Registration Dossier
Registration Dossier
Data platform availability banner - registered substances factsheets
Please be aware that this old REACH registration data factsheet is no longer maintained; it remains frozen as of 19th May 2023.
The new ECHA CHEM database has been released by ECHA, and it now contains all REACH registration data. There are more details on the transition of ECHA's published data to ECHA CHEM here.
Diss Factsheets
Use of this information is subject to copyright laws and may require the permission of the owner of the information, as described in the ECHA Legal Notice.
EC number: 212-449-1 | CAS number: 818-08-6
- Life Cycle description
- Uses advised against
- Endpoint summary
- Appearance / physical state / colour
- Melting point / freezing point
- Boiling point
- Density
- Particle size distribution (Granulometry)
- Vapour pressure
- Partition coefficient
- Water solubility
- Solubility in organic solvents / fat solubility
- Surface tension
- Flash point
- Auto flammability
- Flammability
- Explosiveness
- Oxidising properties
- Oxidation reduction potential
- Stability in organic solvents and identity of relevant degradation products
- Storage stability and reactivity towards container material
- Stability: thermal, sunlight, metals
- pH
- Dissociation constant
- Viscosity
- Additional physico-chemical information
- Additional physico-chemical properties of nanomaterials
- Nanomaterial agglomeration / aggregation
- Nanomaterial crystalline phase
- Nanomaterial crystallite and grain size
- Nanomaterial aspect ratio / shape
- Nanomaterial specific surface area
- Nanomaterial Zeta potential
- Nanomaterial surface chemistry
- Nanomaterial dustiness
- Nanomaterial porosity
- Nanomaterial pour density
- Nanomaterial photocatalytic activity
- Nanomaterial radical formation potential
- Nanomaterial catalytic activity
- Endpoint summary
- Stability
- Biodegradation
- Bioaccumulation
- Transport and distribution
- Environmental data
- Additional information on environmental fate and behaviour
- Ecotoxicological Summary
- Aquatic toxicity
- Endpoint summary
- Short-term toxicity to fish
- Long-term toxicity to fish
- Short-term toxicity to aquatic invertebrates
- Long-term toxicity to aquatic invertebrates
- Toxicity to aquatic algae and cyanobacteria
- Toxicity to aquatic plants other than algae
- Toxicity to microorganisms
- Endocrine disrupter testing in aquatic vertebrates – in vivo
- Toxicity to other aquatic organisms
- Sediment toxicity
- Terrestrial toxicity
- Biological effects monitoring
- Biotransformation and kinetics
- Additional ecotoxological information
- Toxicological Summary
- Toxicokinetics, metabolism and distribution
- Acute Toxicity
- Irritation / corrosion
- Sensitisation
- Repeated dose toxicity
- Genetic toxicity
- Carcinogenicity
- Toxicity to reproduction
- Specific investigations
- Exposure related observations in humans
- Toxic effects on livestock and pets
- Additional toxicological data

Neurotoxicity
Administrative data
Description of key information
The following study has been submitted to address the neurotoxicity endpoint:
EFSA (2004) Opinion of the Scientific Panel on Contaminants in the Food Chain on a request from the Commission to assess the health risks to consumers associated with exposure to organotins in foodstuffs. The EFSA Journal 1002: 1-119
As the study is secondary source it has been allocated a Klimisch score of 4.
Key value for chemical safety assessment
Effect on neurotoxicity: via oral route
Link to relevant study records
- Endpoint:
- neurotoxicity: oral
- Type of information:
- experimental study
- Adequacy of study:
- supporting study
- Reliability:
- 4 (not assignable)
- Rationale for reliability incl. deficiencies:
- other: see 'Remark'
- Remarks:
- Scientific opinion of EFSA citing numerous studies on toxicological effects of oganotin compounds. The Committee preparing the document primarily focused on compounds for which both the toxicological and exposure databases were considered suitable. Data used in the assessment is cited only as short abstracts, and it is therefore not possible to evaluate the reliability of the individual studies reviewed in the document.
