm2/mg (compareTable24toTable25).
Guiding principles for selection of transformation-dissolution data for environmental hazard classification of copper
Table26presents an overview the available transformation-dissolution data. Several forms of copper are considered, which have different environmental hazard classifications due to their different specific surface area. It is critical to select the appropriate transformation-dissolution data for each copper form. Based on the results from the previous studies, some guiding principles for the selection of appropriate transformation-dissolution data were adopted:
1. Data are preferred if the surface loading in the experiment was within the range of the relevant copper powder. This is in line with the results by Rodriguez et al. (2011 and 2012), which show that the copper release per unit surface slightly depends on the surface loading used (a 70-fold difference in surface loading cause approximately a fourfold difference in copper release per unit surface area).
2. Studies including inter-particle abrasion (Skeaff and Hardy, 2005 and Rodriguez et al., 2007) are considered worst-case, and are only used in the absence of other data.
3. Copper release is highest at pH 6. Therefore, in the absence of data at pH 7 and 8, the data at pH 6 can be used as worst-case estimate.
4. In the absence of 28-day transformation-dissolution tests, as a conservative choice, without considering passivation, a linear release can be assumed, i.e. the release after 28-days is equal to four times that after 7 days.
The column “use for classification” inTable26shows which dataset has been selected for which copper forms.
Selection of transformation-dissolution data for copper massive
For environmental classification, a representative transformation-dissolution dataset should be selected and converted to a copper solubility at a mass loading of 1 mg/L in transformation-dissolution test (as required by the GHS transformation-dissolution protocol and in CLP classification schemes). A transformation-dissolution test with a worst-case copper massive particle (SSA 0.67 mm2/mg), and conducted at a mass loading of 1 mg/L, have a surface loading of 0.67 mm2/L. Based on the guiding principles in the previous section, the transformation-dissolution data collected by Rodriguez et al. (2012 and 2017) are retained for environmental classification of copper massive. These data were conducted at the correct surface loading (0.67 mm2/L, equivalent to a mass loading of 1 mg/L), and abrasion was avoided in these tests.
The surface-based release of these data is subsequently converted to a dissolved Cu concentration at a mass loading of 1 mg/L, by using the SSA of a worst-case copper massive particle (0.67 mm2/mg) (see also Table27):
Selection of transformation-dissolution data for copper powder
For environmental classification, a representative transformation-dissolution dataset should be selected. Then, these surface-based release data (in µg Cu/mm2) are converted to a copper release (in µg Cu/L) in a transformation-dissolution test at a mass loading of 1 mg/L, assuming a worst-case particle of the copper form considered (highest SSA). The resulting value is then used to derive the environmental hazard classification as described in the CLP classification schemes.
Copper powder A (fine)
For copper powder A (SSA above 9.1 mm2/mg),a reasonable worst case, very fine powder with particle size of 10 µm diameter is used as the basis for the environmental classification. Assuming spherical particles, this value corresponds to a SSA of 67 mm2/mg. This SSA is in line with the measurements for a very fine copper powder by different techniques (47—107 mm2/mg, depending on the analytical technique, Skeaff & Hardy, 2005) and is therefore a reasonable worst case value.
Transformation-dissolution tests conducted at a mass loading of 1 mg/L would have surface loadings of 9.1—67 mm2/L. Based on the guiding principles, the transformation-dissolution data collected by Rodriguez et al. (2011) are retained for acute environmental classification of copper powder A at pH 6. These data were conducted at a surface loading within the above range (47 mm2/L;principle 1), and abrasion was avoided. The data by Skeaff & Hardy (2005) have uncertain exposed surface area, high variability, and particle abrasion occurred. Therefore, the data by Rodriguez et al. (2011) are preferred.
At pH 7 and 8, the data from Rodriguez et al. (2007) are selected, since these were obtained at surface loadings between 1.2—281 mm2/L (principle 2). These data are a conservative choice, since the release was partly due to particle abrasion. For the evaluation of the long-term environmental hazard, the above data (obtained after 7 days) are multiplied by four to obtain a conservative estimate of the Cu release after 28 days(principle 4).
