Registration Dossier
Registration Dossier
Data platform availability banner - registered substances factsheets
Please be aware that this old REACH registration data factsheet is no longer maintained; it remains frozen as of 19th May 2023.
The new ECHA CHEM database has been released by ECHA, and it now contains all REACH registration data. There are more details on the transition of ECHA's published data to ECHA CHEM here.
Diss Factsheets
Use of this information is subject to copyright laws and may require the permission of the owner of the information, as described in the ECHA Legal Notice.
EC number: - | CAS number: -
- Life Cycle description
- Uses advised against
- Endpoint summary
- Appearance / physical state / colour
- Melting point / freezing point
- Boiling point
- Density
- Particle size distribution (Granulometry)
- Vapour pressure
- Partition coefficient
- Water solubility
- Solubility in organic solvents / fat solubility
- Surface tension
- Flash point
- Auto flammability
- Flammability
- Explosiveness
- Oxidising properties
- Oxidation reduction potential
- Stability in organic solvents and identity of relevant degradation products
- Storage stability and reactivity towards container material
- Stability: thermal, sunlight, metals
- pH
- Dissociation constant
- Viscosity
- Additional physico-chemical information
- Additional physico-chemical properties of nanomaterials
- Nanomaterial agglomeration / aggregation
- Nanomaterial crystalline phase
- Nanomaterial crystallite and grain size
- Nanomaterial aspect ratio / shape
- Nanomaterial specific surface area
- Nanomaterial Zeta potential
- Nanomaterial surface chemistry
- Nanomaterial dustiness
- Nanomaterial porosity
- Nanomaterial pour density
- Nanomaterial photocatalytic activity
- Nanomaterial radical formation potential
- Nanomaterial catalytic activity
- Endpoint summary
- Stability
- Biodegradation
- Bioaccumulation
- Transport and distribution
- Environmental data
- Additional information on environmental fate and behaviour
- Ecotoxicological Summary
- Aquatic toxicity
- Endpoint summary
- Short-term toxicity to fish
- Long-term toxicity to fish
- Short-term toxicity to aquatic invertebrates
- Long-term toxicity to aquatic invertebrates
- Toxicity to aquatic algae and cyanobacteria
- Toxicity to aquatic plants other than algae
- Toxicity to microorganisms
- Endocrine disrupter testing in aquatic vertebrates – in vivo
- Toxicity to other aquatic organisms
- Sediment toxicity
- Terrestrial toxicity
- Biological effects monitoring
- Biotransformation and kinetics
- Additional ecotoxological information
- Toxicological Summary
- Toxicokinetics, metabolism and distribution
- Acute Toxicity
- Irritation / corrosion
- Sensitisation
- Repeated dose toxicity
- Genetic toxicity
- Carcinogenicity
- Toxicity to reproduction
- Specific investigations
- Exposure related observations in humans
- Toxic effects on livestock and pets
- Additional toxicological data

Endpoint summary
Administrative data
Key value for chemical safety assessment
Effects on fertility
Description of key information
A multigeneration study in the rat (Weir, 1966) gave a NOAEL for fertility in males of 17.5 mg B/kg/day.
Effect on fertility: via oral route
- Endpoint conclusion:
- adverse effect observed
- Dose descriptor:
- NOAEL
- 100 mg/kg bw/day
- Species:
- rat
- Quality of whole database:
- The most reliable comparable to guideline study with acceptable restrictions.
Effect on fertility: via dermal route
- Endpoint conclusion:
- no study available
Additional information
Effects on male fertility have been investigated in detail. A dose related effect on the testis was observed in rats, mice and deer mice, with confirmation from limited studies in dogs. Effects in rats start with reversible inhibition of spermiation after 14 days (at 39 mg B/kg bw/day) and 28 days (at 26 mg B/kg bw/day). At doses equal to and above 26 mg B/kg bw/day testicular atrophy, degeneration of seminiferous tubules and reduced sperm counts were observed. Male fertility was further investigated in two serial mating studies of treated male rats with untreated female rats. Infertility of treated males correlated well with germinal aplasia. Similar effects on male fertility were described in deer mice (Peromyscus maniculatus) after treatment with boric acid. Fertility studies in rats (two three-generation study with for boric acid and disodium tetraborate decahydrate) and mice (a continuous breeding study with boric acid) further support effects on testes as the underlying cause for reduced male fertility.
