Registration Dossier
Registration Dossier
Data platform availability banner - registered substances factsheets
Please be aware that this old REACH registration data factsheet is no longer maintained; it remains frozen as of 19th May 2023.
The new ECHA CHEM database has been released by ECHA, and it now contains all REACH registration data. There are more details on the transition of ECHA's published data to ECHA CHEM here.
Diss Factsheets
Use of this information is subject to copyright laws and may require the permission of the owner of the information, as described in the ECHA Legal Notice.
EC number: 231-765-0 | CAS number: 7722-84-1
- Life Cycle description
- Uses advised against
- Endpoint summary
- Appearance / physical state / colour
- Melting point / freezing point
- Boiling point
- Density
- Particle size distribution (Granulometry)
- Vapour pressure
- Partition coefficient
- Water solubility
- Solubility in organic solvents / fat solubility
- Surface tension
- Flash point
- Auto flammability
- Flammability
- Explosiveness
- Oxidising properties
- Oxidation reduction potential
- Stability in organic solvents and identity of relevant degradation products
- Storage stability and reactivity towards container material
- Stability: thermal, sunlight, metals
- pH
- Dissociation constant
- Viscosity
- Additional physico-chemical information
- Additional physico-chemical properties of nanomaterials
- Nanomaterial agglomeration / aggregation
- Nanomaterial crystalline phase
- Nanomaterial crystallite and grain size
- Nanomaterial aspect ratio / shape
- Nanomaterial specific surface area
- Nanomaterial Zeta potential
- Nanomaterial surface chemistry
- Nanomaterial dustiness
- Nanomaterial porosity
- Nanomaterial pour density
- Nanomaterial photocatalytic activity
- Nanomaterial radical formation potential
- Nanomaterial catalytic activity
- Endpoint summary
- Stability
- Biodegradation
- Bioaccumulation
- Transport and distribution
- Environmental data
- Additional information on environmental fate and behaviour
- Ecotoxicological Summary
- Aquatic toxicity
- Endpoint summary
- Short-term toxicity to fish
- Long-term toxicity to fish
- Short-term toxicity to aquatic invertebrates
- Long-term toxicity to aquatic invertebrates
- Toxicity to aquatic algae and cyanobacteria
- Toxicity to aquatic plants other than algae
- Toxicity to microorganisms
- Endocrine disrupter testing in aquatic vertebrates – in vivo
- Toxicity to other aquatic organisms
- Sediment toxicity
- Terrestrial toxicity
- Biological effects monitoring
- Biotransformation and kinetics
- Additional ecotoxological information
- Toxicological Summary
- Toxicokinetics, metabolism and distribution
- Acute Toxicity
- Irritation / corrosion
- Sensitisation
- Repeated dose toxicity
- Genetic toxicity
- Carcinogenicity
- Toxicity to reproduction
- Specific investigations
- Exposure related observations in humans
- Toxic effects on livestock and pets
- Additional toxicological data

Endpoint summary
Administrative data
Description of key information
Additional information
A trend towards fast decomposition of hydrogen peroxide occurs in soil and groundwater. Decomposition in soil or groundwater typically takes minutes to several hours, depending on the concentrations of microorganisms present. This is true whether hydrogen peroxide is initially present at relatively low naturally occurring concentrations (Cooper and Zepp 1990, Cooper et al. 1994), or at much higher concentrations (several thousand fold) characteristic of in situ soil and groundwater remediation treatments (Spain et al. 1989, Pardieck et al. 1992). Difficulty has been encountered in maintaining hydrogen peroxide at the desired in situ treatment concentrations (above 100 mg/L) because of its rapid environmental decomposition (Morgan and Watkinson 1992).
A number of laboratory and field studies have reported effects of catalysed hydrogen peroxide on microbial activity in soil and groundwater. Negligible long-term observable adverse effects of oxidant addition on microbial biomass or the effectiveness of bioremediation ability were found (Sahl and Munakata-Marr 2006). This may be explained by the fact that many microbes in times of stress caused by the presence of hydrogen peroxide and other oxidative compounds are able to produce antioxidative enzymes, such as catalase and superoxide dismutase. These enzymes protect the cells against reactive oxygen species. The majority of aerobic and anaerobic microbes present in soil and groundwater are catalase-positive (Pardieck et al. 1992) and thus potentially protected from exposure to hydrogen peroxide and other oxidative compounds formed by its decomposition. The microbial cell numbers declined at hydrogen peroxide concentrations greater than 77 mg/L (Büyuksönmez et al. 1999). An initial decrease in microbial populations following the introduction of hydrogen peroxide was observed after application of hydrogen peroxide in a laboratory study, which was followed by a rebound with cell numbers exceeding the initial microbial biomass after two weeks (Allen and Reardon 2000). A reestablishment of microbial populations was also observed in a field study using hydrogen peroxide to support the aerobic biodegradation of organic contaminants (Kastner et al. 2000). A rebound of microbial activity following exposure to a chemical oxidant may be attributed to different factors including lower concentrations of contaminants after the oxidation (Chapelle et al. 2005), repopulation of oxidized areas by underlying groundwater or new growth of indigenous microbes after reestablishment of pre-oxidation conditions (Klens et al. 2001). The use of a chemical oxidant in soil and groundwater remediation can lead to a decrease in microbial diversity (Macbeth et al. 2005, Miller et al. 1996), which may be due to microbial specialisation. Evidence exists that methanogenic micro-organisms may be most sensitive to chemical oxidation agents (Klens et al. 2001) as the introduction of oxygen into the ground significantly changes the redox-potential and thus may influence the metabolism of anaerobic micro-organisms.
