Registration Dossier
Registration Dossier
Data platform availability banner - registered substances factsheets
Please be aware that this old REACH registration data factsheet is no longer maintained; it remains frozen as of 19th May 2023.
The new ECHA CHEM database has been released by ECHA, and it now contains all REACH registration data. There are more details on the transition of ECHA's published data to ECHA CHEM here.
Diss Factsheets
Use of this information is subject to copyright laws and may require the permission of the owner of the information, as described in the ECHA Legal Notice.
EC number: 231-984-1 | CAS number: 7783-20-2
- Life Cycle description
- Uses advised against
- Endpoint summary
- Appearance / physical state / colour
- Melting point / freezing point
- Boiling point
- Density
- Particle size distribution (Granulometry)
- Vapour pressure
- Partition coefficient
- Water solubility
- Solubility in organic solvents / fat solubility
- Surface tension
- Flash point
- Auto flammability
- Flammability
- Explosiveness
- Oxidising properties
- Oxidation reduction potential
- Stability in organic solvents and identity of relevant degradation products
- Storage stability and reactivity towards container material
- Stability: thermal, sunlight, metals
- pH
- Dissociation constant
- Viscosity
- Additional physico-chemical information
- Additional physico-chemical properties of nanomaterials
- Nanomaterial agglomeration / aggregation
- Nanomaterial crystalline phase
- Nanomaterial crystallite and grain size
- Nanomaterial aspect ratio / shape
- Nanomaterial specific surface area
- Nanomaterial Zeta potential
- Nanomaterial surface chemistry
- Nanomaterial dustiness
- Nanomaterial porosity
- Nanomaterial pour density
- Nanomaterial photocatalytic activity
- Nanomaterial radical formation potential
- Nanomaterial catalytic activity
- Endpoint summary
- Stability
- Biodegradation
- Bioaccumulation
- Transport and distribution
- Environmental data
- Additional information on environmental fate and behaviour
- Ecotoxicological Summary
- Aquatic toxicity
- Endpoint summary
- Short-term toxicity to fish
- Long-term toxicity to fish
- Short-term toxicity to aquatic invertebrates
- Long-term toxicity to aquatic invertebrates
- Toxicity to aquatic algae and cyanobacteria
- Toxicity to aquatic plants other than algae
- Toxicity to microorganisms
- Endocrine disrupter testing in aquatic vertebrates – in vivo
- Toxicity to other aquatic organisms
- Sediment toxicity
- Terrestrial toxicity
- Biological effects monitoring
- Biotransformation and kinetics
- Additional ecotoxological information
- Toxicological Summary
- Toxicokinetics, metabolism and distribution
- Acute Toxicity
- Irritation / corrosion
- Sensitisation
- Repeated dose toxicity
- Genetic toxicity
- Carcinogenicity
- Toxicity to reproduction
- Specific investigations
- Exposure related observations in humans
- Toxic effects on livestock and pets
- Additional toxicological data

Acute Toxicity: inhalation
Administrative data
- Endpoint:
- acute toxicity: inhalation
- Type of information:
- experimental study
- Adequacy of study:
- key study
- Study period:
- no data
- Reliability:
- 2 (reliable with restrictions)
- Rationale for reliability incl. deficiencies:
- guideline study without detailed documentation
Data source
Reference
- Reference Type:
- publication
- Title:
- Effects of sulfate aerosols in combination with ozone on elimination of tracer particles inhaled by rats.
- Author:
- Phalen RF et al.
- Year:
- 1 980
- Bibliographic source:
- J Toxicol Environ Health 6: 797-810.
Materials and methods
Test guideline
- Qualifier:
- equivalent or similar to guideline
- Guideline:
- OECD Guideline 433 draft (Acute Inhalation Toxicity: Fixed Concentration Procedure) (not officially approved)
- GLP compliance:
- not specified
- Test type:
- other: effect on respiratory defense system
- Limit test:
- yes
Test material
- Reference substance name:
- Ammonium sulphate
- EC Number:
- 231-984-1
- EC Name:
- Ammonium sulphate
- Cas Number:
- 7783-20-2
- Molecular formula:
- H3N.1/2H2O4S
- IUPAC Name:
- diammonium sulfate
- Test material form:
- solid: crystalline
Constituent 1
Test animals
- Species:
- rat
- Strain:
- Sprague-Dawley
- Sex:
- male
- Details on test animals or test system and environmental conditions:
- TEST ANIMALS
male SPF Sprague-Dawley rats
- Source: Hilltop Lab Animals, Inc. (Chatsworth, Calif.)
- Weight at study initiation: ca. 200 g
No further data
ENVIRONMENTAL CONDITIONS
Both temperature and humidity were controlled in this study. Air was supplied to the chambers at about 0.1-0.3 m³/min.