Diminished sperm production may be due to testicular effects on germ cell, Sertoli cell, or Leydig cell function or act via an alteration of the pituitary-hypothalamic axis. There is an indication that LH and FSH are elevated under boric acid treatment (Lee et al., 1978) and that serum testosterone may be decreased in CD-1 mice and F344 rats (Grizzle et al., 1989; reviewed in Fail et al., 1991; Treinen & Chapin, 1991). The decrease in prostate weight at 111.3 mg B/kg bw/day observed by Fail et al. (1991) might be caused by reduced testosterone levels.
A NOAEL of 17.5 mg B/kg bw/day for effects on female fertility was derived in the Transitional Annex XV dossier (TD 2008) based on Weir (1966c-d) and Fail et al,1991. However, the TD failed to adequately distinguish between effects on female fertility and effects on development. Fertility is generally defined in males as the ability to produce sperm which are capable of producing fertilisation of an ovum leading to conception. In females, it is defined as the ability to produce and release ova which can be fertilised leading to conception. To test fertility in animals males and females are pretreated to cover the period of development of the sperm and eggs, then mate and treat until the time of implantation, around Day 6 following mating, and then stop treatment in the females. To test for effects on development pregnant females are treated from Day 6 till the end of pregnancy. Neither the Weir and Fisher multigeneration study nor the Fail RACB studies were performed with this division of treatments. They both treated animals continuously before and during pregnancy and also after delivery.
In a three generation study in rats groups of 8 males and 16 females were treated with boric acid or disodium tetraborate decahydrate equivalent to 0, 5.9, 17.5 and 58.8 mg B/kg bw/day (Weir 1966c,d). An attempt was made to study the fertility of the P1 females at the top dose level by mating them with untreated males but only one litter of 16 pairs was produced. This highest dose level was clearly clinically toxic to the females after 2-3 weeks of dosing, with rough fur, scaly tails, inflamed eyelids and staining of the fur on the face and abdomen. The mating procedure to test the fertility of the females was not a satisfactory one. To avoid treatment of the males used for pairing, food was withdrawn from the cages of the females for 8 hours per day during the pairing process, and this is known to be very stressful to laboratory rats. There was no evidence on whether mating actually occurred for any of the rats, and no vaginal examinations for the presence of sperm were carried out. The females of the top dose P1 generation were sacrificed after 45 weeks of treatment and histopathological examination of the ovaries and uterus carried out. In the ovaries the presence of corpora lutea was regarded as a major indication of cyclic function, and these were found in 7 of 15 females, with reduced or absent function in the remaining 8 animals. The changes in the ovaries were not clearly different from those of controls. No treatment related changes were found in the uterus. No changes were found that could account for the reduced litter production, and no conclusions could be drawn about fertility in the top dose females. Comparable results were found in the Weir and Fisher multigeneration study on borax, with clear testicular atrophy at the top dose levels in males, and no clear explanation of the reduced number of litters in the top dose females, using the same unsatisfactory mating technique. The authors of the study concluded that testis atrophy was clearly produced in males at the top dose level, but that the evidence of the decreased ovulation in females did not account for the reduced number of litters in the cross mating study in females. Thus the Weir and Fisher studies produced clear evidence of adverse effects on male fertility, but did not produce clear evidence for an adverse effect on female fertility.
In a continuous breeding study of boric acid in Swiss mice (NTP, 1990; Fail et al., 1991), the three administered doses were 1000 ppm (26,6 mg B/kg bw/day), 4500 ppm (111,3 mg B/kg bw/day) and 9000 ppm (220,9 mg B/kg bw/day). A dose-related effect on the testis (testicular atrophy and effects on sperm motility, morphology and concentration) was noted; fertility was partially reduced at 111 mg B/kg bw/day, and absent at 221 mg B/kg bw/day.