No direct studies are known on the effect of rapid hydrogen peroxide breakdown in soil to the microbial organisms themselves. The literature seems to suggest that when microbial density and biomass are high compared with the concentration and total amount of available hydrogen peroxide, or if oxygen demand is high, there are no adverse effects to microbial populations (Larish and Duff 1997). In the opposite situation, short-term toxicity to microorganisms is evident, but acclimation and rebound of the populations always takes place (Balvay 1981, Spain et al. 1989, Xenopoulos and Bird 1997). No long-term or irreversible damage to a given microbial biomass as the result of such exposure has been recorded.
Higher soil organisms
A prominent representative of soil macro-fauna is the earthworm Eisenia foetida which plays an important role in the decomposition of organic material in the soil. These earthworms seem to be well-equipped with effective antioxidant defences (glutathione, glutathione-related enzymes, catalase, glycoliprotein) that may protect them against potentially harmful effects of hydrogen peroxide exposure (Saint-Denis et al. 1998). Similar defence mechanisms are also found in other earthworms, such as Lampito mauritii (Maity et al. 2008).
References
Allen S.A. and Reardon K.F. 2000. Remediation of contaminated soils by combined chemical and biological treatments. In G.B. Wickamanayke and A.R. Gavaskar (Eds.). Physical and thermal technologies: Remediation of chlorinated and recalcitrant compounds (pp. 301-306). Columbus: Battelle Press.
Balvay G. 1981. Biological consequences of treating a lake with hydrogen peroxide on the plankton biocenosis. Water Research 15: 69 1-696.
Büyuksönmez F., Hess T.F., Crawford R.L., Paszczynski A. and Watts R.J. 1999. Opitmization of simultaneous chemical and biological mineralization of perchloroethylene. Applied and Environmental Microbiology 65: 2784-2788.
Chapelle F.H., Bradley P.M. and Casey C.C. 2005. Behavior of a chlorinated ethene plume following source-area treatment with Fenton's reagent. Ground Water Monitoring and Remediation 25(2): 131-141.
Cooper, WJ and Zepp RG. 1990. Hydrogen peroxide decay in waters with suspended soils: Evidence for biologically mediated processes. Canadian Journal of Fisheries and Aquatic Science 47:888- 893.
Cooper W, Shao C, Lean DRS, Gordon AS and Scully FE. 1994. Factors affecting the distribution of H202 in surface waters. Pages 391 -422 in L.A. Baker, editor. Environmental Chemistry of Lakes and Reservoirs. American Chemical Symposium Series 237, Washington, D.C.
Kastner J.R., Domingo J.S., Denham M., Molina M. and Brigmon R. 2000. Effect of chemical oxidation on subsurface microbiology and trichloroethene biodegradation. Bioremediation Journal 4(3): 219-236.
Klens J., Scarborough S. and Graves D. 2001. The effects of permanganate oxidation on subsurface microbial populations. In A.
Larish, BC and Duff SJB. 1997. Effect of H202 on characteristics and biological treatment of TCF bleached pulp mill effluent. Water Research 3 1:1694-1700.
Leeson, M.E. Kelley, H.S. Rifai and V.S. Magar (Eds.). Natural attenuation of environmental contaminants (Vol. 6, pp. 253-259). Columbus: Battelle Press.
Macbeth T.W., Peterson L.N., Starr R.C., Sorenson K.S., Goehlert R. and Moor K.S. 2005. ISCO impacts on indigenous microbes in a PCE-DNAPL contaminated aquifer. Paper presented at the Eight International In Situ and On-Site Bioremediation Symposium, Baltimore.
Maity S., Roy S., Chaudhury S. and Bhattacharya S. 2008. Antioxidant responses of the earthworm Lampito mauritii exposed to Pb and Zn contaminated soil.
Miller C.M., Valentine R.L., Roehl M.E. and Alvarez P. 1996. Chemical and microbiological assessment of pendimethalin-contaminated soil after treatment with Fenton's reagent. Water Research 30: 2579-2586.
Morgan, P, and Watkinson RJ. 1992. Factors limiting the supply and efficiency and nutrient and oxygen supplements for the in situ biotreatment of contaminated soil and groundwater. Water Research 26:73-78.
Pardieck D.L., Brouwer E.J. and Stone A.T. 1992. Hydrogen peroxide use to increase oxidant capacity for in situ bioremediation of contaminated soils and aquifers: a review. Journal of Contaminant Hydrology 9: 221-242.
Sahl J. and Munakata-Marr J. 2006. The effects of in situ chemical oxidation on microbiological processes: a review. Remediation 16(3): 57 -70.
Saint-Denis M., Labrot F., Narbonne J.F. and Ribera D. 1998. Glutathione, Glutathione-Related Enzymes, and Catalase Activities in the Earthworm Eisenia fetida andrei. Archives of Environmental Contamination and Toxicology 35(4): 602-614.
Spain JC, Milligan JD, Downey DC and Slaughter JK. 1989. Excessive bacterial decomposition of H202,during enhanced biodegradation. Ground Water 27: 163-167.
Xenopoulos. M. A., and D. F. Bird. 1997. Effect of acute exposure to hydrogen peroxide on the production of phytoplankton and bacterioplankton in a mesohumic lake. Photochemistry and Photobiology 66:471-478.
Information on Registered Substances comes from registration dossiers which have been assigned a registration number. The assignment of a registration number does however not guarantee that the information in the dossier is correct or that the dossier is compliant with Regulation (EC) No 1907/2006 (the REACH Regulation). This information has not been reviewed or verified by the Agency or any other authority. The content is subject to change without prior notice.
Reproduction or further distribution of this information may be subject to copyright protection. Use of the information without obtaining the permission from the owner(s) of the respective information might violate the rights of the owner.
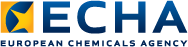