Registration Dossier
Registration Dossier
Data platform availability banner - registered substances factsheets
Please be aware that this old REACH registration data factsheet is no longer maintained; it remains frozen as of 19th May 2023.
The new ECHA CHEM database has been released by ECHA, and it now contains all REACH registration data. There are more details on the transition of ECHA's published data to ECHA CHEM here.
Diss Factsheets
Use of this information is subject to copyright laws and may require the permission of the owner of the information, as described in the ECHA Legal Notice.
EC number: 237-928-2 | CAS number: 14075-53-7
- Life Cycle description
- Uses advised against
- Endpoint summary
- Appearance / physical state / colour
- Melting point / freezing point
- Boiling point
- Density
- Particle size distribution (Granulometry)
- Vapour pressure
- Partition coefficient
- Water solubility
- Solubility in organic solvents / fat solubility
- Surface tension
- Flash point
- Auto flammability
- Flammability
- Explosiveness
- Oxidising properties
- Oxidation reduction potential
- Stability in organic solvents and identity of relevant degradation products
- Storage stability and reactivity towards container material
- Stability: thermal, sunlight, metals
- pH
- Dissociation constant
- Viscosity
- Additional physico-chemical information
- Additional physico-chemical properties of nanomaterials
- Nanomaterial agglomeration / aggregation
- Nanomaterial crystalline phase
- Nanomaterial crystallite and grain size
- Nanomaterial aspect ratio / shape
- Nanomaterial specific surface area
- Nanomaterial Zeta potential
- Nanomaterial surface chemistry
- Nanomaterial dustiness
- Nanomaterial porosity
- Nanomaterial pour density
- Nanomaterial photocatalytic activity
- Nanomaterial radical formation potential
- Nanomaterial catalytic activity
- Endpoint summary
- Stability
- Biodegradation
- Bioaccumulation
- Transport and distribution
- Environmental data
- Additional information on environmental fate and behaviour
- Ecotoxicological Summary
- Aquatic toxicity
- Endpoint summary
- Short-term toxicity to fish
- Long-term toxicity to fish
- Short-term toxicity to aquatic invertebrates
- Long-term toxicity to aquatic invertebrates
- Toxicity to aquatic algae and cyanobacteria
- Toxicity to aquatic plants other than algae
- Toxicity to microorganisms
- Endocrine disrupter testing in aquatic vertebrates – in vivo
- Toxicity to other aquatic organisms
- Sediment toxicity
- Terrestrial toxicity
- Biological effects monitoring
- Biotransformation and kinetics
- Additional ecotoxological information
- Toxicological Summary
- Toxicokinetics, metabolism and distribution
- Acute Toxicity
- Irritation / corrosion
- Sensitisation
- Repeated dose toxicity
- Genetic toxicity
- Carcinogenicity
- Toxicity to reproduction
- Specific investigations
- Exposure related observations in humans
- Toxic effects on livestock and pets
- Additional toxicological data

Hydrolysis
Administrative data
Link to relevant study record(s)
Description of key information
The hydrolysis of BF4− occurs stepwise to BF3OH−, BF2(OH)2− , and BF(OH)3− and ultimately B(OH)4− accompanied with the formation of hydrofluoric acid.
Due to the strong affinity of boron for fluoride, complete hydrolysis of BF4- occurs at low concentrations, yet with a slow initial step, especially at normal temperature and at a neutral or alkaline pH.
Key value for chemical safety assessment
Additional information
Hydrolysis : weight of evidence approach
The fluoroboric anion is stable in concentrated solutions, and hydrolyses slowly in aqueous solution to hydroxyfluoroborates. Wamser described the hydrolysis reaction of fluoroboric acid (HBF4 + H2O ↔ HBF3OH + HF) and determined the equilibrium constants for the hydrolysis at 25°C. The hydrolysis of aqueous fluoroboric acid solutions (at equilibrium) was measured over the concentration range 0.001M to 5.41M, the % hydrolysis at equilibrium ranged from 77.7% to 5.47% respectively and the equilibrium constant between 2.75E-3 to 17.6E-3 respectively. At 0.01M HBF4 the hydrolysis was about 35% and the equilibrium constant 1.98E-3 (Wamser, 1948).
The equilibrium quotient Q in 1 molal NaCl at 25°C shows the strong affinitiy of boron for fluoride (Papcun, 2000).
B(OH)3 + 4 F− + 3 H+ ↔ BF4− + 3 H2O logQ = 19.0 ± 0.1 (Mesmer, Palen, & Baes Jr., 1973)
The hydrolysis of BF4− occurs stepwise to BF3OH− , BF2(OH)2− , and BF(OH)3− and ultimately B(OH)4− accompanied with the formation of hydrofluoric acid. Studies demonstrated that BF4− is very stable to hydrolysis, but is slow to form from BF3OH−and HF. Kinetic results and 19F NMR experiments illustrated that the hydroxyfluoroborates are in rapid equilibrium and easily exchange fluoride (Mesmer et al., 1973; Wamser, 1951). By boiling solutions containing NaBF4 in the presence of calcium carbonate a small amount of hydrolysis was effected and the 19F and 11B spectra were observed for what was believed to be BF3OH- (Mesmer and Rutenberg, 1973).