For cross over mating only the mid dose group (111,3 mg B/kg bw/day) could be mated with control animals, since the high dose produced no litter. Indices of fertility for mid dose males with control females, control males with mid dose females and control males with control females were 5%, 65% and 74%, respectively. The according indices of mating (incidence of copulatory plugs) were 30%, 70% and 79%. This indicates that the primary effect was seen in males, however, slight effects were also noted in females. Live pup weight (adjusted for litter size) was significantly reduced compared to control litters, the average dam weight was significantly lower on postnatal day 0 compared to control dams and the average gestational period of the mid dose females was 1 day longer than in control females. The latter finding has also been observed in the developmental toxicity study by Price et al. (1996, see section 5.9.2).
In task 4 of this continuous breeding study control animals and low-dose F1 animals were mated because in the 9000 ppm groups no litters and in the 4500 ppm group only 3 litters were produced. While mating, fertility and reproductive competence were un-altered compared to control, the adjusted pup-weight (F2) was slightly but significantly decreased. F1 females had significantly increased kidney/adrenal and uterus weights and the oestrus cycle was significantly shorter compared to control females. A crossover mating study of controls and 4500 ppm groups confirmed the males as the affected sex.Necropsy at 27 weeks confirmed reduced testes weight, seminiferous tubule degeneration, decreased sperm count and motility and increase in abnormal sperm.In females at 27 weeks, 4500 ppm boric acid was toxic with decreased liver, kidney and adrenal weights, but no effect on oestrous cycles, mating, number of litters and number of pups. In F1 males a reduction in sperm concentration was observed, but no other sperm parameters were influenced.
While in this study the NOAEL for females of the F0-generation is 1000 ppm this is a LOAEL for males of the F0-generation (motility of epididymal sperms was significantly reduced: 78% ± 3 in controls vs. 69% ± 5 at 1000 ppm). For the F1-generation 1000ppm can be identified as a LOAEL, based on the 25% reduction of sperm concentration in males at this dose. Further, though normal in number, the F2-pups had reduced adjusted bodyweights at 1000 ppm, which is therefore also a LOAEL for F2-generation.
The authors concluded that the male is the most sensitive sex and that the testis is the primary target organ for boron. The NOAEL for testicular pathology in the present mouse study is probably 1000 ppm (26mg B/kg bodyweight). While males are more sensitive to boron induced toxicity, data also suggest an effect of boron on the female reproductive system. A reduced number of pups per litter and number of pups born alive at high dose levels are in agreement with earlier reports and could result from an effect of boron to alter implantation or to disrupt cell division in the embryo. This is supported by results of developmental toxicity studies in rats and mice in which higher dose levels can reduce the number of implants. Although F1 females had significantly increased kidney/adrenal and uterus weights and the oestrus cycle was significantly shorter compared to control female, similar effects were not observed in the 4500 ppm dose group, therefore the NOAEL for fertility in females was the dose level in diet of 4500 ppm, 846 mg/kg bw of boric acid or equivalent to 148 mg B/kg bodyweight.
In conclusion, the effects described in the Fail study on fertility show that 4500 ppm (111.3 mgB/kg bw) is a NOAEL for the females, and that other small effects in females are the result of developmental toxicity for which a NOAEL of <1000ppm (26.6mg B/kg bw) may be valid.
No further studies on the effects of boron on female fertility were reported by the National Toxicology Program team who published several other studies on the mechanism of action of boron on male fertility and on spermatogenesis. No effects on steroidogenic function were found in Leydig cells, and no clear mechanism of action to cause testis atrophy was identified by Ku and Chapin (1994).
