Registration Dossier
Registration Dossier
Data platform availability banner - registered substances factsheets
Please be aware that this old REACH registration data factsheet is no longer maintained; it remains frozen as of 19th May 2023.
The new ECHA CHEM database has been released by ECHA, and it now contains all REACH registration data. There are more details on the transition of ECHA's published data to ECHA CHEM here.
Diss Factsheets
Use of this information is subject to copyright laws and may require the permission of the owner of the information, as described in the ECHA Legal Notice.
EC number: 231-131-3 | CAS number: 7440-22-4
- Life Cycle description
- Uses advised against
- Endpoint summary
- Appearance / physical state / colour
- Melting point / freezing point
- Boiling point
- Density
- Particle size distribution (Granulometry)
- Vapour pressure
- Partition coefficient
- Water solubility
- Solubility in organic solvents / fat solubility
- Surface tension
- Flash point
- Auto flammability
- Flammability
- Explosiveness
- Oxidising properties
- Oxidation reduction potential
- Stability in organic solvents and identity of relevant degradation products
- Storage stability and reactivity towards container material
- Stability: thermal, sunlight, metals
- pH
- Dissociation constant
- Viscosity
- Additional physico-chemical information
- Additional physico-chemical properties of nanomaterials
- Nanomaterial agglomeration / aggregation
- Nanomaterial crystalline phase
- Nanomaterial crystallite and grain size
- Nanomaterial aspect ratio / shape
- Nanomaterial specific surface area
- Nanomaterial Zeta potential
- Nanomaterial surface chemistry
- Nanomaterial dustiness
- Nanomaterial porosity
- Nanomaterial pour density
- Nanomaterial photocatalytic activity
- Nanomaterial radical formation potential
- Nanomaterial catalytic activity
- Endpoint summary
- Stability
- Biodegradation
- Bioaccumulation
- Transport and distribution
- Environmental data
- Additional information on environmental fate and behaviour
- Ecotoxicological Summary
- Aquatic toxicity
- Endpoint summary
- Short-term toxicity to fish
- Long-term toxicity to fish
- Short-term toxicity to aquatic invertebrates
- Long-term toxicity to aquatic invertebrates
- Toxicity to aquatic algae and cyanobacteria
- Toxicity to aquatic plants other than algae
- Toxicity to microorganisms
- Endocrine disrupter testing in aquatic vertebrates – in vivo
- Toxicity to other aquatic organisms
- Sediment toxicity
- Terrestrial toxicity
- Biological effects monitoring
- Biotransformation and kinetics
- Additional ecotoxological information
- Toxicological Summary
- Toxicokinetics, metabolism and distribution
- Acute Toxicity
- Irritation / corrosion
- Sensitisation
- Repeated dose toxicity
- Genetic toxicity
- Carcinogenicity
- Toxicity to reproduction
- Specific investigations
- Exposure related observations in humans
- Toxic effects on livestock and pets
- Additional toxicological data

Endpoint summary
Administrative data
Key value for chemical safety assessment
Genetic toxicity in vitro
Link to relevant study records
- Endpoint:
- in vitro gene mutation study in bacteria
- Remarks:
- Type of genotoxicity: gene mutation
- Type of information:
- read-across from supporting substance (structural analogue or surrogate)
- Adequacy of study:
- weight of evidence
- Justification for type of information:
- Substance considered to fall withing the scope of the read-across 'Nanosilver: Justification of a read-across approach for human health hazard endpoints' (document attached in IUCLID section 13).
- Reason / purpose for cross-reference:
- reference to same study
- Reason / purpose for cross-reference:
- read-across source
- Species / strain:
- S. typhimurium TA 100
- Metabolic activation:
- with and without
- Genotoxicity:
- negative
- Cytotoxicity / choice of top concentrations:
- cytotoxicity
- Remarks:
- Please refer to the field "Additional information on results" in the technical dossier.
- Vehicle controls validity:
- not specified
- Untreated negative controls validity:
- not specified
- True negative controls validity:
- not specified
- Positive controls validity:
- not specified
- Species / strain:
- S. typhimurium TA 98
- Metabolic activation:
- with and without
- Genotoxicity:
- negative
- Cytotoxicity / choice of top concentrations:
- cytotoxicity
- Remarks:
- Please refer to the field "Additional information on results" in the technical dossier.
- Vehicle controls validity:
- not specified
- Untreated negative controls validity:
- not specified
- True negative controls validity:
- not specified
- Positive controls validity:
- not specified
- Species / strain:
- S. typhimurium TA 1537
- Metabolic activation:
- with and without
- Genotoxicity:
- negative
- Cytotoxicity / choice of top concentrations:
- cytotoxicity
- Remarks:
- Please refer to the field "Additional information on results" in the technical dossier.
- Vehicle controls validity:
- not specified
- Untreated negative controls validity:
- not specified
- True negative controls validity:
- not specified
- Positive controls validity:
- not specified
- Species / strain:
- S. typhimurium TA 1535
- Metabolic activation:
- with and without
- Genotoxicity:
- negative
- Cytotoxicity / choice of top concentrations:
- cytotoxicity
- Remarks:
- Please refer to the field "Additional information on results" in the technical dossier.
- Vehicle controls validity:
- not specified
- Untreated negative controls validity:
- not specified
- True negative controls validity:
- not specified
- Positive controls validity:
- not specified
- Additional information on results:
- TEST-SPECIFIC CONFOUNDING FACTORS
- Precipitation: precipitation and aggregation of the Ag-NPs were observed at a dose level of more than 125 µg/plate with and without metabolic activation method (Table 1.; please refer "Any other information on results incl.tables").
EXPERIMENT:
When compared with the negative control, no significant number of revertant colonies was observed for any of the bacterial strains with and without the metabolic activation system. Furthermore, no dose-dependent increase of revertant colonies was observed for any of the bacterial strains (Table 1.; please refer "Any other information on results incl. tables"). .
ADDITIONAL INFORMATION ON CYTOTOXICITY:
In the absence of the metabolic activation system (without S9 mix), cytotoxicity was found at ~ 62.5 µg/plate (TA98, TA1535, TA1537 and WP2uvrA) and ~ 31.25 µg/plate (TA100). Plus cytotoxicity was exhibited at concentration of ~125 µg/plate (TA98), TA100 and TA1537) and ~ 250 µg/plate (TA1535 and WP2uvrA) in the presence of the metabolic activation system (Table 1.; please refer "Any other information on results incl. tables"). - Remarks on result:
- other: all strains/cell types tested
- Remarks:
- Migrated from field 'Test system'.
- Conclusions:
- Interpretation of results (migrated information):
negative
When compared with the negative control, no significant number of revertant colonies was observed for any of the bacterial strains with and without the metabolic activation system. Furthermore, no dose-dependent increase of revertant colonies was observed for any of the bacterial strains. - Endpoint:
- in vitro gene mutation study in bacteria
- Remarks:
- Type of genotoxicity: gene mutation
- Type of information:
- read-across from supporting substance (structural analogue or surrogate)
- Adequacy of study:
- weight of evidence
- Justification for type of information:
- Substance considered to fall withing the scope of the read-across 'Nanosilver: Justification of a read-across approach for human health hazard endpoints' (document attached in IUCLID section 13).
- Reason / purpose for cross-reference:
- reference to same study
- Reason / purpose for cross-reference:
- read-across source
- Species / strain:
- S. typhimurium TA 100
- Metabolic activation:
- with and without
- Genotoxicity:
- negative
- Cytotoxicity / choice of top concentrations:
- cytotoxicity
- Remarks:
- at 500µg/plate and higher
- Untreated negative controls validity:
- not specified
- True negative controls validity:
- not specified
- Positive controls validity:
- not specified
- Species / strain:
- S. typhimurium TA 98
- Metabolic activation:
- with and without
- Genotoxicity:
- negative
- Cytotoxicity / choice of top concentrations:
- cytotoxicity
- Remarks:
- at 500µg/plate and higher
- Untreated negative controls validity:
- not specified
- True negative controls validity:
- not specified
- Positive controls validity:
- not specified
- Species / strain:
- S. typhimurium TA 1537
- Metabolic activation:
- with and without
- Genotoxicity:
- negative
- Cytotoxicity / choice of top concentrations:
- cytotoxicity
- Remarks:
- at 500µg/plate and higher
- Untreated negative controls validity:
- not specified
- True negative controls validity:
- not specified
- Positive controls validity:
- not specified
- Species / strain:
- S. typhimurium TA 1535
- Metabolic activation:
- with and without
- Genotoxicity:
- negative
- Cytotoxicity / choice of top concentrations:
- cytotoxicity
- Remarks:
- at 500µg/plate and higher
- Untreated negative controls validity:
- not specified
- True negative controls validity:
- not specified
- Positive controls validity:
- not specified
- Additional information on results:
- CHARACTERIZATION OF SILVER NANOPARTICLES
Silver nanoparticles were characterized by SEM, TEM, and DLS:
- spherical aggregates that were approximately 58.9 nm in size
- single particle size of silver nanoparticles was 100 nm or less.
- although the size distribution of silver nanoparticles by DLS ranged from 45 to 315 nm due to aggregation, 40-59 nm nanoparticles accounted for approximately 50%
- Energy dispersion spectrum: the profile showed strong silver signal along with a weak oxygen and carbon peak, which according to the authors may be derived from the partial oxidation.
AMES TEST
- growth inhibition by silver nanoparticles was observed in all test strains with or without S9 mix at a concentration of 500 μg/plate or lower.
- large increases in the number of revertant colonies were seen for the positive controls in all cases.
- silver nanoparticle mutagenicity was not detected in any strain with or without S9 mix - Remarks on result:
- other: all strains/cell types tested
- Remarks:
- Migrated from field 'Test system'.
- Conclusions:
- Interpretation of results (migrated information):
negative
Growth inhibition by Ag-NPs was observed in all test strains with or without S9 mix at a concentration of 500μg/plate or higher. Increases in the number of revertant colonies were seen for the positive controls in all cases, indicating that the test system responded appropriately. Ag-NP mutagenicity was not detected in any strain with or without S9 mix. Thus, Ag Np are not mutagenic under the conditions of this test. - Endpoint:
- in vitro cytogenicity / chromosome aberration study in mammalian cells
- Remarks:
- Type of genotoxicity: chromosome aberration
- Type of information:
- read-across from supporting substance (structural analogue or surrogate)
- Adequacy of study:
- weight of evidence
- Justification for type of information:
- Substance considered to fall withing the scope of the read-across 'Nanosilver: Justification of a read-across approach for human health hazard endpoints' (document attached in IUCLID section 13).
- Reason / purpose for cross-reference:
- reference to same study
- Reason / purpose for cross-reference:
- read-across source
- Species / strain:
- Chinese hamster Ovary (CHO)
- Metabolic activation:
- with and without
- Genotoxicity:
- negative
- Cytotoxicity / choice of top concentrations:
- cytotoxicity
- Remarks:
- Please refer to the field "Additional information on results" in the technical dossier.
- Vehicle controls validity:
- not specified
- Untreated negative controls validity:
- not specified
- Positive controls validity:
- not specified
- Additional information on results:
- INFORMATION ON CYTOTOXICITY:
The Ag-NPs were found to induce cytotoxicity. For the groups treated for 24 and 6 h without the S9 mix, a cytotoxic effect was induced above 15.625 µg/mL. Meanwhile , for the group treated for 6 h with the S9 mix, cytotoxicity was induced above 31.25 µg/mL (Table 1; please refer to "Any other information on results incl. tables" above).
EXPERIMENT:
Based on the results of the preliminary cytotoxicity test, chromosome slide samples were made using twofold concentrations (3.906, 7.813 and 15.625 µg/mL), with approximately 50% (50± 5%) of the RCC concentration as the highest concentration. No metaphase chromosomes were observed at any of these dose levels after 24 h of continuous treatment without the S9 mix. Also, no metaphase chromosomes were found after 6 h of treatment and 18 h of recovery without the S9 mix at the dose levels of 7.813 and 15.625 µg/mL. Therefore, slide samples were prepared at lower concentrations without the S9 mix, and metaphase chromosome slides examined after 24 h of treatment (0.488, 0.977 and 1.953 µg/mL) and 6 h of treatment (0.977, 1.953 and 3.906 µg/mL). For the CHO-k1 cells, the AG-NPs did not produce any statistically significant increase in the number of cells with chromosome aberrations when compared with the negative control group at any of the dose levels tested with and without metabolic activation. Furthermore, in both the presence and absence of the S9 mix, the AgNPs did not cause a statistically significant increase in the number of cells with polyploidy or endoreduplication when compared with the negative control group (Table 2, 3 and 4; please refer to "Any other information on results incl. tables" above). . - Remarks on result:
- other: all strains/cell types tested
- Remarks:
- Migrated from field 'Test system'.
- Conclusions:
- Interpretation of results (migrated information):
negative
For the CHO-k1 cells, the AG-NPs did not produce any statistically significant increase in the number of cells with chromosome aberrations when compared with the negative control group at any of the dose levels tested with and without metabolic activation. Furthermore, in both the presence and absence of the S9 mix, the AgNPs did not cause a statistically significant increase in the number of cells with polyploidy or endoreduplication when compared with the negative control group. - Endpoint:
- in vitro gene mutation study in bacteria
- Remarks:
- Type of genotoxicity: gene mutation
- Type of information:
- read-across from supporting substance (structural analogue or surrogate)
- Adequacy of study:
- weight of evidence
- Justification for type of information:
- Substance considered to fall withing the scope of the read-across 'Nanosilver: Justification of a read-across approach for human health hazard endpoints' (document attached in IUCLID section 13).
- Reason / purpose for cross-reference:
- reference to same study
- Reason / purpose for cross-reference:
- read-across source
- Species / strain:
- S. typhimurium TA 100
- Metabolic activation:
- without
- Genotoxicity:
- negative
- Remarks:
- Please refer to the field "Additional information on results" in the technical dossier.
- Cytotoxicity / choice of top concentrations:
- cytotoxicity
- Remarks:
- Please refer to the field "Additional information on results" in the technical dossier.
- Vehicle controls validity:
- not specified
- Untreated negative controls validity:
- not specified
- Positive controls validity:
- not specified
- Species / strain:
- S. typhimurium TA 98
- Metabolic activation:
- without
- Genotoxicity:
- negative
- Remarks:
- Please refer to the field "Additional information on results" in the technical dossier.
