Registration Dossier
Registration Dossier
Data platform availability banner - registered substances factsheets
Please be aware that this old REACH registration data factsheet is no longer maintained; it remains frozen as of 19th May 2023.
The new ECHA CHEM database has been released by ECHA, and it now contains all REACH registration data. There are more details on the transition of ECHA's published data to ECHA CHEM here.
Diss Factsheets
Use of this information is subject to copyright laws and may require the permission of the owner of the information, as described in the ECHA Legal Notice.
EC number: 203-743-0 | CAS number: 110-17-8
- Life Cycle description
- Uses advised against
- Endpoint summary
- Appearance / physical state / colour
- Melting point / freezing point
- Boiling point
- Density
- Particle size distribution (Granulometry)
- Vapour pressure
- Partition coefficient
- Water solubility
- Solubility in organic solvents / fat solubility
- Surface tension
- Flash point
- Auto flammability
- Flammability
- Explosiveness
- Oxidising properties
- Oxidation reduction potential
- Stability in organic solvents and identity of relevant degradation products
- Storage stability and reactivity towards container material
- Stability: thermal, sunlight, metals
- pH
- Dissociation constant
- Viscosity
- Additional physico-chemical information
- Additional physico-chemical properties of nanomaterials
- Nanomaterial agglomeration / aggregation
- Nanomaterial crystalline phase
- Nanomaterial crystallite and grain size
- Nanomaterial aspect ratio / shape
- Nanomaterial specific surface area
- Nanomaterial Zeta potential
- Nanomaterial surface chemistry
- Nanomaterial dustiness
- Nanomaterial porosity
- Nanomaterial pour density
- Nanomaterial photocatalytic activity
- Nanomaterial radical formation potential
- Nanomaterial catalytic activity
- Endpoint summary
- Stability
- Biodegradation
- Bioaccumulation
- Transport and distribution
- Environmental data
- Additional information on environmental fate and behaviour
- Ecotoxicological Summary
- Aquatic toxicity
- Endpoint summary
- Short-term toxicity to fish
- Long-term toxicity to fish
- Short-term toxicity to aquatic invertebrates
- Long-term toxicity to aquatic invertebrates
- Toxicity to aquatic algae and cyanobacteria
- Toxicity to aquatic plants other than algae
- Toxicity to microorganisms
- Endocrine disrupter testing in aquatic vertebrates – in vivo
- Toxicity to other aquatic organisms
- Sediment toxicity
- Terrestrial toxicity
- Biological effects monitoring
- Biotransformation and kinetics
- Additional ecotoxological information
- Toxicological Summary
- Toxicokinetics, metabolism and distribution
- Acute Toxicity
- Irritation / corrosion
- Sensitisation
- Repeated dose toxicity
- Genetic toxicity
- Carcinogenicity
- Toxicity to reproduction
- Specific investigations
- Exposure related observations in humans
- Toxic effects on livestock and pets
- Additional toxicological data

Endpoint summary
Administrative data
Link to relevant study record(s)
Description of key information
Short description of key information on bioaccumulation potential result:
Information regarding the distribution of fumaric acid is available from an older published study (Marshall et al, 1948). These data, combined with the role of fumaric acid in mammalian metabolism and its long history of safe therapeutic use (SCAN, 2003; JECFA, 1999), provide sufficient toxicological information such that additional testing in animals is not necessary.
Key value for chemical safety assessment
- Bioaccumulation potential:
- no bioaccumulation potential
Additional information
Information regarding the distribution of fumaric acid in the rat and its role in mammalian metabolism is available in the published literature. Average concentrations in the blood, brain tissue, kidney tissue, liver and muscle were 3 mg/L, 150 mg/kg, 95 mg/kg, 78 mg/kg, and 23 mg/kg, respectively. Fumaric acid plays an essential role in aerobic respiration and has a long history of safe use as a human therapeutic agent. The European Commission's Scientific Committee for Animal Nutrition stated that a dose of 0.9g fumaric acid equivalent per day was considered safe for humans. The Joint FAO/WHO Committee on Food Additives concluded that there is no safety concern at current levels of intake when fumaric acid is used as a flavouring agent. Based on the available data, fumaric acid is of low concern for toxicity and additional toxicokinetic testing is not required.
