Registration Dossier
Registration Dossier
Data platform availability banner - registered substances factsheets
Please be aware that this old REACH registration data factsheet is no longer maintained; it remains frozen as of 19th May 2023.
The new ECHA CHEM database has been released by ECHA, and it now contains all REACH registration data. There are more details on the transition of ECHA's published data to ECHA CHEM here.
Diss Factsheets
Use of this information is subject to copyright laws and may require the permission of the owner of the information, as described in the ECHA Legal Notice.
EC number: 234-190-3 | CAS number: 10588-01-9
- Life Cycle description
- Uses advised against
- Endpoint summary
- Appearance / physical state / colour
- Melting point / freezing point
- Boiling point
- Density
- Particle size distribution (Granulometry)
- Vapour pressure
- Partition coefficient
- Water solubility
- Solubility in organic solvents / fat solubility
- Surface tension
- Flash point
- Auto flammability
- Flammability
- Explosiveness
- Oxidising properties
- Oxidation reduction potential
- Stability in organic solvents and identity of relevant degradation products
- Storage stability and reactivity towards container material
- Stability: thermal, sunlight, metals
- pH
- Dissociation constant
- Viscosity
- Additional physico-chemical information
- Additional physico-chemical properties of nanomaterials
- Nanomaterial agglomeration / aggregation
- Nanomaterial crystalline phase
- Nanomaterial crystallite and grain size
- Nanomaterial aspect ratio / shape
- Nanomaterial specific surface area
- Nanomaterial Zeta potential
- Nanomaterial surface chemistry
- Nanomaterial dustiness
- Nanomaterial porosity
- Nanomaterial pour density
- Nanomaterial photocatalytic activity
- Nanomaterial radical formation potential
- Nanomaterial catalytic activity
- Endpoint summary
- Stability
- Biodegradation
- Bioaccumulation
- Transport and distribution
- Environmental data
- Additional information on environmental fate and behaviour
- Ecotoxicological Summary
- Aquatic toxicity
- Endpoint summary
- Short-term toxicity to fish
- Long-term toxicity to fish
- Short-term toxicity to aquatic invertebrates
- Long-term toxicity to aquatic invertebrates
- Toxicity to aquatic algae and cyanobacteria
- Toxicity to aquatic plants other than algae
- Toxicity to microorganisms
- Endocrine disrupter testing in aquatic vertebrates – in vivo
- Toxicity to other aquatic organisms
- Sediment toxicity
- Terrestrial toxicity
- Biological effects monitoring
- Biotransformation and kinetics
- Additional ecotoxological information
- Toxicological Summary
- Toxicokinetics, metabolism and distribution
- Acute Toxicity
- Irritation / corrosion
- Sensitisation
- Repeated dose toxicity
- Genetic toxicity
- Carcinogenicity
- Toxicity to reproduction
- Specific investigations
- Exposure related observations in humans
- Toxic effects on livestock and pets
- Additional toxicological data

Endpoint summary
Administrative data
Link to relevant study record(s)
Description of key information
Available human and animal data for the absorption, distribution, metabolism and excretion of chromium (VI) substances are summarised in the publicly available ATSDR toxicological profile for chromium published in 2012.
Key value for chemical safety assessment
Additional information
Unless otherwise stated, the information contained in this section was taken from the ATSDR Toxicological profile for chromium (2012).
1.Absorption
1.1 Inhalation exposure
The absorption of inhaled chromium compounds depends on a number of factors, including physical and chemical properties of the particles (oxidation state, size, solubility) and the activity of alveolar macrophages.
The identification of chromium in urine, serum and tissues of humans occupationally exposed to soluble chromium(III) or chromium(VI) compounds in air indicates that chromium can be absorbed from the lungs (Cavalleri and Minoia 1985; Gylseth et al. 1977; Kiilunen et al. 1983; Mancuso 1997b; Minoia and Cavalleri 1988; Randall and Gibson 1987; Tossavainen et al. 1980). In most cases, chromium(VI) compounds are more readily absorbed from the lungs than chromium(III) compounds, due in part to differences in the capacity to penetrate biological membranes.