- Cytotoxicity / choice of top concentrations:
- cytotoxicity
- Remarks:
- Please refer to the field "Additional information on results" in the technical dossier.
- Vehicle controls validity:
- not specified
- Untreated negative controls validity:
- not specified
- Positive controls validity:
- not specified
- Species / strain:
- S. typhimurium TA 1537
- Metabolic activation:
- without
- Genotoxicity:
- negative
- Remarks:
- Please refer to the field "Additional information on results" in the technical dossier.
- Cytotoxicity / choice of top concentrations:
- cytotoxicity
- Remarks:
- Please refer to the field "Additional information on results" in the technical dossier.
- Vehicle controls validity:
- not specified
- Untreated negative controls validity:
- not specified
- Positive controls validity:
- not specified
- Species / strain:
- S. typhimurium TA 1535
- Metabolic activation:
- without
- Genotoxicity:
- negative
- Remarks:
- Please refer to the field "Additional information on results" in the technical dossier.
- Cytotoxicity / choice of top concentrations:
- cytotoxicity
- Remarks:
- Please refer to the field "Additional information on results" in the technical dossier.
- Vehicle controls validity:
- not specified
- Untreated negative controls validity:
- not specified
- Positive controls validity:
- not specified
- Species / strain:
- S. typhimurium TA 102
- Metabolic activation:
- without
- Genotoxicity:
- negative
- Remarks:
- Please refer to the field "Additional information on results" in the technical dossier.
- Cytotoxicity / choice of top concentrations:
- cytotoxicity
- Remarks:
- Please refer to the field "Additional information on results" in the technical dossier.
- Vehicle controls validity:
- not specified
- Untreated negative controls validity:
- not specified
- Positive controls validity:
- not specified
- Additional information on results:
- CHARACTERIZATION OF SILVER NANOPARTICLES
TEM analysis: silver nanoparticle test article had a size range of 4–12 nm.
- 66% of particles measured had diameters in the range of 4 nm to 8 nm, while 24% of particles had diameters of 8–12 nm. In addition, 4% of AgNPs had diameters of 0–4 nm, and 6% of particles were above 12 nm in diameter.
- silver nanoparticle agglomeration occurred in the water diluted samples, producing agglomeration sizes up to 30 nm.
- UV–visible spectroscopy: silver nanoparticle sample had an absorbance maximum at about 450 nm, with a narrow peak width at half maximum
- Zetasizer analysis indicated that the average size of silver nanoparticles in ultrapure water was 61.2 ± 1.6 nm (mean ± SD), and the average size of the particles in culture medium was 1608.7 ± 175.4 (mean ± SD).
- the average surface charge of the silver nanoparticles was −9.37 mV in water and −8.20 mV in media.
- the same sample of silver nanoparticles in water with a size of 61.2 nm by zetasizer analysis was measured as 30 nm agglomerates by TEM.
AMES TEST
- silver nanoparticle treatment of the different strains did not increase the mutant frequencies although the test article showed clear antibacterial ability.
- the doses of silver nanoparticles that were tested showed toxicity for all the tester strains, but individual strains exhibited different levels of sensitivity to the test article.
- TA98 and TA100: frank toxicity (reduction of the background revertant frequency and/or thinning of the background lawn) was detected at 4.8 µg/plate and higher
- TA1535 and TA1537: toxicity was apparent at 9.6 µg/plate and higher.
- at the highest dose of 76.8 µg/plate, all the bacteria from the 5 different strains were killed. - Remarks on result:
- other: all strains/cell types tested
- Remarks:
- Migrated from field 'Test system'.
- Conclusions:
- Interpretation of results (migrated information):
negative without metabolic activation
According to the authors, 5 nm silver nanoparticles did not induce mutations in five different S. typhimurium strains. - Endpoint:
- in vitro cytogenicity / chromosome aberration study in mammalian cells
- Remarks:
- Type of genotoxicity: chromosome aberration
- Type of information:
- read-across from supporting substance (structural analogue or surrogate)
- Adequacy of study:
- weight of evidence
- Justification for type of information:
- Substance considered to fall withing the scope of the read-across 'Nanosilver: Justification of a read-across approach for human health hazard endpoints' (document attached in IUCLID section 13).
- Reason / purpose for cross-reference:
- reference to same study
- Reason / purpose for cross-reference:
- read-across source
- Species / strain:
- other: human bronchial epithelial BEAS 2B cells
- Metabolic activation:
- not specified
- Genotoxicity:
- negative
- Cytotoxicity / choice of top concentrations:
- no cytotoxicity
- Vehicle controls validity:
- not specified
- Untreated negative controls validity:
- not specified
- Positive controls validity:
- valid
- Additional information on results:
- CHARACTERIZATION OF AgNPs:
- transmission electron microscopy: the silver nanoparticle powder mainly appeared as micron-scale aggregates with silver nanoparticles embedded in an organic matrix.
- in situ EDS analysis: silver constituted more than 80 wt.% of the aggregates.
- X-ray diffraction analysis: main phase was pure silver.
- BET analyses: silver nanoparticle powder had a surprisingly low specific surface area of 0.03 m^2/g. The data show that the surfaces of the silver nanoparticles are not readily accessible, at least in the 160 °C degassed powder aggregates.
- TGA-analyses showed that the total amount of evaporable and combustible organic material associated with the silver nanoparticles was 15 ± 2 wt.% (95% confidence limit) corrected for buoyancy. The organic material was shown to decompose in several steps, which indicated the presence of one or more organic compounds with complex molecular chemistry.
- chemical analyses showed that the coating of the silver nanoparticles was PVP.
INFORMATION ON CYTOTOXICITY:
Silver nanoparticles induced a dose-dependent decrease in cell viability at all time points in the Trypan Blue assay. However, only a very slight cytotoxic effect was seen in the luminescent cell viability assay. In the Trypan Blue assay, the 50 ± 5% cytotoxicity limit was reached at 48 µg/cm^2 in the 4-hour treatment, and at 12–48 µg/cm^2 in the 48-hour treatment, whereas in the 24-hour treatment, there was 34.2% cytotoxicity at the highest dose.
However, since the cells began to show a strange appearance (resembling pre-apoptotic cells), 48 µg/cm^2 was chosen as the upper limit for all the treatments.
CHROMOSOMAL ABERRATIONS:
Silver nanoparticles did not increase the rate of chromatid-type, chromosome-type or total chromosomal aberrations at any of the tested doses or time points, and there was no dose-dependent effect for any type of aberrations. Similarly, the mitotic index was not affected by the silver nanoparticles. The results of the chromosomal aberration analysis can be seen in table 1 in the field "Any other information on results incl. tables" below.
The positive control, mitomycin C (50 ng/ml), induced a significant increase in chromosomal aberrations (P < 0.05), except for chromosome-type aberrations, at both exposure times. The mitotic index was not significantly decreased by mitomycin C. - Remarks on result:
- other: all strains/cell types tested
- Remarks:
- Migrated from field 'Test system'.
- Conclusions:
- Interpretation of results (migrated information):
negative
According to the authors, no induction of chromosomal aberrations was observed at any of the doses or time points. - Endpoint:
- in vitro gene mutation study in mammalian cells
- Remarks:
- Type of genotoxicity: gene mutation
- Type of information:
- read-across from supporting substance (structural analogue or surrogate)
- Adequacy of study:
- weight of evidence
- Justification for type of information:
- Substance considered to fall withing the scope of the read-across 'Nanosilver: Justification of a read-across approach for human health hazard endpoints' (document attached in IUCLID section 13).
- Reason / purpose for cross-reference:
- read-across source
- Species / strain:
- mouse lymphoma L5178Y cells
- Metabolic activation:
- without
- Genotoxicity:
- positive
- Remarks:
- An increase in mutant frequency was observed at 1.5 μg/mL, the highest concentration analysed.
- Cytotoxicity / choice of top concentrations:
- cytotoxicity
- Remarks:
- 2 days after treatment the highest 2 concentrations tested in the absence of S9 mix and the highest 4 concentrations tested in the presence of S9 mix were considered too toxic for selection to determine viability and TFT resistance.
- Vehicle controls validity:
- valid
- Untreated negative controls validity:
- not examined
- Positive controls validity:
- valid
- Species / strain:
- mouse lymphoma L5178Y cells
- Metabolic activation:
- with
- Genotoxicity:
- negative
- Remarks:
- In the presence of S9 mix, no marked increases in mutant frequency were observed at any concentration analysed. A weak linear trend was observed, but this observation was not considered biologically relevant.
- Cytotoxicity / choice of top concentrations:
- cytotoxicity
- Remarks:
- 2 days after treatment the highest 2 concentrations tested in the absence of S9 mix and the highest 4 concentrations tested in the presence of S9 mix were considered too toxic for selection to determine viability and TFT resistance.
- Vehicle controls validity:
- valid
- Untreated negative controls validity:
- not examined
- Positive controls validity:
- valid
- Species / strain:
- mouse lymphoma L5178Y cells
- Metabolic activation:
- without
- Genotoxicity:
- positive
- Remarks:
- Increases in mutant frequency were observed at 1.2 and 1.3 μg/mL, respectively.
- Cytotoxicity / choice of top concentrations:
- cytotoxicity
- Remarks:
- 2 days after treatment the highest 2 concentrations tested in the absence of S9 mix and the highest 5 concentrations in the presence of S9 mix were considered too toxic for selection to determine viability and TFT resistance.
- Vehicle controls validity:
- valid
- Untreated negative controls validity:
- not examined
- Positive controls validity:
- valid
- Species / strain:
- mouse lymphoma L5178Y cells
- Metabolic activation:
- with
- Genotoxicity:
- negative
- Remarks:
- In the presence of S9 mix, no marked increases in mutant frequency were observed at any concentration analysed.
- Cytotoxicity / choice of top concentrations:
- cytotoxicity
- Remarks:
- 2 days after treatment the highest 2 concentrations tested in the absence of S9 mix and the highest 5 concentrations in the presence of S9 mix were considered too toxic for selection to determine viability and TFT resistance.
- Vehicle controls validity:
- valid
- Untreated negative controls validity:
- not examined
- Positive controls validity:
- valid
- Species / strain:
- mouse lymphoma L5178Y cells
- Metabolic activation:
- without
- Genotoxicity:
- negative
- Remarks:
- No marked increases in mutant frequency were observed at any concentration analysed.
- Cytotoxicity / choice of top concentrations:
- cytotoxicity
- Remarks:
- 2 days after treatment the highest 3 concentrations tested were considered too toxic for selection to determine viability and TFT resistance.
- Vehicle controls validity:
- valid
- Untreated negative controls validity:
- not examined
- Positive controls validity:
- valid
- Additional information on results:
- TEST-SPECIFIC CONFOUNDING FACTORS
Osmolality and pH measurements on post-treatment incubation medium were taken in the cytotoxicity Range-Finder Experiment.
- Effects of pH: No marked changes in pH were observed in the 3 and 24 hour Range-Finder Experiment at the highest concentration tested (10.0 μg/mL), compared to the concurrent vehicle controls.
- Effects of osmolality: No marked changes in osmolality were observed in the 3 and 24 hour Range-Finder Experiment at the highest concentration tested (10.0 μg/mL), compared to the concurrent vehicle controls.
- Water solubility: Preliminary solubility data indicated that Disilver(I)sulfate was soluble in water at a concentration of approximately 9.590 mg/mL.
- Precipitation: The solubility limit in culture medium was less than 479.5 μg/mL, as indicated by precipitation at this concentration which was present approximately 24 hours after test article addition.
RANGE-FINDING/SCREENING STUDIES: In the cytotoxicity Range-Finder Experiment, 24 hour treatment, 9 concentrations were tested in the absence of S9 mix, ranging from 0.03906 to 10.00 μg/mL. The highest concentration to provide >10% RTG was 0.625 μg/mL, which gave 45% RTG.
COMPARISON WITH HISTORICAL CONTROL DATA: no further data
ADDITIONAL INFORMATION ON CYTOTOXICITY:
- Experiment 1: The highest concentrations selected were 1.5 μg/mL in the absence of S9 mix and 3 μg/mL in the presence of S9 mix, which gave 12% and 5% Relative Total Growth (RTG), respectively. Steep concentration-related toxicity was observed in the presence of S9 mix, such that a concentration of 2.5 μg/mL gave 61% RTG, therefore it was considered prudent to analyse cultures at 2.5 and 3 μg/mL for mutation.
- Experiment 2 (3 hour treatment): The highest concentrations selected in the absence and presence of S9 mix (1.4 and 2.2 μg/mL, respectively) were later rejected from analysis due to extreme toxicity (<10% RTG). The highest concentrations analysed were 1.3 μg/mL in the absence of S9 mix and 2 μg/mL in the presence of S9 mix, which gave 11% and 20% RTG, respectively.
- Experiment 2 (24 hour treatment): The highest two concentrations selected (0.9 and 1 μg/mL) were later rejected from analysis due to extreme toxicity (<10% RTG). The highest concentration analysed was 0.8 μg/mL, which gave 18% RTG. - Remarks on result:
- other: all strains/cell types tested
- Remarks:
- Migrated from field 'Test system'. Remarks: Experiment I, 3 hours treatment
- Conclusions:
- Interpretation of results (migrated information):
positive without metabolic activation
negative with metabolic activation
It is concluded that Disilver(I)sulfate was mutagenic in this test system when tested up to toxic concentrations for 3 hours in the absence of S-9, but was not mutagenic when tested up to toxic concentrations for 3 hours in the presence of S-9 and for 24 hours in the absence of S-9. - Endpoint:
- in vitro cytogenicity / micronucleus study
- Remarks:
- Type of genotoxicity: chromosome aberration
- Type of information:
- read-across from supporting substance (structural analogue or surrogate)
- Adequacy of study:
- weight of evidence
- Justification for type of information:
- Substance considered to fall withing the scope of the read-across 'Nanosilver: Justification of a read-across approach for human health hazard endpoints' (document attached in IUCLID section 13).
- Reason / purpose for cross-reference:
- read-across source
- Species / strain:
- lymphocytes: from humans
- Metabolic activation:
- with and without
- Genotoxicity:
- negative
- Remarks:
- Treatment resulted in frequencies of micronucleated binucleate (MNBN) cells that were generally similar to (and not significantly different from) those observed in concurrent vehicle controls for all concentrations analysed.