Administration / exposure
- Route of administration:
- inhalation: aerosol
- Type of inhalation exposure:
- nose only
- Vehicle:
- other: water
- Details on inhalation exposure:
- Rats were exposed by inhalation to radioactive tracer particles and then randomly divided into experimental and control groups. One hour after a 20-min nose-only exposure to tracer particles, animals were placed in individual compartments in open-mesh stainless steel exposure cages. Cages were placed on one level only of a 1 m³ stainless steel chamber for a 4-h exposure to either purified or intentionally polluted air.
The tracer particles, tagged with tightly bound 51Cr, were monodisperse polystyrene latex (PSL) spheres having geometric diameters near 1.4 µm. Aerosols were produced from an aqueous suspension of 0.1% solids (by volume), using a Lovelace-type laboratory compressed-air nebulizer. The MMAD of the aerosol particles, as determined with a multistage laboratory impactor was about 1.6 µm.
Tracer particles were aerosolized, dried, brought to charge equilibrium, and passed into a nose-only exposure chamber. The individual tubes for holding rats in the device were made of perforated metal and were thin-walled to reduce thermal stress due to body heat.
The average amount of tracer material deposited per rat was less than 0.1 µCi, which is contained in less than 1 µg of particles. After the deposition of tracer particles was completed, the rats' noses were washed with water to remove radioactive particles. The animals were then placed in individual plastic counting tubes and inserted in a collimated counting apparatus. All rats that underwent deposition of PSL particles were counted twice for 100 s in this apparatus before they were placed in the pollutant exposure chambers. When the 4-h clean air or pollutant exposure was completed, the animals were periodically put into individual plastic counting tubes and the amount of radioactivity in the respiratory tract was determined at five additional predetermined times for up to 17 d. Fecal samples were collected from each rat 11 times during the first 48 h after tracer particle deposition. Coprophagy was minimized by the use of 1/2-inch mesh wire cage bottoms and by the frequent fecal collections.
Clearance curves were determined for each animal and half times obtained from least-squares fits for short- and long-term clearance data. Group mean values for pollutant-exposed and sham-exposed (control) groups were calculated. Half-times for experimental groups were subtracted from those for control groups and the differences tested for significance at the 90% level, using a two-tailed t-test.
Purified air supplied to the exposure chambers had been passed successively through a coarse particulate filter, a humidifier, a heater, and a high-efficiency particulate (HEPA) filter. Both temperature and humidity were controlled in this system. Air was supplied to the chambers at about 0.1-0.3 m³/min. Ammonia levels, due to the presence of rats, were measured as about 0.25 ppm or less under these conditions of exposure.
Stable, controllable salt aerosols with MMAD between 0.4 and 0.6 µm and sulfuric acid aerosols with MMAD of 1.0 µm, at mass concentrations up to 3 or 4 mg/m³ in air, were generated with compressed-air nebulizers loaded with aqueous sulfate solutions. A Collison-type three-jet nebulizer followed by a 85 Kr charge neutralizer and air-dilution drier, was used for ammonium sulfate and ferric sulfate particle generation. Under low humidity (30-40%) chamber conditions these aerosols were dry and were sized by electron microscopy. Ferric sulfate and ammonium sulfate aerosols were collected on electron microscope grids, using an electrostatic precipitator. The size distribution, count median diameter, mass median diameter, and geometric standard deviation were then determined by analysis of photographs. At high humidity (greater than 80%) the aerosols were wet and the multistage laboratory impactor was used.
Sulfuric acid aerosols were generated from solution by an all-glass compressed-air nebulizer. Sizing was performed by determining the titratable acidity.
Airborne mass concentrations were determined by putting two fiberglass filters in series inside the chamber and sampling at constant flow rates for up to 1 h. The first filter captured the aerosol and the second filter gave the change of filter weight due to humidity and allowed the efficiency of the primary sample filter to be verified.
Ozone, produced by passing medical grade oxygen through an electrical ozone generator, was introduced into a chamber run at constant flow rate and slight negative pressure. A Dasibi ultraviolet monitor was used to determine the ozone levels.
All samples for aerosol and gas characterization were acquired from the center of the breathing zone of the animals. Sampling lines were large-bore stainless steel for aerosol with Teflon for ozone. - Analytical verification of test atmosphere concentrations:
- yes
- Duration of exposure:
- 4 h
- Remarks on duration:
- relative humidity: 39% (low-humidity exposure); 85% (high-humidity exposure)
- Concentrations:
- 3.5 mg/m³; MMAD 0.4 µm
- No. of animals per sex per dose:
- 10-12
- Control animals:
- yes
- Statistics:
- two-tailed t-test
Results and discussion
Effect levels
- Key result
- Sex:
- male
- Dose descriptor:
- LC0
- Effect level:
- 3.5 mg/m³ air
- Exp. duration:
- 4 h
Any other information on results incl. tables
Atmospheres
Concentrations and other characteristics of the atmospheres were remarkably stable from one exposure to another. Average data for all runs with standard deviations were: ozone, 0.79 ± 0.02 ppm; aerosol concentrations, 3.6 ± 0.4 mg/m³; low relative humidity, 39 ± 3%; high humidity, 85 ± 4%; MMAD of salt aerosols, 0.4 ± 0.1 µm ; and MMAD of sulfuric acid aerosols, 1.0 ± 0.2 µm. The aerosols had average estimated geometric standard deviations of 1.9 -2.3 from impactor data. Electron microscopy indicated geometric standard deviations of 1.6 -1.7.