Protective Effects of Zinc against Boric Acid Related Effects on Fertility
An important difference between laboratory animals and humans is the intrinsically higher zinc concentration in humans compared to laboratory animals. Normal levels of zinc in soft tissues in humans are over two times greater than in comparative tissues in laboratory animals (Figure 2, also see Appendix G, Figure 14) (King et al. 2000; Ranjan et al. 2011; Yamaguchi et al. 1996; Florianczyk 2000). The high zinc concentrations in humans compared to laboratory animals is also found in the target organs of boric acid, including fetal tissue, epididymis, and testes (Ahokas et al. 1980; Dorea et al. 1987; Suescun et al. 1981). The protective effect of the intrinsically large zinc stores in the human body against boric acid associated toxicity explains in part the absence of effects in humans exposed to high levels of boron.
Boron industry worker studies show that boron appears to concentrate in the semen, however without corresponding adverse effects on the semen. This is likely due to the fact that zinc concentrations are higher in semen in humans (112 µg zinc / ml), protective against the adverse effects of boric acid (Sorensen et al. 1999).
To investigate the effect of zinc on boric acid related toxicity on fertility effects an in vitro spermatogenesis study with boric acid in the presence of varying amounts of zinc was conducted (Durand 2013). Seminiferous tubules were cultured in the presence of 32 µg/ml boric acid and varying concentrations of zinc chloride equivalent to 0.48, 1.2, 2.4 and 4.8 µg Zn / ml to determine the effects on the number of somatic cells (sertoli and myoid cells) and germ cells (pre-meiotic cells (spermatogonia), meiotic cells (young spermatocytes, middle to late pachytene spermatocytes and secondary spermatocytes), and post-meiotic cells (round spermatids)) after 1 and 2 weeks of culture. An absence of boric acid related effects on spermatogenesis was observed in the presence of zinc (Figure 3, see also Appendix G, Figure 15). All germ cells populations were decreased by boric acid at 32 μg / ml. At D14, in the presence of boric acid increasing concentrations of zinc chloride lead to:- A dose dependent increase in the number of germ cells, an increased number of spermatogonia, a dose dependent increase in the number of young spermatocytes, a dose dependent increase of the number of middle to late pachytene spermatocytes, a dose dependent increase of secondary spermatocytes, and a dose dependent increase of round spermatids. Details of this study are presented in Appendix G. These results suggest that zinc interacts with boric acid reducing boric acid induced toxicity on spermatogenesis.
Zinc Borate Toxicity Studies
In addition to the in vitro study , a 28-day oral (gavage) dose range finding toxicity study of zinc borate and 90-day toxicity study of zinc borate in Sprague-Dawley Rats was also recently completed (See Kirkpatrick 2013 in Chemical Safety Report - Zinc Borate). This study is presented in more detail in Appendix G. Dosage levels tested in the 28-day study were 125, 250, 500, 1000 mg ZB/kg bw equivalent to 18.65, 37.3, 74.6 and 149.2 mg Boron/kg bw. A NOAEL of 37.3 mg B/kg bw and LOAEL of 74.6 mg B/kg bw for male fertility effects determined by minimal histopathologic findings in testes and epididymides was observed. Since hypospermia was not observed at the LOAEL, and the histopathological changes were graded as minimal, the effects observed in the 75 mg B/kg bw group were not considered toxicologically significant (Kirkpatrick 2013). The male fertility NOAEL for boric acid is 17.5 mg B/kg bw. No microscopic findings were noted in the ovaries.
Dosage levels tested in the 90-day study were 50, 100, 200, and 375 mg ZB/kg bw equivalent to 7.46, 14.92, 29.84 and 55.95 mg Boron/kg bw. The objectives of this study were to evaluate the potential toxicity of zinc borate when administered daily by oral gavage to Sprague Dawley rats for a minimum of 90 consecutive days and to assess recovery from such effects.
Adverse test substance-related microscopic findings were noted in the 375 mg/kg/day group males and consisted of germ cell degeneration in the testes, decreased size of the epididymides, inflammation of the prostate, and debris in the prostate. Test substance-related effects on spermatogenic parameters were noted in the 200 and 375 mg/kg/day group males at the study week 13 necropsies, as indicated by lower percentages of motility, progressive motility, and normal sperm at 200 and 375 mg/kg/day and a lower sperm production rate at 375 mg/kg/day. No microscopic findings were found in the testes or epididymis in the 200 mg/kg/day dose group. These effects for the 375 mg/kg/day group were considered adverse due to correlating microscopic findings.