- Cytotoxicity / choice of top concentrations:
- cytotoxicity
- Remarks:
- The highest tested concentration in the Main Experiment (120.0 μg/mL) was limited by toxicity (determined in a preliminary cytotoxicity Range-Finder Experiment).
- Vehicle controls validity:
- valid
- Untreated negative controls validity:
- not examined
- Positive controls validity:
- valid
- Additional information on results:
- TEST-SPECIFIC CONFOUNDING FACTORS
Osmolality an pH were measured in the cytotoxicity Range-Finder Experiment.
- Effects of pH: No marked changes in pH were observed at the highest concentration tested (900.0 μg/mL) as compared to the concurrent vehicle controls.
- Effects of osmolality: No marked changes in osmolality were observed at the highest concentration tested (900.0 μg/mL) as compared to the concurrent vehicle controls.
- Water solubility: Preliminary solubility data indicated that Disilver(I)sulfate was soluble in purified water at a concentration of approximately 9.590 mg/mL.
- Precipitation: The solubility limit in culture medium was less than 479.5 μg/mL, as indicated by precipitation at this concentration which persisted for approximately 20 hours after test article addition. A maximum concentration of 900.0 μg/mL was selected for the cytotoxicity Range-Finder Experiment, in order that treatments were performed up to a precipitating concentration.
RANGE-FINDING/SCREENING STUDIES: no further data
COMPARISON WITH HISTORICAL CONTROL DATA: The MNBN cell frequency of all Disilver(I)sulfate treated cultures fell within the observed normal historical ranges.
ADDITIONAL INFORMATION: For the 3+21 hour treatment in the presence of S9 mix, the MNBN cell frequencies in single cultures at 15.0 and 80.0 μg/mL marginally exceeded the 95th percentile of the normal range but both fell within the observed normal range. These observations were not considered biologically relevant. - Remarks on result:
- other: all strains/cell types tested
- Remarks:
- Migrated from field 'Test system'.
- Conclusions:
- Interpretation of results (migrated information):
negative
It is concluded that Disilver(I)sulfate did not induce micronuclei in cultured human peripheral blood lymphocytes in the absence and presence of S-9 when tested up to the limit of cytotoxicity.
Referenceopen allclose all
Table 1. Number of revertant colonies after Ag-NP exposure in absence and presence of S9 mix
|
Type of bacteria (mean ± SD) |
||||
Concentration (µg/plate) |
TA98 |
TA100 |
TA1535 |
TA1537 |
WP2uvrA |
A. Without S9 mix |
|||||
0 |
12 ± 0.6 |
125 ± 6.2 |
13 ± 4.7 |
7 ± 2.1 |
47 ± 7.2 |
0.977 |
10 ± 1.0 |
125 ± 5.1 |
8 ± 3.6 |
8 ± 2.1 |
40 ± 10.6 |
1.953 |
15 ± 0.6 |
124 ± 11.9 |
10 ± 0.6 |
9 ± 3.5 |
48 ± 7.5 |
3.906 |
17 ± 6.1 |
129 ± 12.4 |
8 ± 0.6 |
6 ± 2.3 |
52 ± 10.4 |
7.813 |
15 ± 4.2 |
120 ± 11.3 |
13 ± 3.5 |
6 ± 1.5 |
43 ± 3.1 |
15.625 |
18 ± 8.4 |
123 ± 4.9 |
11 ± 3.2 |
3 ± 1.5 |
51 ± 6.2 |
31.25 |
9 ± 1.5 |
0 ± 0.0 |
10 ± 0.6 |
4 ± 2.1 |
47 ± 4.9 |
62.5 |
0 ± 0.0 |
0 ± 0.0 |
0 ± 0.0 |
0 ± 0.0 |
0 ± 0.0 |
125 |
0 ± 0.0 |
0 ± 0.0 |
0 ± 0.0 |
0 ± 0.0 |
0 ± 0.0 |
Positive control |
501 ± 12.81 |
485 ± 3.22 |
290 ± 16.63 |
2258 ± 173.64 |
637 ± 39.75 |
B. With S9 mix |
|||||
0 |
26 ± 5.0 |
119 ± 6.1 |
12 ± 5.3 |
11 ± 1.5 |
54 ± 2.6 |
7.813 |
22 ± 1.5 |
113 ± 14.2 |
14 ± 4.0 |
8 ± 2.5 |
50 ± 4.5 |
15.625 |
22 ± 7.9 |
126 ± 5.6 |
11 ± 1.2 |
8 ± 1.5 |
51 ± 7.0 |
31.25 |
19 ± 5.1 |
129 ± 15.5 |
12 ± 3.2 |
9 ± 2.3 |
47 ± 1.5 |
62.5 |
17 ± 5.0 |
126 ± 10.5 |
14 ± 4.5 |
8 ± 4.6 |
44 ± 4.5 |
125 |
4 ± 3.2 |
77 ± 2.1 |
8 ± 2.5 |
4 ± 1.2 |
40 ± 9.5 |
250† |
1 ± 0.6 |
3 ± 2.1 |
2 ± 2.1 |
1 ± 0.0 |
12 ± 1.5 |
500† |
0 ± 0.0 |
3 ± 4.9 |
0 ± 0.0 |
0 ± 0.0 |
0 ± 0.0 |
Positive control |
239 ± 13.16 |
462 ± 95.27 |
188 ± 12.28 |
177 ± 19.79 |
284 ± 33.610 |
† = Aggregation and precipitation of test substance
1, 2, and 5 = 2-(2-furyl)-3-(5-nitro-2-furyl) acrylamide
3 = sodium azide
4 = 9-aminoacridine hydrochloride hydrate
6-10 =2-aminoanthracene
Table 1: Test results of RCC for AgNP exposure in absence and presence of S9 mix.
|
RCC (%) |
||
Dose (µg/mL) |
Without S9 mix |
Without S9 mix |
With S9 mix |
Negative control |
100.00 |
100.00 |
100.00 |
7.813 |
70.05 |
77.33 |
83.87 |
15.625 |
49.73 |
53.49 |
81.29 |
31.25 |
20.32 |
34.30 |
54.84 |
62.5 |
0 |
27.33 |
36.77 |
125 |
0 |
0 |
9.68 |
250 |
0 |
0 |
0 |
500 |
0 |
0 |
0 |
1000 |
0 |
0 |
0 |
Cytotoxicity was determined at below around 50% (50 ± 5%) of RCC concentration; RCC = (no. of treated cells/no. of negative control cells) x 100; RCC, relative cell count
Table 2: Number of cells with chromosome aberrations according to hours of AgNP exposure in absence and presence of S9 mix:
Groups treated for 24 h without S9 mix.
|
Type of chromosome aberration |
Total aberrations |
Aberrant cells |
||||||||
Dose (µg/mL) |
No. of cells |
ctb |
cte |
csb |
Cse |
PP |
Gap |
(-)Gap |
(+)Gap |
(-)Gap |
(+)Gap |
N.C |
100 |
0 |
0 |
0 |
0 |
0 |
1 |
0 |
1 |
0 |
1 |
|
100 |
1 |
0 |
0 |
0 |
0 |
0 |
1 |
1 |
1 |
1 |
0.488 |
100 |
0 |
0 |
0 |
0 |
0 |
1 |
0 |
1 |
0 |
1 |
|
100 |
1 |
0 |
0 |
0 |
0 |
0 |
1 |
1 |
1 |
1 |
0.977 |
100 |
0 |
0 |
0 |
0 |
0 |
1 |
0 |
1 |
0 |
1 |
|
100 |
0 |
0 |
0 |
0 |
0 |
0 |
0 |
0 |
0 |
0 |
1.953 |
100 |
0 |
0 |
0 |
0 |
0 |
0 |
0 |
0 |
0 |
0 |
|
100 |
1 |
0 |
0 |
0 |
0 |
0 |
1 |
1 |
1 |
1 |
MMC |
100 |
2 |
28 |
0 |
0 |
0 |
1 |
30 |
31 |
29* |
30 |
|
100 |
7 |
19 |
0 |
0 |
0 |
1 |
26 |
27 |
25* |
26 |
ctb =chromatid-type breakage
cte= chromatid-type exchange
csb = chromosome-type breakage
cse = chromosome-type exchange
MMC = mitomycin C (0.04 µg/mL)
N.C. = negative control
PP = polyploidy
* = Significantly different from negative control at p < 0.05
Table 3: Number of cells with chromosome aberrations according to hours of AgNP exposure in absence and presence of S9 mix:
Groups treated for 6 h without S9 mix
|
Type of chromosome aberration |
Total aberrations |
Aberrant cells |
||||||||
Dose (µg/mL) |
No. of cells |
ctb |
cte |
csb |
Cse |
PP |
Gap |
(-)Gap |
(+)Gap |
(-)Gap |
(+)Gap |
N.C |
100 |
1 |
0 |
0 |
0 |
0 |
0 |
1 |
1 |
1 |
1 |
|
100 |
0 |
0 |
0 |
0 |
0 |
0 |
0 |
1 |
0 |
1 |
0.977 |
100 |
2 |
0 |
0 |
0 |
0 |
0 |
2 |
2 |
2 |
2 |
|
100 |
2 |
0 |
0 |
0 |
0 |
0 |
2 |
2 |
2 |
2 |
1.953 |
100 |
1 |
0 |
0 |
0 |
0 |
0 |
1 |
1 |
1 |
1 |
|
100 |
0 |
0 |
0 |
0 |
0 |
0 |
0 |
0 |
0 |
0 |
3.906 |
100 |
0 |
1 |
0 |
0 |
0 |
0 |
1 |
1 |
1 |
1 |
|
100 |
0 |
2 |
0 |
0 |
0 |
0 |
2 |
2 |
2 |
2 |
MMC |
100 |
4 |
21 |
0 |
0 |
0 |
0 |
25 |
25 |
22* |
22 |
|
100 |
5 |
15 |
0 |
0 |
0 |
1 |
20 |
21 |
20* |
21 |
ctb =chromatid-type breakage
cte= chromatid-type exchange
csb = chromosome-type breakage
cse = chromosome-type exchange
MMC = mitomycin C (0.04 µg/mL)
N.C. = negative control
PP = polyploidy
* = Significantly different from negative control at p < 0.05
Table 4: Number of cells with chromosome aberrations according to hours of AgNP exposure in absence and presence of S9 mix:
Groups treated for 6 h without S9 mix
|
Type of chromosome aberration |
Total aberrations |
Aberrant cells |
||||||||
Dose (µg/mL) |
No. of cells |
ctb |
cte |
csb |
Cse |
PP |
Gap |
(-)Gap |
(+)Gap |
(-)Gap |
(+)Gap |
N.C |
100 |
0 |
0 |
0 |
0 |
0 |
1 |
0 |
1 |
0 |
1 |
|
100 |
1 |
0 |
0 |
0 |
0 |
1 |
1 |
2 |
1 |
2 |
7.813 |
100 |
0 |
0 |
0 |
0 |
0 |
0 |
0 |
0 |
0 |
0 |
|
100 |
1 |
0 |
0 |
0 |
0 |
0 |
1 |
1 |
1 |
1 |
15.625 |
100 |
0 |
0 |
0 |
0 |
0 |
1 |
0 |
1 |
0 |
1 |
|
100 |
1 |
0 |
0 |
0 |
0 |
0 |
1 |
1 |
1 |
1 |
31.25 |
100 |
1 |
0 |
0 |
0 |
0 |
1 |
1 |
2 |
1 |
2 |
|
100 |
0 |
0 |
0 |
0 |
0 |
0 |
0 |
0 |
0 |
0 |
CPA |
100 |
5 |
21 |
0 |
0 |
0 |
1 |
26 |
27 |
25* |
26 |
|
100 |
7 |
23 |
0 |
0 |
0 |
1 |
30 |
31 |
28* |
29 |
ctb =chromatid-type breakage
cte= chromatid-type exchange
csb = chromosome-type breakage
cse = chromosome-type exchange
CPA = cyclophosphamide H2O (10 µg/mL)
N.C. = negative control
PP = polyploidy
* = Significantly different from negative control at p < 0.05
Table 1:
Mean values (SD) of the percentages of cells with chromosomal aberrations (CAs) and mitotic index after exposure of BEAS 2B cells to PVP-coated silver nanoparticles. One hundred cells per replicate (200 per dose) were analyzed for CAs and 500 cells per replicate (1000 per dose) for the mitotic index. Mitomycin C (MMC; 50 ng/ml) was used as a positive control.