The surface-based release is subsequently converted to a dissolved Cu concentration at a mass loading of 1 mg/L, by using the SSA of a representative very fine copper powder A (67 mm2/mg) (see alsoTable26):
Copper powder B (coarse)
For copper powder B (0.67—9.1 mm2/mg), transformation-dissolution tests conducted at a mass loading of 1 mg/L would have surface loadings of 0.67—9.1 mm2/L. Based on the guiding principles, the transformation-dissolution data produced by ECTX (2016) are retained for acute and long-term environmental classification of copper powder B. These tests were conducted at a surface loading within the above range (3.08—3.21 mm2/L;principle 1), and abrasion was avoided (principle 2). Data are available for 7 and 28-days durations and at all pH values.
The surface-based release is subsequently converted to a dissolved Cu concentration at a mass loading of 1 mg/L, by using the SSA of the smallest (worst-case) copper powder B particles (9.1 mm2/mg) (see alsoTable26):
In summary, the transformation-dissolution data show that, in relevant environmental media, only very limited dissolution of copper occurs within 7 or 28 days. In addition, the copper release depends on the exposed surface area. Expressing copper release in transformation-dissolution tests relative to the exposed surface area (i.e. the “specific surface area approach”) therefore is a scientifically sound method to assess copper release in environmental media.
4.0.1.2 Transformation of Cu-ions released in the environment - Copper speciation
Once released to the environment, copper ions have more than one oxidation state and copper is thus characterized as transition metal. The principal ionic forms are cuprous (Cu(I), Cu+) and cupric (Cu(II), Cu2+). The trivalent form (Cu(III), Cu3+) occurs but is relatively unimportant in physical and biological systems. Cu+is unstable in aqueous media and soluble Cu1+compounds readily transforms into soluble Cu2+ions, compounds and/or insoluble Cu2+ions, compounds (eg copper sulfides) that precipitate. This transformation of Cu+to Cu2+is a result of a redox reaction initiated through atmospheric water vapour as well as in aqueous solution. However, monovalent copper cations are only susceptible to such transformation when they are not chemically bound in insoluble compounds or stabilised in complexed forms.
The transformation of Cu(I) to Cu (II) can be described by:
(1) 2 Cu2O + 2H2O = 4Cu++ 4OH-, and
(2) 4Cu++ O2+ 4H+= 4Cu2++ 2H2O
Both sub-reactions are summarised as:
2Cu2O(s) + O2(g) + 4H+= 4Cu2++ 4OH-
Among the copper species released/transformed, Cu (II) is thus the most environmental relevant species. It is further recognised that Cu (II) ions - commonly named free cupric ions- are the most active copper species and that total Cu or Cu(II) concentrations are usually not directly related to ecological effects since exposure of biota may be limited by processes that render Cu unavailable for uptake (ICPS, 1998). Assessing the species of Cu (II) therefore has ecotoxicological relevance. After being released into the environment, the Cu(II) ions typically bind to inorganic and organic ligands contained within water, soil, and sediments. In water Cu(II) binds to dissolved organic matter (e. g., humic or fulvic acids). The Cu(II) ion forms stable complexes with -NH2, -SH, and, to a lesser extent, -OH groups in these organic acids. Cu(II) will also bind with varying affinities to inorganic and organic components in sediments and soils. For example, Cu(II) binds strongly to hydrous manganese and iron oxides in clay and to humic acids, but much less strongly to aluminosilicates in sand. In all environmental compartments (water, sediment, soil), the binding affinities of Cu(II) with inorganic and organic matter is dependent on pH, the oxidation-reduction potential in the local environment, and the presence of competing metal ions and inorganic anions.