- Reason / purpose for cross-reference:
- other: read-across target
- Principles of method if other than guideline:
- Data reviewed and cited by EFSA in a publically available report addressing the potential effects of organotin concentration in food. Reference is made to a study where male Wistar rats were dosed intraperitoneally with DBTCl2 at 4 mg/kg b.w. Original reference cited: Ishizaka, T., Suzuki, T. and Saito, Y. 1989. Metabolism of Dibutyl Dichloride in Male Rats. J. Agric. Food Chem. 37:1096-1101. Other studies are referenced
- GLP compliance:
- not specified
- Conclusions:
- The report concludes:
"While there is clear-cut evidence for neurotoxicity with regard to TMT, TET and recently also TPT other tri- and also dialkyltin are less or – possibly - not neurotoxic at all. Obviously a lack of systematic experimental data exists in the open literature comparing their in vivo neurotoxicity. However there are some indications for neurobehavioral effects. With regard to risk assessment NOAELs cannot be derived from the studies presently available. The lowest effect levels reported for rats were 1 mg TBTCl/kg b.w./day in a neurodevelopmental study and 0.36 mg TPTOAc/kg b.w. and day in the maze learning test." - Endpoint:
- neurotoxicity: oral
- Type of information:
- read-across from supporting substance (structural analogue or surrogate)
- Adequacy of study:
- supporting study
- Reliability:
- 2 (reliable with restrictions)
- Rationale for reliability incl. deficiencies:
- other: Study conducted on read-across material. Source study assigned reliability score of 4.
- Justification for type of information:
- Study conducted on structural analogue dibutyltin chloride
- Reason / purpose for cross-reference:
- read-across source
Referenceopen allclose all
In the report by EFSA, the neurotoxic effects of TBT and DBT are discussed together due to the metabolism of TBT by the liver in vivo tests to form DBT.
Toxicokinetic data included in the published scientific opinion by EFSA stated thatin vivo, the process of biotransformation of TBT particularly in the liver is characterized by hydroxylation and dealkylation via cytochrome P-450 deriving DBT, MBT and inorganic tin. Metabolites are detectable in liver within 3 hours of TBTAc administration (1.2 mg/kg b.w.) to Swiss Webster mice.Intraperitoneal administration of DBTCl2 at 4 mg/kg b.w. to male Wistar rats the parent compound and small amounts of its metabolites 3-OHDBT and MBT were detected in the brain over a period of 7 days (Ishizakaet al., 1989).
After a single oral dose of TBTCl (15 mg/kg b.w.), high concentrations were found initially in the cerebellum but also in the frontal and temporal lobe of rabbits (cited in WHO-IPCS, 1990). Also after a single oral dose of 40 mg TBTCl/kg b.w. to male Wistar rats, TBT initially increased in the brain. It was dealkylated to DBT, MBT and inorganic tin. Mainly MBT and inorganic tin persisted in the brain during the observation period (8 days) (Iwaiet al., 1981).
According to the review, there is currently no reported evidence of neurotoxicity in humans of TBT. Toxic effects are noted after acute exposures, however the reported symptomatology are non-specific. Some kinetic experiments have demonstrated that the transfer of TBT/DBT into the central nervous system is not prevented by the blood brain barrier; existing animal studies do not convincingly show that TBT is neurotoxic in non-lethal doses; at present there are no satisfactory mechanistic explanations. Obviously the neurotoxic potency of OTC differs substantially and for some OTC it is partially unexplored as systematic experimental studies in laboratory animals (dose-response) on their comparative in vivo neurotoxicity are not available.
In Aldridge (1976) the order of effectiveness in causing 50 % inhibition of ATP production in liver mitochondria was found to be TET > TPrT > TBT > THT > TMT. TET was the most effective and the other established human neurotoxin TMT was the “weakest”, suggests that all OTC have the potential to be potent neurotoxins. Reportedly, the dialkyltin compounds, DMT, DET, DBT and DOT have similar biochemical effectsin vitro(Snoeijet al., 1987; Boyer, 1989).