Dose (µg/cm²) |
Cells with CA (%) |
Mitotic index (%)a |
|||||||||
|
Chromatid-type |
Chromosome-type |
Total |
||||||||
|
Gaps |
Breaks |
Exchanges |
Total without gapsa |
Total with gapsa |
Breaks |
Markers and dicentrics |
Totala |
Without gapsa |
With gapsa |
|
24h exposure |
|||||||||||
0c |
1.0 (0.0) |
2.0 (2.8) |
0.0 (0.0) |
2.0 (2.8) |
3.0 (2.8) |
0.0 (0.0) |
1.5 (0.7) |
1.5 (0.7) |
3.0 (2.8) |
4.0 (2.8) |
6.2 (1.7) |
2 |
1.5 (0.7) |
1.5 (0.7) |
0.0 (0.0) |
1.5 (0.7) |
3.0 (1.4) |
0.0 (0.0) |
0.5 (0.7) |
0.5 (0.7) |
2.0 (1.4) |
3.5 (2.1) |
3.9 (3.3) |
4 |
2.5 (2.1) |
3.0 (0.0) |
0.0 (0.0) |
3.0 (0.0) |
5.5 (2.1) |
1.0 (1.4) |
0.5 (0.7) |
1.5 (2.1) |
4.5 (2.1) |
7.0 (0.0) |
7.1 (1.3) |
8c |
3.0 (1.4) |
2.5 (0.7) |
0.0 (0.0) |
2.0 (0.0) |
5.0 (1.4) |
1.0 (0.0) |
0.0 (0.0) |
1.0 (0.0) |
3.0 (0.0) |
6.0 (1.4) |
6.8 (0.0) |
16 |
2.0 (1.4) |
2.0 (1.4) |
0.0 (0.0) |
2.0 (1.4) |
4.0 (0.0) |
0.0 (0.0) |
0.0 (0.0) |
0.0 (0.0) |
2.0 (1.4) |
4.0 (0.0) |
6.0 (0.3) |
24 |
1.5 (0.7) |
1.5 (0.7) |
0.0 (0.0) |
1.5 (0.7) |
3.0 (0.0) |
1.0 (0.0) |
0.5 (0.7) |
1.5 (0.7) |
3.0 (0.0) |
4.5 (0.7) |
6.9 (0.4) |
48c,d |
0.9 (1.3) |
3.9 (2.3) |
0.0 (0.0) |
3.9 (2.3) |
4.8 (3.5) |
1.8 (2.5) |
0.0 (0.0) |
1.8 (2.5) |
3.9 (2.3) |
4.8 (3.5) |
6.9 (1.0) |
MMCc |
3.0 (1.4) |
19.0 (0.0) |
6.0 (4.2) |
23.5 (3.5)b |
26.0 (1.4)b |
3.0 (1.4) |
0.0 (0.0) |
3.0 (1.4) |
26.0 (1.4)b |
28.5 (0.7)b |
3.8 (3.4) |
48h exposure |
|||||||||||
0c |
3.0 (1.4) |
2.0 (0.0) |
0.0 (0.0) |
2.0 (0.0) |
4.5 (2.1) |
1.5 (2.1) |
1.0 (1.4) |
2.5 (0.7) |
4.5 (0.7) |
7.0 (1.4) |
1.1 (0.1) |
0.5c |
0.5 (0.7) |
1.0 (0.0) |
0.0 (0.0) |
1.0 (0.0) |
1.5 (0.7) |
1.5 (2.1) |
0.5 (0.7) |
1.5 (0.7) |
2.5 (0.7) |
3.0 (1.4) |
1.1 (0.7) |
1c |
0.5 (0.7) |
1.0 (0.0) |
0.0 (0.0) |
1.0 (0.0) |
1.5 (0.7) |
2.0 (1.4) |
2.0 (1.4) |
2.5 (0.7) |
3.5 (0.7) |
4.0 (1.4) |
1.0 (0.3) |
2c |
1.0 (1.4) |
1.0 (0.0) |
0.0 (0.0) |
1.0 (0.0) |
2.0 (1.4) |
1.0 (0.0) |
1.0 (0.0) |
2.0 (0.0) |
2.5 (0.7) |
3.5 (2.1) |
1.3 (0.7) |
4 |
1.0 (1.4) |
2.5 (2.1) |
0.0 (0.0) |
2.5 (2.1) |
3.5 (0.7) |
0.5 (0.7) |
0.5 (0.7) |
1.0 (1.4) |
3.5 (0.7) |
4.5 (0.7) |
1.5 (0.7) |
6 |
1.5 (2.1) |
2.0 (0.0) |
0.0 (0.0) |
2.0 (0.0) |
3.5 (2.1) |
0.5 (0.7) |
0.0 (0.0) |
0.5 (0.7) |
2.5 (0.7) |
4.0 (1.4) |
2.4 (1.7) |
8d |
0.5 (0.7) |
3.9 (0.2) |
0.0 (0.0) |
3.9 (0.2) |
4.4 (0.9) |
0.5 (0.7) |
1.9 (2.6) |
2.4 (1.9) |
6.2 (1.7) |
6.7 (1.0) |
1.2 (0.6) |
MMCc |
6.5 (3.5) |
25.0 (1.4) |
9.0 (2.8) |
29.5 (0.7)b |
35.0 (2.8)b |
8.0 (1.4) |
0.0 (0.0) |
8.0 (1.4) |
33.5 (0.7)b |
39.0 (2.8)b |
2.5 (1.8) |
aParameters which were statistically analyzed for differences among doses of silver nanoparticles (Kruskal–Wallis test) or MMC (t-test), and dose–response relationship (linear regression analysis).
bP< 0.05, in comparison with untreated control.
cOne cell had multiple CAs; several multi-aberrant cells were seen in cultures treated with MMC.
dLess than 200 metaphases per dose could be scored (99 metaphases for 48µg/cm2 at 24 h, and 154 metaphases for 8 µg/cm2 at 48 h).
Genetic toxicity in vivo
Link to relevant study records
- Endpoint:
- in vivo mammalian somatic cell study: cytogenicity / bone marrow chromosome aberration
- Remarks:
- Type of genotoxicity: chromosome aberration
- Type of information:
- read-across from supporting substance (structural analogue or surrogate)
- Adequacy of study:
- weight of evidence
- Justification for type of information:
- Substance considered to fall withing the scope of the read-across 'Nanosilver: Justification of a read-across approach for human health hazard endpoints' (document attached in IUCLID section 13).
- Reason / purpose for cross-reference:
- reference to same study
- Reason / purpose for cross-reference:
- read-across source
- Sex:
- male
- Genotoxicity:
- positive
- Toxicity:
- not specified
- Vehicle controls validity:
- not specified
- Negative controls validity:
- not specified
- Positive controls validity:
- not specified
- Additional information on results:
- The aberrations scored were mainly found to be of chromatid breaks, while in animals treated with the positive compound (mitomycin C) both chromatid and chromosome type of aberrations were recorded. ANOVA test revealed the frequency of aberrant cells and the number of breaks per cell to be signifcantly higher (P ≤ 0.05) than the control.
Please also refer to the table in the field "Any other information on results incl. tables" below. - Conclusions:
- Interpretation of results (migrated information): positive
According to the authors, silver nanoparticles can be classified as clastogen. The aberrations scored were mainly found to be of chromatid breaks. - Endpoint:
- in vivo mammalian somatic cell study: cytogenicity / erythrocyte micronucleus
- Remarks:
- Type of genotoxicity: chromosome aberration
- Type of information:
- read-across from supporting substance (structural analogue or surrogate)
- Adequacy of study:
- weight of evidence
- Justification for type of information:
- Substance considered to fall withing the scope of the read-across 'Nanosilver: Justification of a read-across approach for human health hazard endpoints' (document attached in IUCLID section 13).
- Reason / purpose for cross-reference:
- read-across source
- Sex:
- male/female
- Genotoxicity:
- negative
- Toxicity:
- no effects
- Remarks:
- No relevant effects on food consumption, bw, organ weights. Slight alterations in haematological parameters at ≥300 mg/kg bw/d. Due to the poor reporting of the histopathology, a definitive conclusion cannot be derived.
- Additional information on results:
- The results of the study showed that silver nanoparticles did not affect either the frequency of micronucleated polychromatic erythrocytes, taken as an indicator of DNA damage, or the PCE/(PCE+NCE) ratio, an indicator of toxicity to bone marrow.
- Conclusions:
- Interpretation of results (migrated information): negative
- Endpoint:
- in vivo mammalian somatic cell study: cytogenicity / erythrocyte micronucleus
- Remarks:
- Type of genotoxicity: chromosome aberration
- Type of information:
- read-across from supporting substance (structural analogue or surrogate)
- Adequacy of study:
- weight of evidence
- Justification for type of information:
- Substance considered to fall withing the scope of the read-across 'Nanosilver: Justification of a read-across approach for human health hazard endpoints' (document attached in IUCLID section 13).
- Reason / purpose for cross-reference:
- reference to same study
- Reason / purpose for cross-reference:
- read-across source
- Sex:
- male/female
- Genotoxicity:
- negative
- Toxicity:
- no effects
- Vehicle controls validity:
- not specified
- Negative controls validity:
- not specified
- Positive controls validity:
- not specified
- Additional information on results:
- - the frequency of micronucleated polychromatic erythrocytes (MN PCEs) in every 2000 PCEs for the male rats was 0.13, 0.21, and 0.18 percent for the groups exposed to low, middle, and high concentrations of silver nanoparticles, respectively, while that for the control was 0.14 percent.
- the frequency of MN PCEs in every 2000 PCEs for the female rats was 0.09, 0.08, and 0.13 for the groups exposed to low, middle, and high concentrations of silver nanoparticles, respectively, while that for the control was 0.14 percent.
- a dose-related increase was found in the number of MN PCEs in the male rats
- no significant treatment-related increase of MN PCEs was detected in the male and female rats when compared to the corresponding negative controls
- no statistically significant difference in the PCE / (PCE + NCE) ratio, representing the absence of bone marrow cytotoxicity, was observed in the male and female rats after silver nanoparticle exposure when compared with the control.
Please also refer to the tables in the field "Any other information on results incl. tables" below. - Conclusions:
- Interpretation of results (migrated information): negative
According to the authors, there were no statistically significant differences in the micronucleated polychromatic erythrocytes or in the ratio of polychromatic erythrocytes among the total erythrocytes after silver nanoparticle exposure when compared with the control. They also stated that the present results suggest that exposure to silver nanoparticles by inhalation for 90 days does not induce genetic toxicity in male and female rat bone marrow in vivo. - Endpoint:
- in vivo mammalian cell study: DNA damage and/or repair
- Remarks:
- Type of genotoxicity: DNA damage and/or repair
- Type of information:
- read-across from supporting substance (structural analogue or surrogate)
- Adequacy of study:
- weight of evidence
- Justification for type of information:
- Substance considered to fall withing the scope of the read-across 'Nanosilver: Justification of a read-across approach for human health hazard endpoints' (document attached in IUCLID section 13).
- Reason / purpose for cross-reference:
- reference to same study
- Reason / purpose for cross-reference:
- read-across source
- Sex:
- male
- Genotoxicity:
- ambiguous
- Toxicity:
- not specified
- Vehicle controls validity:
- not specified
- Negative controls validity:
- not specified
- Positive controls validity:
- not specified
- Additional information on results:
- CHARACTERIZATION OF SILVER NANOPARTICLES
- five nm PVP-coated silver nanoparticles were well distributed and dispersed in water. Agglomeration seldom occurred in the water-diluted samples according to TEM analysis.
- analysis with UV–visible spectroscopy indicated that the 5 nm PVP-coated silver nanoparticles had an absorbance maximum at about 417 nm
- characterization of 5 nm PVP-coated silver nanoparticles was also confirmed and a similar average size of 4.13 ± 1.53 nm was obtained by TEM before treatment.
- average primary size for PVP-coated silver nanoparticles and silicon-coated silver nanoparticles is 51.421.8 nm and 45.520.9 nm by TEM analysis, respectively.
Please also refer to table 1 in the field "Any other information on results incl. tables" below.
TISSUE DISTRIBUTION OF SILVER NANOPARTICLES IN BONE MARROW, PERIPHERAL BLOOD AND LIVER
Distribution analysis of the silver level in bone marrow, peripheral blood and liver was conducted with the mice treated with 15–100 nm PVP- or 10–80 nm silicon-coated silver nanoparticles.
- silver levels in the treated groups increased over the control in both the bone marrow and blood in a dose-dependent manner.
- the amount of silver for PVP-coated silver nanoparticles was detected in a higher concentration in bone marrow (449.40 ± 85.98 ng/mg protein for the single-dose treatment and 470.60 ± 177.5 ng/mg protein for the three-dose treatment) than silicon-coated silver nanoparticles (3.40 ± 0.16 ng/mg protein for the single-dose treatment and 158.20 ± 37.16 ng/mg protein for the three-dose treatment).
- silver level in circulating blood was also elevated with the increase of the dose for the two types of coated silver nanoparticles.
- according ot the authors, the data suggested that the silver nanoparticles circulated in blood and reached the bone marrow, the target tissue for the genotoxicity assays in this study.
- ICP-MS analysis data also showed that a great amount of silver was accumulated in liver after 3-day continuous treatment.
- ICP-MS analysis: the group that repeatedly treated with PVP-coated silver nanoparticles has the highest concentration of silver in bone marrow. Meanwhile, PVP-coated and silicon-coated silver nanoparticles repeatedly treated groups showed a high level of silver element in liver.
- TEM images: no silver nanoparticles were observed by TEM analysis in the bone marrow and liver of the control group, whereas silver nanoparticles were clearly accumulated in bone marrow and liver in the TEM images. These particles in treated samples were confirmed to be silver nanoparticles by the EDS analysis.
Please also refer to table 2 in the field "Any other information on results incl. tables" below.
IN VIVO COMET ASSAY
No DNA strand breaks were detected in liver for both PVP- and silicon-coated AgNPs in the standard Comet assay while significant induction of oxidative DNA damage by the silver nanoparticles treatment were found in the enzyme-modified Comet assay. - Conclusions:
- Interpretation of results (migrated information): ambiguous
According to the authors, no DNA strand breaks were observed in the liver in the standard Comet assay, but PVP- and silicon-coated silver nanoparticles were found to cause DNA breaks in liver in the enzyme-modified Comet assay. - Endpoint:
- in vivo mammalian somatic cell study: cytogenicity / erythrocyte micronucleus
- Remarks:
- Type of genotoxicity: chromosome aberration
- Type of information:
- read-across from supporting substance (structural analogue or surrogate)
- Adequacy of study:
- weight of evidence
- Justification for type of information:
- Substance considered to fall withing the scope of the read-across 'Nanosilver: Justification of a read-across approach for human health hazard endpoints' (document attached in IUCLID section 13).
- Reason / purpose for cross-reference:
- reference to same study
- Reason / purpose for cross-reference:
- read-across source
- Sex:
- male
- Genotoxicity:
- negative
- Toxicity:
- not specified
- Vehicle controls validity:
- not specified
- Negative controls validity:
- not specified
- Positive controls validity:
- not specified
- Additional information on results:
- CHARACTERIZATION OF SILVER NANOPARTICLES
- five nm PVP-coated silver nanoparticles were well distributed and dispersed in water. Agglomeration seldom occurred in the water-diluted samples according to TEM analysis.
- analysis with UV–visible spectroscopy indicated that the 5 nm PVP-coated silver nanoparticles had an absorbance maximum at about 417 nm
- characterization of 5 nm PVP-coated silver nanoparticles was also confirmed and a similar average size of 4.13 ± 1.53 nm was obtained by TEM before treatment.
- average primary size for PVP-coated silver nanoparticles and silicon-coated silver nanoparticles is 51.421.8 nm and 45.520.9 nm by TEM analysis, respectively.