Some key papers on copper speciation in freshwater, marine waters, sediments and soils are provided in the section "additional information on environmental fate"
4.0.1.3 Copper attenuation, removal from water column, geochemical cycling
As described above, after the release of Cu(II) as copper ions in the environment, further transformations occur which convert the copper ions into other, nontoxic forms. The concentrations of copper ions that are available for uptake by biota depends on different processes: precipitation, dissolution, adsorption, desorption, complexation and competition for biological adsorption sites (ligands). These processes are critical for the fate of copper in the environment. This was recognized in the Guidance to Regulation (EC) No 1272/2008 Classification, Labelling and Packaging of substances and mixtures (metal annex):
“Environmental transformation of one species of a metal to another species of the same does not constitute degradation as applied to organic compounds and may increase or decrease the availability and bioavailability of the toxic species. However as a result of naturally occurring geochemical processes metal ions can partition from the water column. Data on water column residence time, the processes involved at the water – sediment interface (i.. e. deposition and re-mobilisation) are fairly extensive, but have not been integrated into a meaningful database. Nevertheless, using the principles and assumptions discussed above in Section IV.1, it maybe possible to incorporate this approach into classification. “
The use of laboratory mesocosm and field tests for evaluating removal of soluble metal species through precipitation/partitioning processes over a range of environmentally relevant conditions are described in theguidance (2009) but their acceptance for the CLP guidance (2012) was not conclusive. For copper, such modelling, laboratory, mesocosm and field tests have been assessed. This information is discussed separately for the water, sediment, and soil compartments. The information on rapid removal from the water column is both relevant for environmental fate properties (this section) and for classification for environmental hazards (section 7 of the CSR).
Water compartment
Copper removal rates from the water column have been quantified from laboratory experiments, field experiments, and modelling approaches under a range of environmentally relevant conditions. The experimental data can be summarized as follows:
• Batch incubation studies, which assess metal removal from well-mixed solutions amended with a small amount of sediment material, are ongoing. These experiments address metal removal in relatively simple lab tests (synthetic solutions with added sediment under reasonable worst case conditions). Preliminary results under relatively conservative conditions show that 70% of the copper is removed within 2 days.
These datasets therefore consistently demonstrate removal, and in most cases rapid removal, of copper ions. The fact that removal rates in one study are somewhat slower does not invalidate the conclusion that rapid removal occurs under most environmentally relevant conditions. As analogy, if ”rapid degradation” of an organic substance is concluded from a screening test at a temperature of 20-25°C, then in cold waters degradation rates will surely be slower.
The modelling assessment with the Ticket unit world model (Rader 2013) validated the above findings and allowed to assess more broadly spatial and temporal variability as applicable to European waters. Measured Kdvalues were used to initiate the assessment. Additionally, extensive sensitivity analysis, using WHAM modelling at varying pH, DOC, POC and settling velocities, demonstrated rapid removal due to partitioning/settling in most cases.
• Suspended solids/sediments are present in all functioning ecosystems (rivers and lakes) and scavenge copper from the water-column. The field monitoring data of European surface waters (copper risk assessment report) show that, following continuous weathering of copper from natural systems and anthropogenic uses of copper, dissolved copper concentrations in European surface waters remain generally low, with copper deficiency in open marine waters. Such information is surely consistent with the concept of rapid removal of copper ions in river and lake systems.
From the data, it is therefore concluded that under typical, environmentally relevant conditions, copper ions are rapidly removed from the water column with 70% removal within 28 days. This information is considered relevant to the environmental classification if such removal is accompanied by “irreversible” change in speciation to insoluble/non-available forms.
Sediment compartment
In the sediment compartment,copper binds to the sediment organic carbon (particulate and dissolved) and to the anareobic sulfides, resulting in the formation of CuS. CuS has a very low stability constants/solubility limit (LogK=-41 (Di Toro et al.,1990) – see sectionadsorption/desorption) and therefore the “insoluble” CuS keeps copper in the anaerobic sediment layers, limiting the potential for remobilization of Cu-ions into the water column.
Following the rapid removal from the water-column, evidence on change in speciation to non-soluble, non-available copper sulphides and the “irreversibility” of the formation of such “non-available forms” is available from modelling, laboratory experiments, field experiments, and monitoring data.
Where sediments are deposited, these coincide with the deposition of organic matter. The organic matter is subjected to bacterial mineralisation and therefore linked to a continuous creation of sulphides. The top layer may in some cases indeed be oxic, but this is only a few mm thick and transient. The monitoring data and the equilibrium chemistry confirm that Cu-S precipitates dominate the speciation in sediments, even if other metals are present at considerably higher concentrations. This is due to the high intrinsic binding capacity of Cu relative to other metals. The general presence of sufficient acid volatile sulphide (AVS) to bind copper in European surface waters was clearly demonstrated in the Copper Voluntary Risk Assessment (2008). In all the EU surface waters (rivers and lakes) assessed, more than 90% of the samples has excess AVS.