Based on the results of this study, oral administration of zinc borate to Sprague Dawley rats for a minimum of 90 consecutive days resulted in no adverse effects for females at dosage levels of 50, 100, 200, and 375 mg/kg/day. For males, a dosage level of 375 mg/kg/day resulted in adverse effects on male reproductive organs, including effects on spermatogenic parameters with corresponding lower organ weights and gross and microscopic findings. Adverse effects on spermatogenic parameters were also noted at 200 mg/kg/day although there were no correlating microscopic findings. Therefore, no-observed-adverse-effect level (NOAEL) was 100 mg/kg/day for males and 375 mg/kg/day for females. Absence of microscopic findings in the testes and epididymides of the 200 mg/kg/day dose group suggest that zinc interacts with boric acid reducing boric acid induced toxicity on male reproductive organs.
Of note, even with high exposures to zinc, tissue concentrations in the testes, epididymis, and ovaries of rats remain well below normal zinc levels found in comparative human tissues. Although zinc tissue concentrations were not measured in the 28-day or 90-day zinc borate studies, zinc levels were measured in select tissues in a 90 day inhalation toxicity study of zinc oxide (ZnO) in rats sponsored by the U.S. Library of Congress and conducted by Battelle Laboratories(Placke 1990). The purpose of the study was to evaluate the potential toxicity of inhaled zinc oxide aerosol following sub-chronic exposures in rats (5 days per week for 13 consecutive weeks), to define the concentration response, to identify and characterize effects on target organs, to determine the tissue distribution of zinc as a function of concentration and continued exposure, to determine the reversibility of exposure-related toxic effects, to evaluate specific toxic potential on the immune, hematopoietic, and reproductive systems and to select concentrations for a possible subsequent chronic toxicity and carcinogenicity study in Fischer 344 Rats. Animals were exposed for 6 hours/day, 5 days/week for 13 weeks, to ZnO aerosol at 0, 1, 3, 10, 50 and 200 mg/m3. Five rats/sex/group were measured to determine absorption, distribution, accumulation, excretion and clearance of zinc. Exposure to 200 mg/m3 of ZnO resulted in a significantly increased total body burden of zinc. Tissue levels of zinc increased in most tissues (with the exception of testes, epididymis, ovaries, and RBC) during exposure and returned to near control levels during the recovery period (with the exception of lung and bone which showed zinc retention). Tissues with the greatest increases were lung, liver, pancreas and femer. Even after 90-days of exposure at the highest inhalation exposure concentration of ZnO, no increase in zinc levels were seen in the testes, epididymis, ovaries, and RBC and still well below the intrinsic levels observed in humans (See graph Appendix G). A greater degree of protection from boric acid related fertility effects would be expected in human tissues with substantially greater concentrations of zinc.
Although boron has been shown to adversely affect male reproduction in laboratory animals, male reproductive effects attributable to boron have not been demonstrated in studies of highly exposed workers.
Effects on developmental toxicity
Description of key information
A benchmark dose of 59 mg/kg bw/day (10.3 mg B/kg bw/day) for developmental toxicity developed by Allen et al. (1996) was based on the studies of Heindel et al. (1992), Price, Marr & Myers (1994) and Price et al. (1996).
Effect on developmental toxicity: via oral route
- Endpoint conclusion:
- adverse effect observed
- Dose descriptor:
- BMDL05
- 59 mg/kg bw/day
- Species:
- rat
- Quality of whole database:
- The study meets acceptable scientific standards with acceptable restrictions.
Effect on developmental toxicity: via inhalation route
- Endpoint conclusion:
- no study available
Effect on developmental toxicity: via dermal route
- Endpoint conclusion:
- no study available
Additional information
Developmental effects have been observed in three species, rats, mice and rabbits. The most sensitive species being the rat with a NOAEL of 9.6 mg B/kg bw/day. This is based on a reduction in mean foetal body weight/litter, increase in wavy ribs and an increased incidence in short rib XIII at 13.3 mg B/kg bw/day. The reduction in foetal body weight and skeletal malformations had reversed, with the exception of short rib XIII, by 21 days postnatal. At maternally toxic doses, visceral malformations observed included enlarged lateral ventricles and cardiovascular effects.