Please also refer to table 1 in the field "Any other information on results incl. tables" below.
TISSUE DISTRIBUTION OF SILVER NANOPARTICLES IN BONE MARROW, PERIPHERAL BLOOD AND LIVER
Distribution analysis of the silver level in bone marrow, peripheral blood and liver was conducted with the mice treated with 15–100 nm PVP- or 10–80 nm silicon-coated silver nanoparticles.
- silver levels in the treated groups increased over the control in both the bone marrow and blood in a dose-dependent manner.
- the amount of silver for PVP-coated silver nanoparticles was detected in a higher concentration in bone marrow (449.40 ± 85.98 ng/mg protein for the single-dose treatment and 470.60 ± 177.5 ng/mg protein for the three-dose treatment) than silicon-coated silver nanoparticles (3.40 ± 0.16 ng/mg protein for the single-dose treatment and 158.20 ± 37.16 ng/mg protein for the three-dose treatment).
- silver level in circulating blood was also elevated with the increase of the dose for the two types of coated silver nanoparticles.
- according ot the authors, the data suggested that the silver nanoparticles circulated in blood and reached the bone marrow, the target tissue for the genotoxicity assays in this study.
- ICP-MS analysis data also showed that a great amount of silver was accumulated in liver after 3-day continuous treatment.
- ICP-MS analysis: the group that repeatedly treated with PVP-coated silver nanoparticles has the highest concentration of silver in bone marrow. Meanwhile, PVP-coated and silicon-coated silver nanoparticles repeatedly treated groups showed a high level of silver element in liver.
- TEM images: no silver nanoparticles were observed by TEM analysis in the bone marrow and liver of the control group, whereas silver nanoparticles were clearly accumulated in bone marrow and liver in the TEM images. These particles in treated samples were confirmed to be silver nanoparticles by the EDS analysis.
Please also refer to table 2 in the field "Any other information on results incl. tables" below.
IN VIVO MICRONUCLEUS ASSAY
- PVP-coated silver nanoparticles (5 nm) caused a statistically significant decrease (maximum 30%) in % reticulocytes at all the concentrations tested from 0.5 to 20 mg/kg, indicating toxicity to the bone marrow .
- 15–100 nm PVP-coated silver nanoparticles caused 33 and 22% reductions in % reticulocytes over the control in the single and repeated treatments, respectively.
- no toxicity to the bone marrow was found following both the single and repeated treatment of silicon-coated silver nanoparticles.
- 5 nm and 15–100 nm PVP-coated silver nanoparticles caused cytotoxicity in the bone marrow
- no increase was observed in reticulocyte micronucleus frequency (% MN-RET) when the treatments were compared with the vehicle control, indicating these silver nanoparticles did not exert genotoxicity in mice by in vivo micronucleus assay.
- the positive control (140 mg/kg) caused a significant reduction in % reticulocytes and a significant increase in % MN-RETs when compared to the control. - Conclusions:
- Interpretation of results (migrated information): negative
According to the authors, PVP-coated silver nanoparticles were more cytotoxic than the silica-coated silver nanoparticles in the bone marrow; but the silver nanoparticles did not induce genotoxicity. According to the authors, silver nanoparticles were confirmed to accumulate in the bone marrow and they also stated that a higher silver level was detected in the bone marrow in mice treated with PVP-coated silver nanoparticles than in the mice treated with the silicon-coated silver nanoparticles.
Referenceopen allclose all
Table 1: Chromosomal aberrations of mice bone marrow cells following treatment with different doses of silver nanoparticles.
Test chemical |
Dose (mg/kg) |
Total chromosome aberrationsa |
% aberrant cellsb |
Number of aberrationc/cell |
||||
G’ |
G’’ |
B’’ |
B’’ |
RR |
||||
Negative control (distilled water) |
- |
6 |
- |
5 |
- |
- |
2.00 ± 0.81 |
0.02 ± 0.01 |
Silver nanoparticles |
10 |
7 |
1 |
41 |
4 |
- |
17.07 ± 4.6* |
0.18 ± 0.01 |
20 |
10 |
3 |
48 |
5 |
- |
19.10 ± 1.01* |
0.21 ± 0.04 |
|
40 |
12 |
1 |
65 |
2 |
- |
23.33 ± 4.38* |
0.27 ± 0.03 |
|
80 |
8 |
3 |
36 |
1 |
- |
15.66 ± 6.48* |
0.15 ± 0.02 |
|
Mitomycin C |
2.5 |
26 |
12 |
130 |
41 |
2 |
17.00 ± 1.08* |
0.59 ± 0.04 |
G'. G'': chromatid and isochromatid gaps; B'. B'': chromatid and isochoromosome breaks; RR: chromatid rearrangements.
a = 50 metaphase cells/animal (5 animals/dose).
b = Percentage of cells with damaged metaphase (excluding gaps).
c = Number of chromosome aberration/cell (excluding gaps)
* P ≤ 0.05
OBSERVATIONS
- all the animals appeared normal and remained healthy until the bone marrow was harvested. There were no significant changes in the body weights of the male rats.
- a dose-dependent deposition of silver nanoparticles was found in the blood, stomach, brain, liver.kidneys, lungs, and testes, indicating that the silver nanoparticles were systemically distirbuted in the mammalian tissues.
Table 1: Frequency of MN PCEs and PCE / (PCE + NCE) ratio in bone marrow of male rats
Dose |
No. of rats (Male) |
Frequency of MN PCEs in every 2000 PCEs (Mean ± SE, %) |
PCE / (PCE + NCE) (Mean ± SE, %) |
0 |
10 |
0.14 ± 0.10 |
0.36 ± 0.10 |
30 |
10 |
0.13 ± 0.09 |
0.39 ± 0.07 |
300 |
10 |
0.21 ± 0.09 |
0.31 ± 0.05 |
1000 |
10 |
0.18 ± 0.13 |
0.30 ± 0.08 |
MN PCE: micronucleated polychromatic erythrocytes
PCE: polychromatic erythrocytes
NCE: normochromatic erythrocytes
Table 2: Frequency of MN PCEs and PCE / (PCE + NCE) ratio in bone marrow of female rats
Dose |
No. of rats (Male) |
Frequency of MN PCEs in every 2000 PCEs (Mean ± SE, %) |
PCE / (PCE + NCE) (Mean ± SE, %) |
0 |
10 |
0.14 ± 0.08 |
0.29 ± 0.08 |
30 |
10 |
0.09 ± 0.06 |
0.30 ± 0.09 |
300 |
10 |
0.08 ± 0.06 |
0.35 ± 0.08 |
1000 |
10 |
0.13 ± 0.10 |
0.31 ± 0.08 |
MN PCE: micronucleated polychromatic erythrocytes
PCE: polychromatic erythrocytes
NCE: normochromatic erythrocytes
Table 1: Characterization of silver nanoparticles
Coating materials |
Size distribution (nm) |
Primary size (nm) |
Hydrodynamic Diameter (nm) |
Maximum Wavelength (nm) |
Zeta Potential (mV) |
PVP |
3–8 |
5.4 ± 1.2 |
N/A* |
417 |
N/A* |
PVP |
15–100 |
51.4 ± 21.8 |
101.6 ± 2.5 |
426 |
-12.4 ± 0.4 |
Silica |
10–80 |
45.5 ± 20.9 |
1343 ± 61.8 |
472 |
-4.04 ± 1.3 |
*The particle size is too small to be suitable for DLS or Zeta Potential analysis due to the detection limits of the current instrumentation for metal nanoparticles.
Table 2: Silver levels in the bone marrow, peripheral blood and liver of mice (n = 5) treated with a dose of 25 mg/kg of PVP- or silicon-coated silver nanoparticles (AgNPs) once or in triplicate for 3 consecutive days.
Treatment group |
Sampling time after final treatment (h) |
Concentration of silver in bone marrow (ng/mg protein) |
Concentration of silver in blood (ng/ml blood) |
Concentration of silver in liver (ng/mg liver) |
Vehicle control |
48 |
3.67 ± 0.01 |
0.07 ± 0.07 |
0.29 ± 0.31 |
Single dose Silicon coated AgNPs |
48 |
3.40 ± 0.16### |
20.67 ± 7.21* |
- |
PVP coated AgNPs |
48 |
449.40 ± 85.98***,### |
35.92 ± 18.87** |
- |
Repeated Dose Silicon coated AgNPs |
48 |
158.20 ± 37.16** |
72.60 ± 18.04*** |
696.64 ± 75.93*** |
PVP coated AgNPs |
48 |
470.60 ± 177.55*** |
65.11 ± 21.54*** |
774.33 ± 104.54*** |
The data are expressed as meanSD.
*, ** and *** indicate p<0.05, p<0.01 and p<0.001, respectively for the treatment group vs. vehicle control group.
### represent p<0.001 for the comparison between silicon-coated and PVP-coated silver nanoparticles at the same treatment schedule.
Table 1: Characterization of silver nanoparticles
Coating materials |
Size distribution (nm) |
Primary size (nm) |
Hydrodynamic Diameter (nm) |
Maximum Wavelength (nm) |
Zeta Potential (mV) |
PVP |
3–8 |
5.4 ± 1.2 |
N/A* |
417 |
N/A* |
PVP |
15–100 |
51.4 ± 21.8 |
101.6 ± 2.5 |
426 |
-12.4 ± 0.4 |
Silica |
10–80 |
45.5 ± 20.9 |
1343 ± 61.8 |
472 |
-4.04 ± 1.3 |
*The particle size is too small to be suitable for DLS or Zeta Potential analysis due to the detection limits of the current instrumentation for metal nanoparticles.
Table 2: Silver levels in the bone marrow, peripheral blood and liver of mice (n = 5) treated with a dose of 25 mg/kg of PVP- or silicon-coated silver nanoparticles (AgNPs) once or in triplicate for 3 consecutive days.
Treatment group |
Sampling time after final treatment (h) |
Concentration of silver in bone marrow (ng/mg protein) |
Concentration of silver in blood (ng/ml blood) |
Concentration of silver in liver (ng/mg liver) |
Vehicle control |
48 |
3.67 ± 0.01 |
0.07 ± 0.07 |
0.29 ± 0.31 |
Single dose Silicon coated AgNPs |
48 |
3.40 ± 0.16### |
20.67 ± 7.21* |
- |
PVP coated AgNPs |
48 |
449.40 ± 85.98***,### |
35.92 ± 18.87** |
- |
Repeated Dose Silicon coated AgNPs |
48 |
158.20 ± 37.16** |
72.60 ± 18.04*** |
696.64 ± 75.93*** |
PVP coated AgNPs |
48 |
470.60 ± 177.55*** |
65.11 ± 21.54*** |
774.33 ± 104.54*** |
The data are expressed as meanSD.
*, ** and *** indicate p<0.05, p<0.01 and p<0.001, respectively for the treatment group vs. vehicle control group.
### represent p<0.001 for the comparison between silicon-coated and PVP-coated silver nanoparticles at the same treatment schedule.
Additional information
Introduction:
Introduction:
The hazard assessment for nanosilver is determined by the extent by which silver ions are released (as determined by in-vivo toxicokinetic data). In this respect, the key data for the hazard assessment of nanosilver is restricted to studies using nanosilver as test item.
The data used for the human health hazard assessment of nanosilver (‘target substances’) are not restricted to studies referring to only these substances as test item. Instead, a more generic approach for the toxicological assessment of “silver” in general is adopted by also including information/data generated with other inorganic silver substances (like silver nitrate or silver sulphate) or organic silver salts (such as silver acetate) that have the potential to release silver ions (‘source substances’) as supporting evidence.
The basic assumption for this is that the systemic toxicity is driven by the released silver ions (Ag+), which is considered the primary relevant species of silver for the hazard assessment. It is noted that many silver substances have a low to negligeable water solubility and are considered of low bioavailability in the body. Once dissolved, the counter-ions released from the silver salt (such as chloride or sulphate anions) are not considered further in the hazard assessment, since these are typically either ubiquitous ions in a physiological environment (like chloride) or generally known to be of no relevant toxicological (systemic) concern (like acetate or sulphate). Note that a different approach is applied if a substance is causing local effects, such as the soluble and corrosive silver nitrate. The read-across approach is used maximally to avoid unnecessary animal testing.
The approach and justification for the applied human health hazard assessment is detailed in the read-across justification document attached to the silver IUCLID file in section 13. The various human health endpoints of the silver REACH dossiers have been updated on an individual basis to reflect the approach that is described in the read-across justification document.
In vitro studies
Bacterial mutagenicity
Silver nanoparticles did not induce gene mutations in an assay with four Salmonella typhimurium (TA1535, TA 1537, TA 95, TA 100) and one E. coli strain when using the pre-incubation method (Kim, J. S. et al., 2012). No statistical increase in revertants was observed in triplicate plating up to concentrations causing cytotoxicity (-S9: 0, 0.977, 1.953, 3.906, 7.813, 15.625, 31.25, 62.5 and 125 µg/plate; +S9: 0, 7.813, 15.625, 31.25, 62.5, 125, 250 and 500 µg/plate) with and without metabolic activation.
The mutagenic potential of silver nanoparticles was also investigated by Kim H. R. et al. (2013) in four Salmonella typhimurium strains (TA1535, TA 1537, TA 95, TA 100) using the plate incorporation method. Bacteria were exposed to five concentrations (100-500µg/plate), showing no increase of revertants either with or without metabolic activation. The authors used commercially available silver nanoparticles, which were not further characterised.
Eliopoulos and Mourelatos (1998) reported a weakly positive finding with silver iodide formulated in a polyacrylamide suspension using the plate incorporation method with four S. typhimurium strains (TA102, TA1535, TA97, TA98) with and without metabolic activation. Concentrations ranged from 10-150µg AgI-polyacrylamide suspension/mL. An increase in revertants was observed in strain TA102 without metabolic activation in two medium doses, and in strain TA97 with metabolic activation in the maximum dose. The authors themselves stated that positive findings were only observed at concentrations toxic to bacteria. Further, the reference does not contain information on the silver iodide purity nor the silver content of the preparation. Based on the above shortcomings, the publication is considered of limited relevance for human health hazard assessment.
Li (2012) investigated the bacterial reverse mutation potential of silver nanoparticles in five Salmonella typhimurium strains (TA98, TA100, TA102, TA1535, TA1537) without metabolic activation. Using the plate incorporation assay up to a concentration of 76.8µg/plate, no increase in revertants was observed. The maximum concentration was limited by toxicity.