To examine the potential for remobilization of copper from sediments, a series of 1-year simulations were performed, using the TICKET-UWM. These focused on re-suspension, diffusion, and burial to/from the sediment layer, their net effect on copper concentrations in the water column, and changes in speciation in the sediment. Simulations were made with varying conditions (concentrations of AVS, pH, hardness, suspended solids and solid re-suspension rates). In simulated sediments with AVS present in excess of copper, essentially all copper in sediment was present as copper sulfide because the affinity of copper for sulfides is much larger than that for Organic Carbon. CuS has a very low solubility product constant (Kps) and therefore, full copper sulfide precipitation was generally demonstrated: in all cases where AVS>1 µmol (reasonable worst case AVS concentration in European surface waters) and at environmentally relevant copper concentrations (<0.1mg/L). As a result of this strong binding, the sedimentKdgreatly exceeded the water columnKd.. Therefore, the net diffusive flux of copper was directed into the sediment. For anoxic sediments devoid of AVS, and for oxic sediments, the net diffusive flux was small and directed out of the sediment. However, for all cases considered, the pseudo steady-state total and dissolved copper concentrations were at least 8 times below the concentration required for 70% removal from the water column.
The assessment of two lake experiments with intermittent copper dosing (Lake Courtille and the Saint Germain les Belles Reservoir, Rader, 2013) provides support for the continued flux to the sediments and absence of re-mobilisation. Since both water bodies are shallow, polymictic lakes, wind-driven re-suspension is expected to play a role in copper dynamics in the water column. Nevertheless, even if long-term re-suspension does in fact occur, for both water bodies > 70% removal in less than 28 days was still observed. This therefore supports a continued flux to the sediment and absence of remobilisation from the water column even where re-suspension is observed (Rader, 2013)
Short term re-suspension experiments further demonstrate that re-suspension (high turbidity zones) does not lead to re-mobilisation.
• Simpson et al (1998) investigated the oxidation rates of model metal sulfide phases to provide mechanistic information for interpreting the observations on natural sediments. CuS phases were kinetically stable over periods of several hours.
• Fetters et al, 2014 confirmed that rigorous re-suspension during 4 hours did not lead to re-mobilisation of copper (presented at the Sept 2014 workshop)
Long term irreversibility in river type systems was further evidenced experimentally. Thisprovides field evidence on the stability of the CuS binding:
• Sundelin and Erikson (2001) found evidence that, after long term oxygenation of sediment cores (3 to 7 months), Cu remained comparatively unavailable.
• Costello et al, 2014 observed that, for 2 copper spiked sediments (high and low AVS) exposed continuously to oxygenated water for 213 days in a flow-through river mesocosm, 97—99.7% of the spiked copper remained in the sediment compartment, bound to sulphides and crystalline metal oxides. Sediment porewater Cu concentrations declined throughout the experiment (62 – 83% decline within 21 days). The decline in porewater copper concentrations was consistent with the lower toxicity to Hyallela azteca toxicity as time progressed.
• De Jong et al, 2010 demonstrated that oxygenation of overlying water during 60 days had no effect on Cu DGT-lability from a highly sulfidic (AVS = 230 µmol/g dw) Flemish river sediment contaminated with Cu (110 mg/kg dw).
The data therefore demonstrate that, following partitioning and settling of copper containing solids, the processes at the water-sediment interface continuously ensure the formation of insoluble, unavailable Cu-species (e.g. copper sulphides). Copper remobilisation from lake and river sediments to aqueous phases is not expected, not even during resuspension and oxygenation of anoxic sediments. Cu bioavailability decreases with time in sediments and the oxygenation of anoxic sediments does not increase Cu porewater concentrations, copper lability, or copper toxicity.
The data, therefore, supports the view that that under environmentally relevant conditions, copper ions are rapidly removed from the water-column and the processes involved at the water – sediment interface result in “irreversible” change in speciation to insoluble/non-available forms. This allows us to conclude that copper ions are rapidly removed from the water-column, equivalent to the “biodegradability” of organic substances, and consistent with the metal-specific guidance and the conclusions from the Feb 2012 ECHA workshop.