Slice cultures of immature hippocampus from PND 10 Wistar rats testsed with TBT induced neuronal death in a concentration- (0.1 - 10µM) (time-dependent). The morphology of the cell death had apoptosis-like features and free radical scavengers or antagonists to the AMPA glutamate receptor subtype were shown to be protective (Mizuhashiet al., 2000).
At concentrations of TMT ten-fold lower than those required to induce neurotoxicity, disruption of the cell integrity were observed (Noraberget al., 1998). In the mouse (male Swiss-Webster), TBT was reported as a potent inhibitor of GABA uptake into the forebrain synaptosomes with a reported IC50 value ten-fold lower than that observed with TMT. This was partly attributed to the inhibition of Na+/K+ATPases (Costa, 1985). In immortalized cell lines, primary neuronal cells (Thompsonet al., 1996) and in pheochromocytoma PC 12 cells (Vivianiet al., 1995) TBT has been shown to induce apoptosis. This is consistent with other reports obtained in testing of non-neuronal cells (Aldridge, 1976; Snoeijet al., 1986a,b) and also consistent with the reports of disruption in chemical energy synthesis in neurons. In male ICR mice, Kobayashiet al.,(1996), demonstrated in the cortex cortex, butyl tins (1 - 100µM) inhibited various parameters of cholinergic activity, TBT being the more potent compound ahead of DBT and MBT. DBT appeared to be more toxic than TMT in brain cell cultures of 16 day old foetal rat (Eskeset al., 1999).
A number of experimental attempts have been made to demonstrate neurotoxic effects of TBT. Typically TBT was applied in dosages between 0.5 and 10 mg/kg b.w.. However, no study was available which convincingly showed TBT as toxic to the nervous system, no valid conclusions could be drawn (Benya, 1997; Poitouet al., 1978). Most noticeably the effects Barnes and Stoner (1958, 1959) observed seem to resemble the effects of TET as they reported the induction of interstitial oedema of the white matter of the central nervous system in a subchronic feeding study (three months) of TBTOAc (100 mg/kg feed) to male rats or after administration of a lethal dose to female rats. This, in principle is consistent with the results seen in TET toxicity, this also concurs with thein vitroevidence of the interference of TBT with chemical energy production by the mitochondrial chain. In ocular studies, toxicity in which the induction of corneal oedema was reported (Yoshizukaet al., 1991). However, this has never been reproduced. Krajncet al.,(1984) observed ataxia (usually indicative of disease of white matter) after feeding a dietary concentration of 320 mg/kg TBTO to Wistar rats (equivalent to 30 mg/kg b.w./day) over four weeks. No evidence of brain oedema was seen. TBTO was given orally to male Sprague-Dawley rats in dosages of either 37.5 or 75 mg/kg b.w. for three consecutive days. Mortality rates were 12 and 30 %, respectively; in the remaining animals aggression and seizures were seen. Some histopathological features remained non-specific, however a complete absence of Purkinje cells in the cerebellum was detected 7 days post-treatment. The activity of total brain ATPase, Na+/K+ATPase, and Mg2+ATPase was found to be suppressed (Elsabbaghet al., 2002).I.p.injection of 10 mg TBTO/kg b.w. or 80 mg DBTO/kg b.w. induced a reduction of cerebral 5-hydroxytryptamine and noradrenaline concentrations in the rat (cited in Benya, 1997). The implications of this observation should be considered, changes in monoamine levels is not trivial as neither behavioural alterations nor morphological changes were described. In a similar study exposure of female Wistar rats to DBT dilaureate (20, 40 or 80 mg/kg b.w.) for three days, at all treatment levels a decrease in levels of noradrenaline, dopamine and serotonin was observed. The hypothalamus and frontal cortex were the most affected. Maximum decrease of dopamine was found in corpus striatum, nordrenaline in pons medulla and of serotonin in frontal cortex. A decrease in spontaneous locomotor activity and learning at all doses was observed (a NOAEL was not established) (Alamet al., 1988). The