Fumaric acid is an organic dicarboxylic acid (C4H4O4). Fumaric acid occurs naturally in the metabolism. It plays a role in the tricarboxylic cycle (TCA cycle) and in the carry over of the amino-N from aspartate. Succinate (acetyl-CoA and oxaloacetate are synthetised to citrate, which is -by multiple steps - converted to succinate) is oxidised to fumarate. Succinate also enters as succinyl-CoA the TCA-cycle as a conversion product of the glucogenic amino acids methionine, isoleucine and valine. The glucogenic amino acids phenylalanine and tyrosine enter the TCA cycle at the stage of fumarate. Fumarate is further converted to malate (by saturation of the double bond). Hydration of fumaric acid is one means of producing malic acid (consequently some information is used as read-across for the similar fumaric acid). Oxidation of malate produces oxaloacetate. By transamination oxaloacetate is in a reversible equilibirum with aspartate. Malate is also important outside the TCA cycle ((i) as a shuttle of reducing equivalents (NADH) from cytoplasm into mitochondria, (ii) it can be converted in the cytoplasm to pyruvate (NADPH source by malic enzyme) and (iii) as a precursor of cytoplasmic synthesis of fatty acids). Aspartate is used for protein synthesis and as a precursor of the pyrimidine bases (the carbon skeleton is used for Uracil, Thymine and Cytosine) and the purine bases (Adenosine and Guanine contain the N1 from amino-N-aspartate and another amino group from aspartate on the C6 of Adenosine). Fumarate results from the the carry over of the aspartate-amino-N via succinate. By the same kind of reaction fumarate also results from the urea cycle in the (periportal) hepatocytes. Citrulline and aspartate form arginino-succinate, which is in turn converted to arginine and fumarate. This reaction is reversible. Aspartate can again be resynthesised from fumarate via malate and oxaloacetate. The urea cycle leads also to binding and excretion of bicarbonate and takes therefore part in the regulation of the acid-base-equilibrium. Urea formation is reduced if pH and/or the HCO3 - concentration in the extracellular space decrease. The resulting increase in ammonia concentration is compensated by ammonia fixation as glutamin (in perivenous hepatocytes). Fumarate results also from the degradation of the amino acid tyrosine (hydroxyphenylalanine). Tyrosine serves as a precursor of several syntheses (melanine (a pigment in skin and hair), dopamine and the catecholamines (hormones of the perineal glands), thyroid hormones and probably ubiquinone).
Discussion on bioaccumulation potential result:
Fumaric acid is an organic dicarboxylic acid (C4H4O4). Fumaric acid occurs naturally in the metabolism. It plays a role in the tricarboxylic cycle (TCA cycle) and in the carry over of the amino-N from aspartate.
Succinate (acetyl-CoA and oxaloacetate are synthetised to citrate, which is -by multiple steps - converted to succinate) is oxidised to fumarate. Succinate also enters as succinyl-CoA the TCA-cycle as a conversion product of the glucogenic amino acids methionine, isoleucine and valine. The glucogenic amino acids phenylalanine and tyrosine enter the TCA cycle at the stage of fumarate. Fumarate is further converted to malate (by saturation of the double bond). Hydration of fumaric acid is one means of producing malic acid (consequently some information is used as read-across for the similar fumaric acid). Oxidation of malate produces oxaloacetate. By transamination oxaloacetate is in a reversible equilibirum with aspartate. Malate is also important outside the TCA cycle ((i) as a shuttle of reducing equivalents (NADH) from cytoplasm into mitochondria, (ii) it can be converted in the cytoplasm to pyruvate (NADPH source by malic enzyme) and (iii) as a precursor of cytoplasmic synthesis of fatty acids). Aspartate is used for protein synthesis and as a precursor of the pyrimidine bases (the carbon skeleton is used for Uracil, Thymine and Cytosine) and the purine bases (Adenosine and Guanine contain the N1 from amino-N-aspartate and another amino group from aspartate on the C6 of Adenosine). Fumarate results from the the carry over of the aspartate-amino-N via succinate. By the same kind of reaction fumarate also results from the urea cycle in the (periportal) hepatocytes. Citrulline and aspartate form arginino-succinate, which is in turn converted to arginine and fumarate. This reaction is reversible. Aspartate can again be resynthesised from fumarate via malate and oxaloacetate. The urea cycle leads also to binding and excretion of bicarbonate and takes therefore part in the regulation of the acid-base-equilibrium. Urea formation is reduced if pH and/or the HCO3 - concentration in the extracellular space decrease. The resulting increase in ammonia concentration is compensated by ammonia fixation as glutamin (in perivenous hepatocytes). Fumarate results also from the degradation of the amino acid tyrosine (hydroxyphenylalanine). Tyrosine serves as a precursor of several syntheses (melanine (a pigment in skin and hair), dopamine and the catecholamines (hormones of the perineal glands), thyroid hormones and probably ubiquinone).
Information regarding the distribution of fumaric acid is available from an older published study (Marshall et al, 1948). These data, combined with the role of fumaric acid in mammalian metabolism and its long history of safe therapeutic use (SCAN, 2003; JECFA, 1999), provide sufficient toxicological information such that additional testing in animals is not necessary.
Information on Registered Substances comes from registration dossiers which have been assigned a registration number. The assignment of a registration number does however not guarantee that the information in the dossier is correct or that the dossier is compliant with Regulation (EC) No 1907/2006 (the REACH Regulation). This information has not been reviewed or verified by the Agency or any other authority. The content is subject to change without prior notice.
Reproduction or further distribution of this information may be subject to copyright protection. Use of the information without obtaining the permission from the owner(s) of the respective information might violate the rights of the owner.
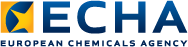