Chen et al. (2015) investigated in a combined study silver nanoparticles in three different test systems. The results of the bacterial reverse mutation assay using three Salmonella typhimurium strains (TA98, TA100, TA1535) are reported here. Concentrations ranges from 0.2-1.6µg/plate using the plate incorporation method. The test item (poly(styrene-co-maleic anhydride, SMA)-coated silver nanoparticles) did not show an increase in revertants. The maximum concentrations were limited by cytotoxicity. The reference is considered not reliable, due to several serious shortcomings. The test item description islacking basic information, such as source, physico-chemical characterisation, description of the coating procedure with SMA, analytical information on by-products or impurities. Further, only three strains were used, although the use of five strains is recommended.
In the light of the above publications performed with uncoated silver nanoparticles (Kim, J. S. et al., 2012; Kim H. R. et al., 2013), it was concluded that silver nanoparticles are not mutagenic in the Ames test. Typically, bacterial reverse mutation assays are of limited relevance for metals risk assessment, since the uptake of metal cations by bacteria is considered to be poor, and thus the sensitivity of bacterial test systems for the detection of the mutagenic potential of dissolved metal ions appears to be low (cf. REACH Guidance on information requirements and chemical safety assessment, Chapter R.7a (Version May 2008), Page 390; HERAG fact sheet No 5 Mutagenicity, Chapter 2.1). However, silver is a known bactericidal/bacteriostatic agent with a long history in biocidal applications, such as treatment of burns in patients and in disinfection of water systems. It has been shown that silver cations and silver nanoparticles in fact interact with bacterial cells and become systemically available (R. M. Slawson, 1990; E. Bae, 2011). Consequently, studies on bacterial reverse mutations may be considered relevant for the assessment of genetic toxicity of silver and silver substances.
Several other investigations were not considered since serious shortcomings render these references unsuitable for human health risk assessment purposes.
Chromosomal aberrations (CA) in mammalian cells
Kim, J. S. et al (2013) studied chromosomal aberrations in CHO-K1 cells. Cells were exposed in pulse and continuous treatment with and without metabolic activation to silver nanoparticles (24h –S9: 0.488, 0.977 and 1.953µg/mL, 6h, -S9: 0.977, 1.953, 3.906µg/mL, +S9: 7.813, 15.625, 31.25µg/mL), the concentration range being limited by cytotoxicity. For the CHO-K1 cells, the AG-NPs did not elicit any statistically significant increase in the number of cells with chromosomal aberrations when compared to the negative control group at any of the dose levels tested with and without metabolic activation. Furthermore, in both the presence and absence of the S9 mix, the silver nanoparticles did not cause any statistically significant increase in the number of cells with polyploidy or endoreduplication when compared to the negative control group.
Nymark et al. (2013) studied both chromosomal aberrations and induction of micronuclei (discussed further below) of PVP coated silver nanoparticles (85% silver, 15% PVP coating) in human bronchial epithelial BEAS 2B cells. Treatment was conducted only without metabolic activation for 24 and 48 hours (24 hour exposure: 2, 4, 8, 16, 24 and 48 µg/cm² (corresponding to 10, 20, 40, 80, 120, 180 and 240 µg/mL); 48 hour exposure: 0.5, 1, 2, 4, 6, and 8 µg/cm² (corresponding to 2.5, 5, 10, 20, 30 and 40 µg/mL)), and the maximum concentration was limited by cytotoxicity. Silver nanoparticles did not increase the rate of chromatid-type, chromosome-type or total chromosomal aberrations at any of the tested doses or time points, and there was no dose-dependent effect for any type of aberration. In contrast, the positive control mitomycin C (50 ng/ml) induced a significant increase in chromosomal aberrations at both exposure times.
In a poorly documented reference, the induction of chromosomal aberrations by silver nanoparticles was investigated (Hackenberg et al., 2011). Human adipose-tissue-derived mesenchymal stem cells were exposed for 1 hour to concentrations of 0.01, 0.1, 1, 10µg/mL in distilled water, MMS was used as positive control substance. Cytotoxic effects were assessed by Trypan blue exclusion dye analysis. The authors concluded that a significant increase in CAs could be found at concentrations of 0.1µg/ml and higher with a dose-response relationship, indicating a substance-induced mechanism. The reference exhibits serious shortcomings: (i) exposure duration too short, (ii) number of scored metaphases too low, (iii) unclear which aberrations were scored and were used for the genotoxicity assessment, (iv) unclear whether a confirmatory experiment was performed. Thus, the reference was considered insufficient for consideration in a human health hazard assessment.
Chen et al. (2015) investigated in a combined study silver nanoparticles in three different test systems. The results of the in vitro chromosome aberration test in CHO-K1 cells are reported here. The cells were incubated with SMA coated silver nanoparticles at 1.875, 3.75 and 7.5 µg/mL. It is unclear whether the incubation was performed with or without metabolic activation. after 18-21 hrs incubation cells were harvested, incubated in 0.5% KCl, fixed stained and analysed. At least 100 metaphases were scored for each group. Authors report a significant increase in chromosome aberrations (not further specified) already at the lowest concentration and showing a dose dependent increase. The reference is considered not reliable, due to several serious shortcomings. The description of the experimental procedure is confusing, e.g. it is unclear in which order the cells were treated with test item, control substances or cytokinesis block. It is also not described how the authors ensured a test item carry over to the evaluation procedure. The test item description islacking basic information, such as source, physico-chemical characterisation, description of the coating procedure with SMA, analytical information on by-products or impurities. The aberration frequency in the negative control group (8.7% ±0.6) is 8-10-fold too high, compared with historical data from publications or other labs which is usually in the range of 0.9-1.3% and which would have also been expected for the well-established CHO-K1 cell line. It appears that the authors experienced problems with the stability of the cell line for such a test system and that the results of this assay should be viewed with caution. Finally, authors did not report findings of the cytotoxicity measurements, it remains therefore unclear whether the increased aberration frequency was caused by a direct clastogenic effect of the test item or whether this positive finding was caused by excessive cytotoxicity.
From the chromosomal aberration assays discussed above, it may be concluded by weight-of-evidence that silver nanoparticles are not clastogenic when tested in mammalian cells up to concentrations inducing cytotoxicity. These findings are however somewhat in contradiction to the equivocal findings in the MN assays (reported below).
Induction of micronuclei (MN) in mammalian cells
Nymark et al. (2013) studied both chromosomal aberrations (discussed above) and induction of micronuclei of PVP coated silver nanoparticles (85% silver, 15% PVP coating) in human bronchio-epithelial BEAS 2B cells. Treatment was conducted only without metabolic activation for 48 hours (2, 4, 8, 16, 24, 36 and 48 µg/cm² of silver nanoparticles (corresponding to 10, 20, 40, 80, 120, 180 and 240 µg/mL)), and the maximum concentration was limited by cytotoxicity/morphological alteration of cells. Silver nanoparticles did not increase the number of micronucleated binucleate BEAS 2B cells after the 48-hour exposure at any of the tested doses, and no linear dose-dependence could be observed either. The positive control, mitomycin C (150 ng/mL), significantly increased the number of micronucleated binucleate cells (85.5/1000 binucleate cells, P = 0.03).
In the study by Li et al. (2013), the cytotoxic and genotoxic effects of silver nanoparticles on primary Syrian hamster embryo (SHE) cells were investigated. Cell viability was assessed using methyl tetrazolium (MTT), and genotoxic potential was evaluated in a cytokinesis-block micronucleus (CBMN) assay. Freshly isolated SHE cells were exposed to silver nanoparticles at 10, 20, 40 µg/mL for 24 hours in triplicate. The cytotoxicity was assessed in a separate MTT experiment – no check on the MTT influence of the silver NP was performed. The highest concentration induced cytotoxicity of >60%, which might lead to false positive findings in the micronucleus assay. Silver nanoparticles induced a dose-dependent increase of MN frequency up to a 3-fold in the highest dose. The findings in this study are difficult to assess, since MN induction at the highest dose was measured at a concentration with excessive cytotoxicity. The results of this study are therefore difficult to evaluate and should only be assessed in conjunction with other genotoxicity studies in a weight-of-evidence approach.
In a poorly reported study Kim, H. R. et al. (2013) performed a micronucleus test with and without cytokinesis block using silver nanoparticles. In both assays, cells were treated with an unusual dose selection involving four concentrations (0.01, 0.1, 1, 10µg/mL) for 24 hours. For the assay with cyto-B, the test was performed with and without S9-mix. MN induction was seen in the MN assay and CBMN assay with or without S9 mix. The authors did not report any correlation of the micronuclei findings with cytotoxicity, hence it is not clear whether micronuclei were induced by cytototoxic effects. Based on the lack of cytotoxicity information, this reference is considered of limited relevance for human health risk assessment.
Kawata et al. (2009) performed a micronucleus test with a human hepatoma-derived cell line (HepG2). The cells were exposed to silver nanoparticles at a dose level of 1 mg/L for a duration of 24 hours. Authors observed an increase of MN cells from 2.1% for the control culture to 47.9%. The values reported in this publication appear excessively high: even for the untreated control culture a MN frequency of 2% is highly unusual (a value around 0.4-0.5% would have been expected, Unger et al. 2012). Based on the inadequate reporting and since only one concentration was tested, this reference is considered of limited relevance for human health risk assessment.
Li (2012) investigated the induction of micronuclei in human lymphoblastoid cells (TK6) by silver nanoparticles. The cells were treated with concentrations 10, 15, 20, 25, and 30 µg/mL without metabolic activation in triplicate cultures. The maximum concentration was limited by toxicity, reducing the relative population doubling (RPD) to 40% ±5% (60% ±5% cytotoxicity) which is above the maximum toxicity recommended by the OECD TG 487. The micronucleus analysis was performed via flow cytometry by counting 10000 nuclei, thus the results show a good statistical robustness. Micronucleus frequencies were increased by the silver nanoparticle treatment in a dose-dependent manner, with significant increases in micronuclei measured at both 25 and 30 µg/mL. The 25µg/ml treatment produced a 2.59-fold increase over the vehicle control with a net increase of 1.02% and 30 µg/mL produced a slightly greater response of 3.17-fold over the control with a net increase of 1.60%. Overall, the increase of the MN frequency is weak and the highest MN frequency was evoked at a concentration with excessive cytotoxicity. Since the MN frequency shows a dose-dependency and a significant increase at 25µg/mL at acceptable toxicity, the positive finding can be considered substance-related.
Xu et al. (2012) tested the induction of micronuclei in HeLa cells treated with silver nanoparticles contained in a hydrogel. The preparation contained silver at a concentration of 0.38µg/mL and further unknown ingredients. The authors observed a dose-dependent increase of MN frequency up to the highest concentration of 60mg/mL (it is unclear whether this concentration relates to the preparation or the silver nanoparticles). Authors did not report any toxicity findings, hence it is unclear whether the increase in MN was related to primary effects of the silver nanoparticles or secondary to any toxic effect. Based on the poor reporting quality and the fact that cells were exposed to a silver nanoparticle-containing preparation with unknown composition, this reference is not considered relevant for human health risk assessment purposes.
AshaRani et al. (2009) investigated the induction of micronuclei of starch-coated silver nanoparticles on human glioblastoma cells (U251) and normal human lung fibroblast cells (IMR-90). Cells were treated with dispersed silver nanoparticles at concentrations of 100 and 200 µg/mL for 48 hours without metabolic activation. Metabolic activity was determined in separate experiments after 24, 48 and 72 hrs exposure using the cell titre blue cell viability assay. The results of these experiments were presented only graphically, and are thus difficult to evaluate quantitatively. It was not shown whether the decrease in metabolic activity was related to a cytotoxic mode of action. According to the authors, a significant increase of MN frequency was seen in U251 cells. However, due to (i) the unclear cytotoxicity and (ii) the fact that only two concentrations were tested, this reference is considered of limited relevance for human health hazard assessment purposes.
In an unpublished fully guideline-compliant study (Lloyd, M. 2010) performed according to OECD TG 487 and under GLP, disilver(I) sulfate was tested in an in vitro micronucleus assay using duplicate human lymphocyte cultures prepared from the pooled blood of two male donors in a single experiment. Treatments covering a broad range of concentrations, separated by narrow intervals, were performed both in the absence and presence of metabolic activation (S-9). The test article was formulated in purified water and the highest concentration used in the main experiment, 120 ug/mL (limited by toxicity), was determined following a preliminary cytotoxicity range-finder experiment. Treatments were conducted for 48 hours following mitogen stimulation for 3 hours (with an without S9) and 24 hours (without S9). Micronuclei were analysed at three or four concentrations. Mitomycin C (MMC) and vinblastine (VIN) were employed as clastogenic and aneugenic positive control chemicals, respectively, in the absence of rat liver S-9. Cyclophosphamide (CPA) was employed as a clastogenic positive control chemical in the presence of rat liver S-9. Treatment of cells with disilver(I) sulfate in the absence and presence of metabolic activation (S-9) resulted in frequencies of MNBN cells that were generally similar to (and not significantly different from) those observed in concurrent vehicle controls for all concentrations analysed. The MNBN cell frequency of all disilver(I) sulfate treated cultures fell within the observed normal ranges. It is concluded that disilver(I) sulfate did not induce micronuclei in cultured human peripheral blood lymphocytes in the absence and presence of S-9 when tested up to the limit of cytotoxicity.
The references contained in the summary entry for in vitro micronucleus tests are of limited value for risk assessment purposes. All references do not fulfil the criteria for quality, reliability and adequacy of experimental data for the fulfilment of data requirements and hazard assessment purposes. The information contained therein were included for information purposes only and the deficiencies of the studies are listed below:
Aktepe et al. (2014) reported in a short abstract on the induction of micronuclei in human lymphocytes exposed to silver nanoparticles(not further characterised) at concentrations of 12.5, 50, 250 µg/mL. Authors report an increase in MN frequency in exposed cells, although only one frequency value was reported for the exposed group, although three values should have been expected. Due to the brevity of the abstract, the reference is lacking basic information on experimental conditions, results and discussion. Consequently, the information contained therein are of no relevance for hazard assessment purposes.