The NOAEL for this endpoint is 9.6 mg B/kg bw/day corresponding to 55 mg boric acid/kg bw/day; 85 mg disodium tetraborate decahydrate/kg, 65 mg disodium tetraborate pentahydrate/kg and 44.7 mg disodium tetraborate anhydrous/kg.
The critical effect is considered to be decreased fetal body weight in rats, for which the NOAEL was 9.6 mg/kg body weight per day. A benchmark dose developed by Allen et al. (1996) was based on the studies of Heindel et al. (1992), Price, Marr & Myers (1994) and Price et al. (1996). The benchmark dose is defined as the 95% lower bound on the dose corresponding to a 5% decrease in the mean fetal weight (BMDL05). The BMDL05of 10.3 mg/kg body weight per day as boron is close to the Price et al. (1996) NOAEL of 9.6 mg/kg body weight per day.
There is no evidence of developmental effects in humans attributable to boron in studies of populations with high exposures to boron (Tuccar et al 1998; Col et al. 2000; Chang et al. 2006).
Protective Effects of Zinc against Boric Acid Related Developmental Effects
Comparative zinc concentrations in humans and rat indicate that the protective effects of zinc are present early in the developing human fetus. Significantly higher concentrations (3x) of zinc in the human fetus compared to laboratory animals have been reported (Figure 2, see also Appendix G, Figure 14).
To investigate the effect of zinc on boric acid related toxicity on developmental effects, a GLP-compliant embryonic stem cell test (EST) conducted according to INVITTOX Protocol No. 113 was conducted to investigate the embryotoxic potential of boric acid in the presence of varying concentrations of zinc in vitro. No greater sensitivity of embryonic stem cells compared to fully differentiated cells was observed and no concern for in vivo embryotoxicity is triggered for boric acid at various concentrations of zinc. A reduction in the boric acid inhibition of differentiation of D3 embryonic stem cells was observed with increasing concentrations of zinc (Figure 4, see also Appendix G, Figure 16) (Hofman-Huther 2013).
The potential of zinc borate for developmental toxicity was evaluated based on the results of the Developmental Toxicity Test (Edwards 2014). Three treatment groups of 25 Sprague Dawley rats were administered the test article via oral gavage at dose levels of 100, 125 or 150 mg/kg/day. No test substance-related visceral developmental variations were noted in the 100, 125, and 150 mg/kg/day groups. There were no test substance-related skeletal malformations noted for foetuses in the test substance-treated groups. When the total malformations and developmental variations were evaluated on a proportional basis, no statistically significant differences from the control group were noted. Higher mean litter proportions of reduced ossification of the 13th rib(s) and sternebra(e) nos. 5 and/or 6 unossified were noted in the 100, 125, and 150 mg/kg/day groups compared to the control group. These findings were considered secondary to the reduced foetal weights noted in these groups. In addition, higher mean litter proportions of 7th cervical ribs and lower mean litter proportions of 14th rudimentary rib(s) were noted in the 100, 125, and 150 mg/kg/day groups and higher mean litter proportions of 25 presacral vertebrae were noted in the 125 and 150 mg/kg/day groups compared to the control group. No test substance-related foetal malformations were observed in the test substance-treated groups. Based on these results, a dosage level of 150 mg/kg/day was considered to be the no-observed-adverse-effect level (NOAEL) for maternal toxicity and a dosage level of <100 mg/kg/day was considered to be the NOAEL for embryo/foetal development when zinc borate was administered orally by gavage to inbred Crl: CD(SD) rats.
Justification for classification or non-classification
Boric acid is classified under the 1stATP to CLP as Repr. 1B; H360FD.