The rate constant obtained from the earlier part of the hydrolysis reaction of 0.1 M HBF4 was found to be 0.00090 min-1 (Wamser, 1948). Wamser also stated that a 0.001M solution of fluoroboric acid requires about two months to come to equilibrium and that the salts of fluoboric acid hydrolyze very slowly in the presence of alkali (rapidly only at the boiling point and in the presence of excess calcium ions) (Wamser, 1948).
Anbar and Guttmann demonstrated that the rate of hydrolysis of fluoroborate ions was first order for BF4− and first order for the hydrogen ion concentration in the acid region; hydrolyis rate = k [H+] [BF4−]. There was found no catalytic effect of HF initially present on the rate of hydrolysis. This result is confirmed by the fact that no autocatalytic deviation from first rate law was observed. In another series of experiments bisulfate ions were added up to 0.9 M keeping the pH constant, no appreciable change of the rate of hydrolysis could be detected. The activation energy ΔE = 25.1 kcal./mole-1, was derived from data at 25°, 37°, (1.23 ± 0.05 X 10-3 mol-1L. sec.-1), 60° (k = 1.33 ± 0.05 X 10-2 X 10-3 mol-1L. sec.-1) and at 100° (k = 1.10 X 100mol-1L. sec.-1). The specific rate constant of fluoroborate hydrolysis in acid solution is therefore k = 2.3 x 1014e-25100/RTmol-1L. sec.-1. In neutral and basic solutions the rate of hydrolysis is much lower and no effect of alkalinity on the rate of hydrolysis could be detected from measurements at 60°C and at 100°C. The following rate constant for non-acid hydrolysis of fluoroborate ion was found: k = 8 x 105e-15500/RTsec.-1 (Anbar & Guttmann, 1960)
The specific rate constants of the monomolecular dissociation of BF4− at 25° is slower by a factor of about 1010 from that of HBF4, although its energy of activation is lower by about 9.6 kcal/mole. This points to a spectacular increase in the entropy of activation of the dissociation process on addition of a proton to the BF4− ion (Anbar & Guttmann, 1960).
The result for the recently conducted OECD guideline study 111 (Tier 1) is well in line with literature (TNO Triskelion BV, 2012). The degree of hydrolysis as observed in this study, i.e. between 42 -56 % after 5 days at 50°C is in line with the result observed by Wamser, i.e. 35% (Wamser, 1948).
Also the recent F-NMR investigations (Hildebrand, 2012) confirmed the existing literature, i.e. at a higher concentration tested ( 0,5%, i.e. 0.4 M) the hydrolysis of the BF4- was less pronounced, i.e. ± 5% after 5 days at room temperature and ± 15% after 5 days at 50°C.
Conclusion
The hydrolysis of BF4− occurs stepwise to BF3OH− , BF2(OH)2− , and BF(OH)3− and ultimately B(OH)4− accompanied with the formation of hydrofluoric acid. Due to the strong affinity of boron for fluoride, complete hydrolysis of BF4- occurs at low concentrations, yet with a slow initial step, especially at normal temperature and at a neutral or alkaline pH.
References
- Anbar, M., & Guttmann, S. (1960). The isotopic exchange of fluoroboric acid with hydrofluoric acid. J. Phys. Chem.,64, 1896–1899.
- TNO Triskelion BV (2012). Hydrolysis of the BF4- ion of KBF4 as a function of pH according to OECD guideline 111, a preliminary test (Tier 1) and identification of hydrolysis products. Unpublished report.
- Mesmer, R. E., Palen, K. M., & Baes Jr., C. F. (1973). Fluroborate Equilibria in Aqueous Solutions. Inorganic Chemistry,12(1), 89–95.
- Mesmer, R.E. & Rutenberg, A.C. (1973). Fluorine-19 Nuclear Magnetic Resonance studies on fluoroborate Species in Aqueous Solutions. Inorganic Chemistry, 12(3), 699 -702.
- Papcun, J. R. (2000). Fluorine Compounds, Inorganic, fluoroboric Acid and Fluoroborates. Kirk-Othmer Encyclopedia of Chemical Technology(pp. 1–13). John Wiley & Sons, Inc. doi:10.1002/0471238961.0612211516011603.a01
- Wamser, C. A. (1948). Hydrolysis of fluoboric Acid in Aqueous Solution. J. Am Chem. Soc.,70, 1209–1215.
- Wamser, C. A. (1951). Equilibria in the System Boron Trifluoride-Water at 25 °C. J. Am Chem. Soc.,73, 409–416.
- Hildebrand, M (2012). KBF4 hydrolytical stability in water. Unpublished report
Information on Registered Substances comes from registration dossiers which have been assigned a registration number. The assignment of a registration number does however not guarantee that the information in the dossier is correct or that the dossier is compliant with Regulation (EC) No 1907/2006 (the REACH Regulation). This information has not been reviewed or verified by the Agency or any other authority. The content is subject to change without prior notice.
Reproduction or further distribution of this information may be subject to copyright protection. Use of the information without obtaining the permission from the owner(s) of the respective information might violate the rights of the owner.
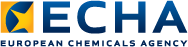