Jiang et al. (2013) exposed CHO-K1 cells to BSA coated silver nano particles to concentrations of 1, 5 and 10 µg/mL. Cells were exposed for 24 hrs, fixed, stained and analysed by flow cytometry. Authors report an up to 2.5-fold increase in MN frequency. The reference is lacking basic information on the test item, such as source, physico-chemical characterisation, description of the coating procedure with BSA, analytical information on by-products or impurities. It remains therefore unclear whether any of the findings were elicited by a true test item induced effect, or whether effects were caused by any by-product or impurity. The analysis of MN was conducted using flow-cytometry. The high number of cells measured by this method should lead to statistically robust data with a low SD. However, the results presented here show a high SD. Further, authors did not show any exemplary histogram, showing gating or level of staining. The results therefore remain undocumented. Finally, authors did not check for interference of cell growth with the solid matter. Based on the above given shortcomings, the reference is considered not reliable and of no relevance for hazard assessment purposes.
In summary, there are several publications investigating the induction of MN by silver nanoparticles. One reliable publication on PVP coated silver nanoparticles conclusively shows a complete absence of a clastogenic or aneugenic mode of action. Three publications with limited quality indicate a potential for MN induction by uncoated silver nanoparticles. However, these findings are in contradiction to a substantial body of findings in in-vitro chromosome aberration assays reported above, where no induction of aberration or, as reported in one study, no induction of polyploidy was observed. In a guideline and GLP compliant study, the silver salt disilver(I) sulfate did not induce micronuclei in cultured human peripheral blood lymphocytes in the absence and presence of S-9 when tested up to the limit of cytotoxicity.
Overall, in a conservative approach the in-vitro data on the clastogenic and aneugenic potential of uncoated silver nanoparticles appears to be equivocal.
Gene mutations in mammalian cells
Mei, N. et al. (2012) tested silver nanoparticles for induction of TK mutations in mouse lymphoma cells. Treatment was only for 4 hours in the absence of metabolic activation. Concentrations ranged from 3-6 µg/ml, with a RTG of <10% at the highest dose. The MFs at the concentrations of 4 and 5 µg/mL of the Ag-NPs were 400 ± 230 x 10-6(mean ± SD) and 453 ± 171 x 10-6, respectively, while the MF for the control group was 89 ± 20 x 10-6, clearly exceeding the Global Evaluation Factor (GEF) of +126 mutants per 106cells as recommended by Moore et al. (2006). In the positive cultures, both large and small colony mutants were induced, but the predominant response was induction of small colony mutants (87%), more indicative of a clastogenic (chromosome damaging) effect. Therefore, silver nanoparticles may induce TK mutations in mouse lymphoma cells with the effect being predominantly clastogenic.
Kim Y. J. et al. (2010) tested silver nanoparticles for the induction of TK mutations in L5178Y mouse lymphoma cells. Treatment was only for 3 hours in the presence and absence of metabolic activation (S9). The concentrations ranged from 313 up to 2500 µg/mL (-S9) and 3750µg/mL (+S9), selection made based on the IC20 value determined in a dose range finding experiment. The treatment of silver nanoparticles did not lead to a significant increase of MF at any of the tested concentrations, with or without metabolic activation.
In an unpublished guideline study performed under GLP, disilver(I) sulfate was assayed for its ability to induce mutation at the TK locus (5-trifluorothymidine [TFT] resistance) in mouse lymphoma cells using a fluctuation protocol (Lloyd, M., 2010). In the absence of S-9 (3 hour treatment), when tested up to toxic concentrations in Experiment 1, an increase in mutant frequency (MF) of approximately 129, which marginally exceeded the Global Evaluation Factor (GEF, 126 mutants per 106viable cells) was observed at 1.5 μg/mL, the highest concentration analysed. However, the MF value exceeded the GEF in only one of the replicate cultures at this concentration. When tested up to toxic concentrations in Experiment 2 (3 hour treatment), increases in MF of approximately 168 and 189 (both slightly exceeding the GEF) were observed at 1.2 and 1.3 μg/mL, respectively, and the individual MF values of the replicate cultures exceeded the GEF at both concentrations. Significant linear trends were observed in both experiments. In the absence of S-9 (24 hour treatment), when tested up to toxic concentrations, no marked increases in MF (which exceeded the GEF) were observed at any concentration analysed in Experiment 2. In the presence of S-9, no marked increases in MF (which exceeded the GEF) were observed at any concentration analysed in Experiments 1 and 2. In the positive cultures, both large and small colony mutants were induced, but the predominant response was induction of small colony mutants, indicative of a clastogenic (chromosome damaging) effect. The exceedance of the sum of the mean control MF plus GEF in the second experiment was observed at marketed cytotoxicity with a %RTG of ≤15%. Since these effects are regarded as primarily clastogenic because of colony sizing, it can be concluded that the positive findings are more a direct result of cytotoxicity rather than a clear mutagenic effect of the test item. Further, no positive findings were reported in the continuous treatment over 24 hours, which is considered the most critical treatment regime.
From the references discussed above it can be concluded that silver nanoparticles as well as a soluble silver salts do not induce point mutations, but show a weak clastogenic mode of action in the TK assay with mouse lymphoma cells, observed by small colony formation at concentrations with high cytotoxicity.
Overall, there was no convincing or consistent evidence of induction of gene mutations in either bacterial or mammalian cells in vitro, but an equivocal evidence for a clastogenic mode of action of silver in mammalian cells.
In vitro DNA damage assays
The majority of published experiments on silver substances are investigations of DNA damage of highly variable quality and reliability involving the comet assay as test system. The comet assay is a powerful tool to detect even low levels of DNA damage. However, this assay is also prone to positive findings not caused by the test item but by e. g. inappropriate test design. Minimum quality criteria were therefore used to rate these comet studies for their relevance in chemical safety assessment. The criteria as published by Tice et al. (2000) were used for this screening. In case a reference did not fulfil the criteria stated therein, it was rated as “not rateable” and not further considered for chemical safety assessment purposes.
Nine references, investigating DNA damage via comet assay are available. The following test substances were used:
(i) commercially available silver nanoparticles (≤100nm, Ghosh 2012; 20 and 200nm, Asare 2012; <50nm, Hackenberg 2011, Kim 2013)
(ii) self-synthesised silver nanoparticles by reduction of silver nitrate with sodium borohydrate (Flower 2012)
(iii) PVP coated silver nanoparticles (42.5nm, 85%Ag, 15% residues, Nymark 2013)
(iv) oligonucleotide and lactose modified self-synthesised silver nanoparticles (50nm, Sur 2012)
(v) silver nanoparticles of unknown source and purity (<100nm, Kim 2010).
All references exhibit serious shortcomings in the experimental design and/or the reporting which render them unsuitable for human health risk assessment purposes, such as:
(i) the authors did not correlate DNA damage findings observed in the comet assay with the cytotoxicity at the respective exposure concentration. Thus, it remains unclear whether DNA damage was caused via cytotoxic effects or via direct test item-DNA interaction (Asare 2012, Ghosh 2012, Sur 2012, Cronholm 2013)
(ii) the experimental procedures for cell exposure, staining and scoring were insufficiently or not described, which does not allow an independent evaluation of the results (Hackenberg 2011, Flower 2012, Nymark 2013, AshaRani 2009, Cronholm 2013)
(iii) positive control substances were not used in parallel experiment to assess the sensitivity of the test system (Flower 2012, Cronholm 2013)
(iv) none of the researchers properly considered substance carry-over in the lysis/unwinding/ electrophoresis phase, which might lead to false positive findings due to direct DNA-silver nanoparticle interaction (Kim 2010, Kim 2013)
(v) the substance identity and/or particle size was not conclusively reported. Authors did not demonstrate whether cells were exposed to nanoparticles or whether positive findings were elicited by impurities. This quality criterion was established by the Scientific Committee on Consumer Safety (SCCS) during study evaluation of nano-sized titanium dioxide (“…study is of little value in relation to assessment for nano-form of TiO2 as there is a lack of data on characterisation (particle size distribution) of the tested materials to show that they were nanomaterials. ”, Scientific Committee on Consumer Safety (SCCS) (2013) Opinion on Titanium dioxide (nano form), COLIPA n° S75, document number SCCS/1516/13).
In vivo studies in animals
Micronucleus (MN) test
Kim et al. (2011) investigated the induction of micronuclei in the bone marrow of male and female Sprague-Dawley rats exposed via inhalation to silver nanoparticles for 13 weeks at three dose levels (49, 133 and 515 µg/m³); note: 133µg/m³ is the sub-chronic inhalation NOAEC. The frequency of micronucleated polychromatic erythrocytes (MN PCEs) in every 2000 PCEs for the male rats was 0.13, 0.21, and 0.18 percent for the groups exposed to low, middle, and high concentrations of silver nanoparticles, respectively, while that for the control was 0.14 percent. The frequency of MN PCEs in every 2000 PCEs for the female rats was 0.09, 0.08, and 0.13 for the groups exposed to low, middle, and high concentrations of silver nanoparticles, respectively, while that for the control was 0.14 percent. No significant treatment-related increase of MN PCEs was detected in the male and female rats when compared to the corresponding negative controls. A dose-dependent deposition of silver nanoparticles was found in various tissues, indicating that the silver was systemically distributed throughout the test organism. The results suggest that exposure to silver nanoparticles by inhalation for 90 days does not induce genetic toxicity in male and female rat bone marrow in vivo. Systemic exposure was shown by increased silver tissue levels.
In a micronucleus test, 10 male and female Sprague-Dawley rats were exposed orally via gavage to silver nanoparticles at doses of 30, 300 and 1000 mg/kg bw/day over a period of 28-days. No increase of the micronucleated polychromatic erythrocyte frequency was observed (Kim, Y. S. et al., 2008). Systemic exposure was shown by increased silver tissue levels, however direct bone marrow exposure was not analytically verified in this particular study.
Ghosh et al. (2012) investigated the induction of chromosomal aberrations in the bone marrow of male Swiss albino mice following intraperitoneal administration of silver nanoparticles at 10, 20, 40 and 80mg/kg bw. It was found that silver nanoparticles significantly increased the number of chromosome damages, mainly chromatid breaks.
Chen et al. (2015) investigated in a combined study silver nanoparticles in three different test systems. The results of the in vivo micronucleus test in male ICR mice intraperitoneally injected with SMA coated silver nanoparticles. The six week old animals were divided in three groups of 5 animals each, injected with 0.25 and 1 mg/kg SMA coated silver nanoparticles. Positive control animals received cyclophosphamide. A negative control group was presumably included, but details were not provided in the methods description. Peripheral blood samples were drawn via retro-orbital bleeding 48 and 72 hrs after exposure. Cells were stained, and a total of 1000 RET were scored for MN. For the PCE/NCE ratio 1000 cells were scored. No increase in the MN frequency was seen after 48 or 72 hours. The MN frequencies in the negative control and treated animals were within the historical control range of published data or other labs. The reference is considered not reliable, due to several serious shortcomings. The description of the experimental procedure is confusing, e.g. it is how many animals were assigned to how many groups – the method described three groups with 5 animals each, the results report 4 groups with unknown number of animals. The experimental procedure is described very briefly, so that the cell preparation procedure remains unclear. The number of RET scored for presence of MN is too low, compared with the guideline recommendation. The test item description islacking basic information, such as source, physico-chemical characterisation, description of the coating procedure with SMA, analytical information on by-products or impurities.
The increase of micronuclei frequency was investigated by Kovvuru et al. (2015) in C57BL/6J pun/punmice (4 - 6 mice/group, in equal proportions of males and females) following oral administration of 500 mg/kg bw/day PVP coated silver nanoparticles over 5 days. Peripheral blood was taken from treated and control animals via sub-mandibular bleeding 24 hours after one daily dose and 24 hours after five daily doses. Bone marrow samples were collected 24 hours after five daily doses. At least 4000 peripheral blood erythrocytes and 2000 bone marrow erythrocytes were analysed per mouse. According to the authors, PVP-coated silver nanoparticles induced chromosomal damage in bone marrow and peripheral blood. However, the results of this study cannot be evaluated, due to reporting deficiencies and shortcomings in the experimental design. The test item was insufficiently characterised, the presence of PVP-coated silver nanoparticles was not sufficiently demonstrated. The number of animals per group was too low compared with the guideline recommendation, which reduces the statistical robustness of the results. Only one dose was used during testing, which does not allow to investigate a dose-response relationship as recommended by the guideline. In addition, no positive control was used in the study design. The negative control MN frequency was in an unusually very low range (0.025-0.1 %), compared with published historical control data (0.1-0.35%) and the MN frequencies were within the range of published historical negative control data (0.25-0.35%). It appears that authors concluded on a positive finding due to the unusual low MN frequency in the negative control animals. Since a positive control was not used in these experiments, the selectivity of the system was not demonstrated. The statistically significant positive findings in the treated animals might therefore be an accidental finding without biological relevance. Finally, authors did not report any markers of cytotoxicity, thus findings might as well have been caused by elevated cytotoxicity and not a true clastogenic response. Overall, the results were only presented in graphs and raw data was not available. Consequently, the reference is rated not reliable and considered unsuitable for hazard assessment purposes.
Chromosome aberration test
El Mahdy et al. (2014) conducted a chromosome aberration study with self-synthesised silver nanoparticles using mature female albino rats. Groups of five rats received doses of 1, 2 and 4 mg/kg bw/day via intraperitoneal injection for 28 days, negative control group was run concurrently. A positive control group was not included. Chromosomal aberration was measured in bone marrow at the end of the treatment period. At least 250 metaphases of each animal were scored, characterising different types of chromosomal aberrations. The reference exhibits serious shortcomings which renders it unsuitable for its use in hazard or risk assessment. Authors did not provide information on: (i) rat strain used in the experiments, (ii) the dose setting or MTD and provide no data on any clinical signs during the course of the study (iii) historical positive or negative control data, thus eligibility of the lab or the rat strain used in the CA experiments were not documented. No positive control group was added in the study, thus the sensitivity of the test system remains undocumented. The test item was given via intraperitoneal injection. Such an non-physiological route of administration is not suitable for the hazard assessment of industrial chemicals and is not compliant with the guideline recommendation (OECD TG 475) which clearly states that “intraperitoneal injection is generally not recommended since it is not an intended route of human exposure”.Finally, the test item description islacking basic information, such as source, physico-chemical characterisation and analytical information on by-products or impurities. It remains therefore unclear whether the cytotoxicity and DNA damage was caused by the test item itself or by any by-product or impurity. Self-synthesised nanoparticles have also not relevance for the risk assessment of industrially manufactured nanomaterials with clearly defined physico-chemical characteristics and analytically verified impurity profiles.Consequently, the reference is rated not reliable and considered unsuitable for hazard assessment purposes.