behavioural effects of a single oral exposure to nonlethal doses of TBTCl (6.3, 12.5, 25.0 or 50.0 mg/kg b.w.) were studied in male Wistar rats. Spontaneous motor activity (SMA) and acquisition of conditioned avoidance responses were monitored. TBT was found to cause a dose-related decrease in SMA during the dark phase. The 24-hour total daily and 12 hours nocturnal activity was decreased at 12.5 mg/kg and above. The acquisition of shock avoidance responses was inhibited in all TBTCl-treated groups in a dose-dependent manner. The difference was significant at 25 mg/kg b.w.. The conclusion from the authors was that TBT can cause significant changes in rat behaviour (Emaet al., 1991). Dietary exposure of male mice (BALB/c) to TBT (1 - 125 mg/kg feed) for 30 days produced inhibition of ligand binding to the NMDA receptor (Konnoet al., 2001). In Gardlundet al.,(1991) 1 or 5 mg/kg b.w. TBTCl was administered orally during the 6th to the 20th day of pregnancy to Sprague-Dawley rats. As adults, obvious alterations in behaviour were noted; effects such as a general hyperactivity, dysfunction of spatial learning performance and a drastic potentiation of the stimulating effects of amphetamine were noted in the TBT treated offspring. This occurred at doses that did not induce overt maternal toxicity or decreased offspring viability. No neurochemical correlate for these observations were found, neurohistological changes were not examined (a NOAEL was not also not established). In O’Callaghan and Miller (1988) a single dose of TBTO (2, 3 or 4 mg/kg i.p.) in the neonatal rat (Long-Evans, PND 5) induced dose- and region-dependent decreases in brain weight most noticeably so in the cerebellum. Reductions of p38 (synaptic vesicle associated protein) and myelin basic protein (oligodendroglia and myelin-sheath associated protein) were seen in the cerebellum and forebrain, but not in the hippocampus. These effects, determined on postnatal day 13, 22 and 60, were seen at dosages that did not affect brain, thymus, or body weight; if dosages did not influence body weight, reductions in brain weight, p38 and myelin basic protein were reversible.
In the report by EFSA, the neurotoxic effects of TBT and DBT are discussed together due to the metabolism of TBT by the liverin vivotests to form DBT.
Toxicokinetic data included in the published scientific opinion by EFSA stated thatin vivo, the process of biotransformation of TBT particularly in the liver is characterized by hydroxylation and dealkylation via cytochrome P-450 deriving DBT, MBT and inorganic tin. Metabolites are detectable in liver within 3 hours of TBTAc administration (1.2 mg/kg b.w.) to Swiss Webster mice.Intraperitoneal administration of DBTCl2at 4 mg/kg b.w. to male Wistar rats the parent compound and small amounts of its metabolites 3-OHDBT and MBT were detected in the brain over a period of 7 days (Ishizakaet al., 1989).
After a single oral dose of TBTCl (15 mg/kg b.w.), high concentrations were found initially in the cerebellum but also in the frontal and temporal lobe of rabbits (cited in WHO-IPCS, 1990). Also after a single oral dose of 40 mg TBTCl/kg b.w. to male Wistar rats, TBT initially increased in the brain. It was dealkylated to DBT, MBT and inorganic tin. Mainly MBT and inorganic tin persisted in the brain during the observation period (8 days) (Iwaiet al., 1981).
According to the review, there is currently no reported evidence of neurotoxicity in humans of TBT. Toxic effects are noted after acute exposures, however the reported symptomatology are non-specific. Some kinetic experiments have demonstrated that the transfer of TBT/DBT into the central nervous system is not prevented by the blood brain barrier; existing animal studies do not convincingly show that TBT is neurotoxic in non-lethal doses; at present there are no satisfactory mechanistic explanations. Obviously the neurotoxic potency of OTC differs substantially and for some OTC it is partially unexplored as systematic experimental studies in laboratory animals (dose-response) on their comparative in vivo neurotoxicity are not available.