In summary, from the available evidence on the environmental fate of dissolved copper in the water and sediment compartments, it can be concluded that under “environmentally relevant” conditions, more than 70% of dissolved copper is removed within 28 days. Copper is transformed to sulfide complexes in the sediment (Cu-S) which are stable. Remobilisation of Cu to the water-column is not likely to occur.Copper is therefore considered rapidly removed, conceptually equivalent to “rapid degradation” for organic substances.This information is also used in the context of classification for environmental hazards (section 7 of the CSR).
Soil compartment
In soils,decreases in copper solubility and in copper bio-availability are observed following copper spiking in the laboratory and from long-term field copper exposure experiments. Short term attenuation and long term ageing of copper, spiked in soluble forms to soils was demonstrated from laboratory and field experiments (Ma et al., 2006a and 2006b) and reported in the section “adsorption/desorption”.
The soil environmental factors governing short term attenuation and ageing rates are soil pH, organic matter content, incubation time and temperature with soil pH being the key factor for ageing of Cu added to soils. From a range of laboratory and field experiments an ageing factor of 2 was derived as a reasonable worst case when considering field exposure data.
4.0.2 Transport and distribution
Relevant partitioning coefficients are available from literature.
-Aquatic compartment
Partition coefficient in freshwater suspended matter Kpsusp= 30,246 l/kg (log Kp (pm/w) = 4.48) (50thpercentile)
Partition coefficient in freshwater sediment Kpsed = 24,409 l/kg (log Kp(sed/w) = 4.39) (50th percentile)
Partition coefficient in estuarine suspended matter Kpsusp= 56,234 l/kg (log Kp (pm/w) = 4.75) (50thpercentile)
Partition coefficient in marine suspended matter Kpsusp= 131,826 l/kg (log Kp (pm/w) = 5.12) (50thpercentile)
-Terrestrial compartment
Partitioning coefficient Kd value soil: 2120 L/kg(log Kp (pm/w) = 3.33) (50thpercentile)
4.0.3. Bioaccumulation
Because copper is an essential nutrient, all living organisms have well developed mechanisms for regulating copper intake, copper elimination and internal copper binding. The information in the accumulation section demonstrates that copper is well regulated in all living organisms and that highest/ BAF values are noted when copper concentrations in water, sediments and soils are low and for organisms/ life stages with high nutritional needs. The BCF/BAF values therefore have no ecotoxicological meaning. It should be mentioned that the non-applicability of BCFs for metal and especially for essential metals was already recognized in the regulatory framework of aquatic hazard classification (OECD,2001).
Importantly, the literature review demonstrates that copper is not biomagnified in aquatic or terrestrial ecosystems.
The section further includes critical data related to (1) the accumulation of copper on critical target tissues (eg gills in aquatic organisms); (2) the influence of environmental parameters (eg Organic Carbon, pH, Cationic Exchange Capacity) as well as food intake on the accumulation of copper.
This information is relevant to the understanding of the accumulation as well as the mechanism of actions, described in the section ecotoxicological information
More detailed summaries on respectively aquatic and terrestrial bioaccumulation are available from the aquatic and terrestrial bioaccumulation summary sections
The information relevant to assessing copper toxicity from dietary exposure - of relevance to secondary poisoning assessments is included in the section "ecotoxicological information".
The summary record “ecotoxicological information “ further provides an overall summary of the rationale for the absence of bio-accumulation and no-concern for secondary poisoning (see alos section 7.5.3 – secondary poisoning)
Information on Registered Substances comes from registration dossiers which have been assigned a registration number. The assignment of a registration number does however not guarantee that the information in the dossier is correct or that the dossier is compliant with Regulation (EC) No 1907/2006 (the REACH Regulation). This information has not been reviewed or verified by the Agency or any other authority. The content is subject to change without prior notice.
Reproduction or further distribution of this information may be subject to copyright protection. Use of the information without obtaining the permission from the owner(s) of the respective information might violate the rights of the owner.
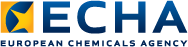