DNA damage (Comet assay) test
The in vivo comet assay study design was checked against the OECD test guideline 489 (26 September 2014), which is based on internationally agreed study designs suggested by various authors in the public domain (e.g. Kirkland 2008, Brendler-Schwaab 2005, Burlinson 2007 and 2012, Smith 2008, Hartmann 2003, McKelvey-Martin 1993, Tice 2000, Singh 1998, Rothfuss 2010). Although the OECD guideline was published after publication of some references summarised below, the comparison with OECD 489 is appropriate, since the majority of the underlying protocols existed at the time of study planning and conduct.
Li, Y. et al. (2013) investigated the induction of DNA damage via comet assay and micronuclei in the bone marrow of B6C3F1 mice. Five male animals were intravenously injected with a single or a repeated dose for 3 consecutive days of 0.5-20 mg/kg bw with (i) PVP-coated and (ii) silicon coated silver nanoparticles. Target organ exposure was analytically verified via ICP-MS analysis. Bone marrow toxicity was only observed in the test group exposed to PVP coated silver nanoparticles. None of the treatments resulted in a significant increase of the percent of micronucleated reticulocyte over the concurrent controls. For the DNA damage analysis, liver samples were collected from mice treated with 25 mg/kg bw PVP- or silicon coated silver nanoparticles, or the vehicle for 3 consecutive days and assayed for DNA damage 3 h after the last treatment. No DNA strand breaks were detected in liver for both PVP- and silicon-coated silver nanoparticles in the standard comet assay while significant induction of oxidative DNA damage was found in the hOGG1-modified comet assay. It remains unclear why the standard alkaline comet assay did not detect any DNA damage whereas the modified comet assay (specific for oxidative DNA strand breaks) was positive.
In a disregarded neutral comet assay, DNA damage was observed in the spleen of male and female Balb/c mice treated via intraperitoneal injection of a single silver nanoparticle dose. The unit of the dose was reported as g Ag/L, due to the lack of body weight information the actual administered dose could not be calculated (Ordzhonikidze et al., 2009).
Dobrzyńska, M. et al. (2014) performed a combined micronucleus test and comet assay in male Wistar rats. Rats were injected intravenously with a single dose of 5 or 10 mg/kg bw of 20 nm silver nanoparticles or with 5 mg/kg bw 200 nm silver nanoparticles. The samples for the comet assay were taken at 24 hours, 1 week and 4 weeks following the exposure. The study showed that silver nanoparticles did not cause cytotoxicity to bone marrow white blood cells. Moreover, the results with the comet assay did not show any significant increase of DNA damage in bone marrow leukocytes of rats. This lack of observations is valid for all time points post single treatment with silver nanoparticles. In silver-nanoparticle-treated animals, a significant increase in the number of micronuclei per 1000 PCE was observed at 24 hours after exposure independent of dose and particle size. One week after the exposure, the significantly enhanced as compared to the negative control levels of micronuclei was noted in all silver nanoparticles exposed groups. Four weeks following the single exposure still the significantly higher frequency of micronuclei was observed only in animals administered to 10 mg/kg silver nanoparticles of 20 nm size. The reference shows inconsistencies in the reporting of the genetic toxicity findings, two tables report scoring results for MN cells, namely MN-PCE and MN-Reticulocytes. Since only one MN experiment was performed, it remains unclear why two tables are reporting results for the identical cell type but with different scoring results.
An unequivocally negative outcome was seen in rats and mice following repeated exposures to silver nanoparticles via inhalation (90-days, with exposures beyond the NOAEC; Kim, Y. S. et al., 2008) and oral administration (28-days, with dosing up to the limit dose, i. e. 1,000 mg/kg bw/d; Kim et al., 2011). The induction of chromosomal aberrations by silver nanoparticles reported by Ghosh et al. (2012) and Dobrzyńska, M. et al. (2014) as well the DNA damages reported by Ordzhonikidze et al. (2009) and Dobrzyńska, M. et al. (2014) were only observed after a non-physiological route of exposure. Thus, the results are considered to lack direct relevance for human health risk assessment purposes for industrial chemicals under REACH. Experiments with PVP- and silicone-coated silver nanoparticles showed a negative outcome in the in vivo MN assay in bone marrow in mice exposure via single and repeated intravenous injection. The standard alkaline comet assay in liver was negative, whereas a modified hOGG1-comet assay indicated oxidative DNA strand breaks – which remained unexplained by the authors.
Awasthi et al. (2015) examined the induction of DNA damage in the liver of male mice after oral administration of self-synthesised silver nanoparticles. Animals (6 males per group) were given (i) a single dose of 50 and 100 mg/kg and (ii) doses of 10 and 20 mg/kg bw/day over 5 weeks via gavage. Animals were sacrificed 3 or 24 hrs after single and 3 hrs after the last of repeated administrations, respectively. Triton X-100 was administered as vehicle control, negative control animals received no treatment, positive control animals received 25 mg/kg cyclophosphamide via oral route. After sacrifice, animals were dissected and single liver cell suspensions were prepared. In addition liver slides were prepared for histopathological examination. Liver function was determined by AST, ALT and ALP measurements in serum. For the comet analysis cells were fixed in low-melting agarose, unwinded and lysed in alkaline buffer. After electrophoresis, slides were stained with ethidium bromide. DNA migration was determined in 100 cells per group via tail length, %DNA in tail and tail moment. Authors observed a dose dependent increase of DNA damage after single and repeated administration. In the histopathological examination treated animals showed hepatic congestion and haemorrhage. All three enzyme levels (AST, ALT and ALP) were increased in the treated animals, indicating cytotoxicity to the liver. According to the authors, silver nanoparticles has the potential to cause genetic damage. However, the results of this study cannot be evaluated, due to shortcomings in the experimental design. First of all, changes in DNA migration observed at doses also show marketed toxic effects in the target tissue (i.e. AST, ALT and ALP increased, haemorrhage in liver). The number of cells analysed (100 cells per group of 6 animals) was significantly too low, compared with the guideline recommendation (150 cells per animal). Conflicting information were presented on how the positive control was administered. Overall, a substance specific induction of DNA damage has not been demonstrated in this study. Finally, the test item description islacking basic information, such as source, physico-chemical characterisation, analytical information on by-products or impurities. It remains therefore unclear whether the cytotoxicity and DNA damage was caused by the test item itself or by any by-product or impurity. Self-synthesised nanoparticles have also not relevance for the risk assessment of industrially manufactured nanomaterials with clearly defined physico-chemical characteristics and analytically verified impurity profiles.Consequently, the reference is rated not reliable and considered unsuitable for hazard assessment purposes.
Al Gurabi et al. (2015) examined the induction of DNA damage in the liver of Swiss albino mice (sex not reported) after intraperitoneal injection of self-synthesised silver nanoparticles. Animals (unclear number of animals per sex and group) were given the test item for 24 and 72 hours at 26, 52, and 78 mg/kg bw silver nanoparticles. The frequency of treatment is unclear, since no further information on the treatment conditions was given. Vehicle control animals received water, no positive control animals were included. Blood samples were drawn and lymphocytes separated from the whole blood. For the comet analysis cells were fixed in low-melting agarose, unwinded and lysed in alkaline buffer. After electrophoresis, slides were stained with ethidium bromide. DNA migration was determined %DNA in tail in 250 cells per treatment group. For the positive control ex-vivo liver cells were treated with 100µM H2O2 for 10 minutes.The authors reported the induction of DNA damage in lymphocytes of mice treated with silver nanoparticles. However, the results of this study cannot be evaluated, due to severe reporting deficiencies and shortcomings in the experimental design. The dosing regimen of the animals is unclear (only treatment for 24 or 72 hrs was reported, without indication of the treatment frequency – it is unclear how to treat animals via i.p. injection over a period of 24 or 72 hrs) as well as the number and sex of animals used during testing. In addition, no valid in vivo positive control was used. Instead ex-vivo liver cells were treated with H2O2, which is not an accepted positive control substance, an ex-vivo treatment of cells is also not a valid method for an in vivo study positive control. Microscopic pictures and fluorescent pictures of comets were of poor quality and therefore unsuitable for assessment. Poor picture quality raises doubts on the percentage tail DNA evaluation.Finally, the test item description islacking basic information, such as source, physico-chemical characterisation, analytical information on by-products or impurities. It remains therefore unclear whether the cytotoxicity and DNA damage was caused by the test item itself or by any by-product or impurity. Self-synthesised nanoparticles have also no relevance for the risk assessment of industrially manufactured nanomaterials with clearly defined physico-chemical characteristics and analytically verified impurity profiles.Consequently, the reference is rated not reliable and considered unsuitable for hazard assessment purposes.
In vivo studies in humans
In the study by Aktepe et al. (2015) the genotoxic effect of silver particle exposure among silver jewellery workers was investigated. The silver jewellery workers were assumingly exposed to the silver particles by inhalation. DNA damage in peripheral mononuclear leukocytes was measured by using the alkaline comet assay. According to the authors, exposure to silver particles by inhalation caused significant genotoxicity in mononuclear leukocytes in silver jewellery workers. This publication has a low relevance for human risk assessment, since exposure conditions were not determined. It is unknown to which substances the jewellery workers were expose to and the duration and concentration of exposure is not known. Furthermore, confounding factors were not considered at all, such as sex, smoking habits, alcohol consumption, recreational behaviour, medication. No significant correlation between (assumed) silver exposure and presence of DNA damage could be shown by the authors.
Conclusion
The available data on genetic toxicity allow a conclusive statement on the genetic toxicity for silver and silver substances (including nanoparticles). Irrespective of the reporting quality of the publications, initially positive as well as negative findings are reported in in vitro as well as in vivo test systems.
Following rigorous relevance and reliability screening, it can be concluded that silver nanoparticles show a clastogenic potential in mammalian cells in-vitro, but at concentrations with high levels of cytotoxicity. In contrast, high-quality in vivo studies conversely do not report any clastogenic or aneugenic effects after silver nanoparticle exposure via physiologically relevant routes of exposure (i. e. via inhalation or oral routes of administration).
Consequently, the positive in vitro clastogenicity findings are conclusively superseded by the negative in vivo findings. Further, there is convincing evidence that silver nanoparticles do not induce gene mutations either in bacterial or in mammalian cells.
Overall, there is no consistent evidence of induction of genetic toxicity with relevance to humans for silver and silver substances.
References
Slawson, RM, H. Lee, H, Trevors, JT (1990) Bacterial interactions with silver, Biology of Metals, 3(3-4), 151-154
Bae, E. et al. (2011) Bacterial uptake of silver nanoparticles in the presence of humic acid and AgNO3, Korean J. Chem. Eng., 28(1), 267-271
Unger, P., Melzig F. (2013) Comparative Study of the Cytotoxicity and Genotoxicity of Alpha- and Beta-Asarone, Sci Pharm. 2012; 80: 663–668
Kirkland, D., G. Speit (2008), Evaluation of the ability of a battery of three in vitro genotoxicity tests to discriminate rodent carcinogens and non-carcinogens III. Appropriate follow-up testing in vivo, Mutation Research, Vol. 654/2, pp. 114-32.
Brendler-Schwaab,S. et al. (2005), The in vivo Comet assay: use and status in genotoxicity testing, Mutagenesis, Vol. 20/4, pp. 245-54.
Burlinson, B. et al. (2007), Fourth International Workgroup on Genotoxicity Testing: result of the in vivo Comet assay workgroup, Mutation Research, Vol. 627/1, pp. 31-5.
Burlinson,B. (2012), The in vitro and in vivo Comet assays, Methods in Molecular Biology, Vol. 817, pp.143-63.
Smith,C.C, et al. (2008), Recommendations for design of the rat Comet assay, Mutagenesis, Vol. 23/3, pp. 233-40.
Hartmann, A.et al. (2003), Recommendations for conducting the in vivo alkaline Comet assay, Mutagenesis, Vol. 18/1, pp. 45-51.
McKelvey-Martin, V.J. et al (1993), The single cell gel electrophoresis assay (Comet assay): a European review, Mutation Research, Vol. 288/1, pp. 47-63.
Tice, R.R.et al.(2000), Single cell gel/Comet assay: guidelines for in vitro and in vivo genetic toxicology testing, Environmental and Molecular Mutagenesis, Vol. 35/3, pp. 206-21.
Singh, N.P.et al. (1988),A simple technique for quantitation of low levels of DNA damage in individual cells, Experimental Cell Research, Vol. 175/1, pp. 184-91.
Rothfuss, A. et al. (2010), Collaborative study on fifteen compounds in the rat-liver Comet assay integrated into 2-and 4-week repeat-dose studies, Mutation Research, Vol., 702/1, pp. 40-69.
Short description of key information:
By applying a weight-of-evidence approach, after consideration of the predominantly negative test results of highly reliable in-vitro and in-vivo genotoxicity assays and their route of administration, the overall conclusion is reached that no genotoxicity needs to be expected from exposure to silver substances, regardless of whether they were administered in ionic form or as nanomaterials.
Endpoint Conclusion: No adverse effect observed (negative)
Justification for classification or non-classification
In consideration of the predominantly negative test results in highly reliable genotoxicity assays, no genotoxicity needs to be expected from exposure to silver substances, regardless of whether they were administered in ionic form or as nanomaterials. In consequence, no classification is required.
Information on Registered Substances comes from registration dossiers which have been assigned a registration number. The assignment of a registration number does however not guarantee that the information in the dossier is correct or that the dossier is compliant with Regulation (EC) No 1907/2006 (the REACH Regulation). This information has not been reviewed or verified by the Agency or any other authority. The content is subject to change without prior notice.
Reproduction or further distribution of this information may be subject to copyright protection. Use of the information without obtaining the permission from the owner(s) of the respective information might violate the rights of the owner.
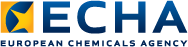