Clearance Measurements
The low relative humidity sham-exposed animals were selected as primary controls to examine the effect of high relative humidity as a potential cotoxin. A total of 12 groups of 10-15 rats each were exposed to low-humidity clean air. The clearance data for these animals are given in Table 1 (see attached file). Some animals were excluded from the data analysis because they did not consume food or water at a sufficient rate to remove tracer particles from the gastrointestinal tract. When this occurred, in about 5% of the rats, clearance half-time values could not be obtained.
The effect of high humidity on clearance was interesting The short-term clearance half-time was longer in the high-humidity group by 0.9 h ; the long-term clearance half-time was diminished by high relative humidity by about 90 h (significant at p= 0.1).
As shown in Table 2 (see attached file), ozone alone at 0.8 ppm and low humidity statistically significantly slowed early clearance and accelerated late clearance. These effects were even greater at high humidity, the effects of humidity being roughly additive to those of ozone.
Ammonium sulfate at high or low humidity did not have any significant effects on early or late clearance compared to that in low-humidity clean-air controls.
The clearance data for aerosols combined with ozone are very similar to those for ozone alone. In no case is there a statistically significant difference between ozone alone and ozone with an aerosol at the same humidity. Further, in the majority of cases clearance patterns with sulfate particles and ozone both present lie between those for sham-exposed groups and groups exposed to ozone only. The atmosphere with the greatest effect on short-term clearance was sulfuric acid mist with ozone at high humidity.
Table 1: Clearance data for rats exposed to low-and high humidity clean air
number of animals | clearance half-time (h) a |
|||
humidity | short-term | long-term | short-term | long-term |
low | 124 | 123 | 11.17± 2.29 | 465 ± 328 |
high | 98 | 96 | 12.06± 2.31 | 376 ± 250 |
0.89± 0.33 | -89 ± 42 |
a mean ± SD
Table 2. Particle Clearance Responses of Rats Exposed for 4 h to Ozone and Sulfate Aerosols (3.5 mg/m3): Group Mean Differences from Low-Humidity Sham-exposed Animals
|
Change in clearance half-time (h)a |
|
Treatment |
Short-term |
Long-term |
|
Low relative humidity |
|
Sham |
0 ± 0.3 (124)b |
0 ± 42 (123) |
0.8 ppm O3 |
0.9 ± 0.5c(39) |
-152 ± 66c(40) |
(NH4)2SO4d |
0.1 ± 0.6 (29) |
-14 ± 38 (29) |
Fe2(SO4)3d |
-0.3 ± 0.7 (28) |
113 ± 53c(30) |
H2SO4d |
0.5 ± 0.7 (30) |
79 ± 37c(30) |
(NH4)2SO4+ 0.8 ppm O3 |
1.5 ± 0.6c(33) |
-104 ± 53c(33) |
Fe2(SO4)3+ 0.8 ppm O3 |
1.0 ± 0.6 (30) |
-107 ± 95 (30) |
H2SO4 ±0.8 ppm O3 |
0.7 ± 0.5 (40) |
-208 ± 79c(40) |
|
|
|
|
High relative humidity |
|
Sham |
0.9 ± 0.3c(98) |
-89 ± 42c(96) |
0.8 ppm O3 |
1.7 ± 0.8c(38) |
-174 ± 85c(40) |
(NH4)2SO4 |
0.6 ± 0.8 (29) |
38 ± 80 (30) |
Fe2(SO4)3 |
0.4 ± 0.8 (29) |
-78 ± 79 (30) |
H2SO4 |
1.3 ± 0.8 (29) |
-69 ± 79 (30) |
(NH4)2SO4+ 0.8 ppm O3 |
0.9 ± 0.7 (39) |
-153 ± 107 (30) |
Fe2(SO4)3+ 0.8 ppm O3 |
0.8 ± 0.9 (29) |
-133 ± 92 (29) |
H2SO4 ±0.8 ppm O3 |
2.6 ± 0.8c(40) |
-158 ± 99 (27) |
a Mean ± SE. Positive values represent slowing and negative values acceleration of clearance.
b Number of rats in each group is shown in parentheses.
c Significantly different from low-humidity sham-exposed animals (p<0.1, two-tailedt-test).
d Form inhaled is not usually the pure undissociated sulfate.
Applicant's summary and conclusion
Information on Registered Substances comes from registration dossiers which have been assigned a registration number. The assignment of a registration number does however not guarantee that the information in the dossier is correct or that the dossier is compliant with Regulation (EC) No 1907/2006 (the REACH Regulation). This information has not been reviewed or verified by the Agency or any other authority. The content is subject to change without prior notice.
Reproduction or further distribution of this information may be subject to copyright protection. Use of the information without obtaining the permission from the owner(s) of the respective information might violate the rights of the owner.
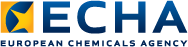