While boron has been shown to adversely affect male reproduction in laboratory animals, there is no clear evidence of male reproductive effects attributable to boron in studies of highly exposed workers (Whorton et al. 1994; Sayli 1998, 2001; Robbins et al. 2010; Scialli et al. 2010, Duydu 2011). There is also no evidence of developmental effects in humans attributable to boron in studies of populations with high exposures to boron (Tuccar et al 1998; Col et al. 2000; Chang et al. 2006).
Comparison of Blood, Semen and Testes Boron Levels in Human and Rat
A comparison of blood, semen and target organ boron levels in studies of laboratory animals and human studies shows that boron industry worker exposures are lower than untreated control rats. Background boron levels in standard rat chow are high (10-20 ppm), as a result control rats in toxicity studies receive 45 times more boron than background exposure in humans. Blood boron levels in female control rats is about 0.23 µg B/g (Price et al. 1997), approximately equal to the blood levels in boron industry workers in China, Turkey and U.S. of 0.25, 0.22 and 0.26 µg B/g, respectively (Scialli et al. 2010; Culver et al. 1994; Duydu et al. 2011). Plasma and seminal vesicle fluid (the major component of semen) boron levels in untreated male control rats were 1.94 and 2.05 µg B/g, respectively, while boron levels in testes in rats dosed at the rat fertility LOAEL (26 mg B/kg) was 5.6 µg B/g (Ku et al. 1991,1993). Values in male control rats were higher than corresponding boron levels in the highest exposed Chinese boron industry workers with blood boron levels of 1.56 µg B/g and 1.84 µg B/g in semen (Scialli et al. 2010). Blood and semen boron levels in highly exposed Turkish boron workers were also lower than control rats with levels of 0.22 and 1.88 µg B/g, respectively (Duydu et al. 2011). Boron levels in testes of rats dosed at the rat fertility LOAEL was over 3x the blood boron levels in highest exposure group of Chinese boron industry workers. The blood level at the lowest animal LOAEL (13 mg B/kg) was 1.53 µg B/g, about 6 times greater than typical boron industry workers (Price et al. 1997). No adverse effects on sperm were seen in Turkish boron industry workers or in the most highly exposed subgroup of Chinese boron industry workers drinking boron contaminated water (mean blood level 1.52 µg B/g, the human NOAEL). Only under extreme conditions do human levels reach those of this animal LOAEL: the subgroup of Chinese boron workers who also drank contaminated water. Since no boron accumulation occurs in soft tissues (testes) over plasma levels biological monitoring in humans provide direct comparison to test animal target organ boron levels. Workers in boron mining and processing industries represent the maximum possible human exposure however their blood and semen boron levels are less than levels in untreated control rats. This provides an explanation why studies of highly exposed boron industry workers have shown no adverse effects and demonstrates that maximal possible exposures in humans are insufficient to cause reproductive toxicity effects. Graphs comparing the rodent and human exposure, blood, semen and tissue boron levels are presented in Appendix C. The human exposure data do not support classification of boric acid as Category 1(B) reproductive toxicant.
Extensive evaluations of sperm parameters in highly exposed workers have demonstrated no effects on male fertility. While no developmental effects have been seen in highly exposed populations, epidemiological studies of developmental effects are not as robust as the fertility studies. Reproductive effects data for the developmental epidemiological studies were obtained by self-reported data collected by personal interviews of workers and questionnaires, small sample sizes, and lack of actual exposure measurements during pregnancy limit the conclusions that can be made from the developmental studies in humans.
Protective Effects of Zinc against Boric Acid Related Developmental Effects
Comparative zinc concentrations in humans and rat indicate that the protective effects of zinc are present early in the developing human fetus. Significantly higher concentrations (3x) of zinc in the human fetus compared to laboratory animals have been reported (Figure 2, see also Appendix G, Figure 14).