In Aldridge (1976) the order of effectiveness in causing 50 % inhibition of ATP production in liver mitochondria was found to be TET > TPrT > TBT > THT > TMT. TET was the most effective and the other established human neurotoxin TMT was the “weakest”, suggests that all OTC have the potential to be potent neurotoxins. Reportedly, the dialkyltin compounds, DMT, DET, DBT and DOT have similar biochemical effectsin vitro(Snoeijet al., 1987; Boyer, 1989).
Slice cultures of immature hippocampus from PND 10 Wistar rats testsed with TBT induced neuronal death in a concentration- (0.1 - 10µM) (time-dependent). The morphology of the cell death had apoptosis-like features and free radical scavengers or antagonists to the AMPA glutamate receptor subtype were shown to be protective (Mizuhashiet al., 2000).
At concentrations of TMT ten-fold lower than those required to induce neurotoxicity, disruption of the cell integrity were observed (Noraberget al., 1998). In the mouse (male Swiss-Webster), TBT was reported as a potent inhibitor of GABA uptake into the forebrain synaptosomes with a reported IC50 value ten-fold lower than that observed with TMT. This was partly attributed to the inhibition of Na+/K+ATPases (Costa, 1985). In immortalized cell lines, primary neuronal cells (Thompsonet al., 1996) and in pheochromocytoma PC 12 cells (Vivianiet al., 1995) TBT has been shown to induce apoptosis. This is consistent with other reports obtained in testing of non-neuronal cells (Aldridge, 1976; Snoeijet al., 1986a,b) and also consistent with the reports of disruption in chemical energy synthesis in neurons. In male ICR mice, Kobayashiet al.,(1996), demonstrated in the cortex cortex, butyl tins (1 - 100µM) inhibited various parameters of cholinergic activity, TBT being the more potent compound ahead of DBT and MBT. DBT appeared to be more toxic than TMT in brain cell cultures of 16 day old foetal rat (Eskeset al., 1999).
A number of experimental attempts have been made to demonstrate neurotoxic effects of TBT. Typically TBT was applied in dosages between 0.5 and 10 mg/kg b.w.. However, no study was available which convincingly showed TBT as toxic to the nervous system, no valid conclusions could be drawn (Benya, 1997; Poitouet al., 1978). Most noticeably the effects Barnes and Stoner (1958, 1959) observed seem to resemble the effects of TET as they reported the induction of interstitial oedema of the white matter of the central nervous system in a subchronic feeding study (three months) of TBTOAc (100 mg/kg feed) to male rats or after administration of a lethal dose to female rats. This, in principle is consistent with the results seen in TET toxicity, this also concurs with thein vitroevidence of the interference of TBT with chemical energy production by the mitochondrial chain. In ocular studies, toxicity in which the induction of corneal oedema was reported (Yoshizukaet al., 1991). However, this has never been reproduced. Krajncet al.,(1984) observed ataxia (usually indicative of disease of white matter) after feeding a dietary concentration of 320 mg/kg TBTO to Wistar rats (equivalent to 30 mg/kg b.w./day) over four weeks. No evidence of brain oedema was seen. TBTO was given orally to male Sprague-Dawley rats in dosages of either 37.5 or 75 mg/kg b.w. for three consecutive days. Mortality rates were 12 and 30 %, respectively; in the remaining animals aggression and seizures were seen. Some histopathological features remained non-specific, however a complete absence of Purkinje cells in the cerebellum was detected 7 days post-treatment. The activity of total brain ATPase, Na+/K+ATPase, and Mg2+ATPase was found to be suppressed (Elsabbaghet al., 2002).I.p.injection of 10 mg TBTO/kg b.w. or 80 mg DBTO/kg b.w. induced a reduction of cerebral 5-hydroxytryptamine and noradrenaline concentrations in the rat (cited in Benya, 1997). The implications of this observation should be considered, changes in monoamine levels is not trivial as neither behavioural alterations nor morphological changes were described. In a similar study exposure of female Wistar rats to