Embryonic Stem Cell Test with Zinc Chloride and Boric Acid
A GLP-compliant embryonic stem cell test (EST) conducted according to INVITTOX Protocol No. 113 to investigate the embryotoxic potential ofboric acid in the presence of varying concentrations of zincin vitro.The concentration of boric acid used in this study has been shown in previous EST tests to cause a 50% inhibition of differentiation of D3 cells into contracting myocardial cells (Genschow et al. 2004). The set concentration of boric acid tested was 150 µg/ml with concentrations of zinc chloride of 0, 1.04, 2.08, 10.42, 20.83, 41.67, 83.33 and 125 µg ZnCl/ml, equivalent to 0, 0.5, 1, 5, 10, 20, 40 and 60 µg Zn/ml. No greater sensitivity of embryonic stem cells compared to fully differentiated cells was observed and no concern for in vivo embryotoxicity is triggered for boric acid at various concentrations of zinc. A reduction in the boric acid inhibition of differentiation of D3 embryonic stem cells was observed with increasing concentrations of zinc (Figure 4, see also Appendix G) (Hofman-Huther 2013).
An Oral (Gavage) Prenatal Developmental Toxicity Study of Zinc Borate in Rats
The potential of zinc borate for developmental toxicity was evaluated based on the results of the Developmental Toxicity Test (Edwards 2014). Three treatment groups of 25 Sprague Dawley rats were administered the test article via oral gavage at dose levels of 100, 125 or 150 mg/kg/day. No test substance-related visceral developmental variations were noted in the 100, 125, and 150 mg/kg/day groups. There were no test substance-related skeletal malformations noted for foetuses in the test substance-treated groups. When the total malformations and developmental variations were evaluated on a proportional basis, no statistically significant differences from the control group were noted. Higher mean litter proportions of reduced ossification of the 13th rib(s) and sternebra(e) nos. 5 and/or 6 unossified were noted in the 100, 125, and 150 mg/kg/day groups compared to the control group. These findings were considered secondary to the reduced foetal weights noted in these groups. In addition, higher mean litter proportions of 7th cervical ribs and lower mean litter proportions of 14th rudimentary rib(s) were noted in the 100, 125, and 150 mg/kg/day groups and higher mean litter proportions of 25 presacral vertebrae were noted in the 125 and 150 mg/kg/day groups compared to the control group. No test substance-related foetal malformations were observed in the test substance-treated groups. Based on these results, a dosage level of 150 mg/kg/day was considered to be the no-observed-adverse-effect level (NOAEL) for maternal toxicity and a dosage level of <100 mg/kg/day was considered to be the NOAEL for embryo/foetal development when zinc borate was administered orally by gavage to inbred Crl: CD(SD) rats.
These data show that humans are likely less sensitive to the reproductive and developmental effects of boric acid than laboratory animals due to the comparative high zinc stores in target tissues in humans compared to laboratory animals.
Therefore, based on a total weight of evidence, Category 2 H361d: suspected human reproductive toxicant, suspected of damaging the unborn child is considered the appropriate classification. Extensive evaluations of sperm parameters in highly exposed workers have demonstrated no effects on male fertility. While no developmental effects have been seen in highly exposed populations, epidemiological studies of developmental effects are not as robust as the fertility studies, warranting the Category 2 H361d.
Specific Concentration Limit
Boric acid was formally classified under the 30th ATP to the Dangerous Substances Directive (67/548/EEC) as a Reproductive toxicant Category 2 with a Specific Concentration Limit of 5.5%. The classification was transferred to the CLP Regulation under the 1st ATP, where the classified category was aligned with the GHS (Category 1B) and the Specific Concentration Limit (SCL) was maintained. Although the ECHA CLP guidance includes setting concentration limits for reproductive toxicants, the current SCL for boric acid was agreed by the ECB Technical Committee on Classification & Labelling and accordingly became law when the 30th ATP entered into force.
Additional information
Information on Registered Substances comes from registration dossiers which have been assigned a registration number. The assignment of a registration number does however not guarantee that the information in the dossier is correct or that the dossier is compliant with Regulation (EC) No 1907/2006 (the REACH Regulation). This information has not been reviewed or verified by the Agency or any other authority. The content is subject to change without prior notice.
Reproduction or further distribution of this information may be subject to copyright protection. Use of the information without obtaining the permission from the owner(s) of the respective information might violate the rights of the owner.
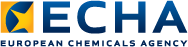