DBT dilaureate (20, 40 or 80 mg/kg b.w.) for three days, at all treatment levels a decrease in levels of noradrenaline, dopamine and serotonin was observed. The hypothalamus and frontal cortex were the most affected. Maximum decrease of dopamine was found in corpus striatum, nordrenaline in pons medulla and of serotonin in frontal cortex. A decrease in spontaneous locomotor activity and learning at all doses was observed (a NOAEL was not established) (Alamet al., 1988). The behavioural effects of a single oral exposure to nonlethal doses of TBTCl (6.3, 12.5, 25.0 or 50.0 mg/kg b.w.) were studied in male Wistar rats. Spontaneous motor activity (SMA) and acquisition of conditioned avoidance responses were monitored. TBT was found to cause a dose-related decrease in SMA during the dark phase. The 24-hour total daily and 12 hours nocturnal activity was decreased at 12.5 mg/kg and above. The acquisition of shock avoidance responses was inhibited in all TBTCl-treated groups in a dose-dependent manner. The difference was significant at 25 mg/kg b.w.. The conclusion from the authors was that TBT can cause significant changes in rat behaviour (Emaet al., 1991). Dietary exposure of male mice (BALB/c) to TBT (1 - 125 mg/kg feed) for 30 days produced inhibition of ligand binding to the NMDA receptor (Konnoet al., 2001). In Gardlundet al.,(1991) 1 or 5 mg/kg b.w. TBTCl was administered orally during the 6th to the 20th day of pregnancy to Sprague-Dawley rats. As adults, obvious alterations in behaviour were noted; effects such as a general hyperactivity, dysfunction of spatial learning performance and a drastic potentiation of the stimulating effects of amphetamine were noted in the TBT treated offspring. This occurred at doses that did not induce overt maternal toxicity or decreased offspring viability. No neurochemical correlate for these observations were found, neurohistological changes were not examined (a NOAEL was not also not established). In O’Callaghan and Miller (1988) a single dose of TBTO (2, 3 or 4 mg/kg i.p.) in the neonatal rat (Long-Evans, PND 5) induced dose- and region-dependent decreases in brain weight most noticeably so in the cerebellum. Reductions of p38 (synaptic vesicle associated protein) and myelin basic protein (oligodendroglia and myelin-sheath associated protein) were seen in the cerebellum and forebrain, but not in the hippocampus. These effects, determined on postnatal day 13, 22 and 60, were seen at dosages that did not affect brain, thymus, or body weight; if dosages did not influence body weight, reductions in brain weight, p38 and myelin basic protein were reversible.
Additional information
The SPCFC (2004) review of organotins considered evidence of neurotoxicity and concluded that, while there is clear-cut evidence of neurotoxicity with regard to trimethyl tin, triethyltin and triphenyltin, other tri- and also di-alkyltins are less or - possibly - not neurotoxic at all. There is a lack of systematic experimental data showing NOAELs. Dibutyltin shows the same biochemical effect (decoupling of oxidative phosphorylation). With regard to risk assessment NOAELs cannot be derived from the studies. It is however clear from available studies, that any potential neurotoxicity is not the most sensitive marker of dibutyltin toxicity.
Justification for classification or non-classification
Information on Registered Substances comes from registration dossiers which have been assigned a registration number. The assignment of a registration number does however not guarantee that the information in the dossier is correct or that the dossier is compliant with Regulation (EC) No 1907/2006 (the REACH Regulation). This information has not been reviewed or verified by the Agency or any other authority. The content is subject to change without prior notice.
Reproduction or further distribution of this information may be subject to copyright protection. Use of the information without obtaining the permission from the owner(s) of the respective information might violate the rights of the owner.
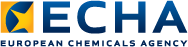