Rats exposed to 2.1 mg chromium(VI)/m3 as zinc chromate 6 hours/day achieved steady-state concentrations in the blood after ~4 days of exposure (Langård et al. 1978). Rats exposed for a single inhalation of chromium(VI) trioxide mist from electroplating at a concentration of 3.18 mg chromium(VI)/m3 for 30 minutes rapidly absorbed chromium from the lungs. The content of chromium in the lungs declined from 13.0 mg immediately after exposure to 1.1 mg at 4 weeks in a triphasic pattern with an overall half-life of 5 days (Adachi et al. 1981). Based on a study in rats exposed to chromium(VI) as potassium dichromate or to chromium(III) as chromium trichloride, the pulmonary clearance of both valence states was dependent on particle size, and chromium(VI) was more rapidly and extensively transported to the bloodstream than chromium(III). The rats had been exposed to 7.3–15.9 mg chromium(VI)/m3 as potassium dichromate for 2–6 hours or to 8 or 10.7 mg chromium(III)/m3 as chromium trichloride for 6 or 2 hours, respectively. Chromium(VI) particles of 1.5 or 1.6 μm had a two-compartment pulmonary clearance curve with half-lives of 31.5 hours for the first phase and 737 hours for the second phase. Chromium(VI) particles of 2 μm had a single component curve with a half-life between 151 and 175 hours. Following exposure to chromium(VI), the ratio of blood chromium/lung chromium was 1.44 at 0.5 hours, 0.81 at 18 hours, 0.85 at 48 hours, and 0.96 at 168 hours after exposure.
Chromium(III) particles of 1.5–1.8μm had a single component pulmonary clearance curve with a half-life of 164 hours. Following exposure to chromium(III), the ratio of blood chromium/lung chromium was 0.39 at 0.5 hours, 0.24 at 18 hours, 0.22 at 48 hours, and 0.26 at 168 hours after exposure.Therefore, the amount of chromium(VI) transferred to the blood from the lungs was always at least 3 times greater than the amount of chromium(III) transferred (Suzuki et al. 1984). Other studies reporting absorption from the lungs are intratracheal injection studies (Baetjer et al. 1959b; Bragt and van Dura 1983; Visek et al. 1953; Wiegand et al. 1984, 1987).These studies indicate that 53–85% of chromium(VI) compounds (particle size <5μm) are cleared from the lungs by absorption into the bloodstream or by mucociliary clearance in the pharynx; the rest remain in the lungs. Absorption by the bloodstream and mucociliary clearance was only 5–30% for chromium(III) compounds.
The kinetics of three chromium(VI) compounds, sodium chromate, zinc chromate, and lead chromate, were compared in rats in relation to their solubility. The rats received intratracheal injections of the51chromium-labeled compounds (0.38 mg chromium(VI)/kg as sodium chromate, 0.36 mg chromium(VI)/ kg as zinc chromate, or 0.21 mg chromium(VI)/kg as lead chromate). Peak blood levels of51chromium were reached after 30 minutes for sodium chromate (0.35μg chromium/mL), and 24 hours for zinc chromate (0.60μg chromium/mL) and lead chromate (0.007μg chromium/mL). At 30 minutes after administration, the lungs contained 36, 25, and 81% of the respective dose of the sodium, zinc, and lead chromate. On day 6, >80% of the dose of all three compounds had been cleared from the lungs, during which time, the disappearance from lungs followed linear first-order kinetics. The residual amounts left in the lungs on day 50 or 51 were 3.0, 3.9, and 13.9%, respectively. The results indicate that zinc chromate, which is ~1,000 times less soluble than sodium chromate, is more slowly absorbed from the lungs, but peak blood levels are higher than sodium chromate. Lead chromate was more poorly and slowly absorbed, as indicated by very low levels in blood and other tissues, and greater retention in the lungs (Bragt and van Dura 1983).
The fate of lead chromate(VI), chromium(VI) trioxide, chromium(III) oxide and chromium(III) sulfate were examined when solutions or suspensions of these chemicals were slowly infused into the tracheal lobe bronchus of sheep via bronchoscopic catheterization (Perrault et al. 1995). At 2, 3, 5, and 30 days, the samples of bronchoalveolar lavage were taken, and on day 31, the animals were sacrificed and lung specimens were examined for chromium particulates. There was no difference in lung particle concentrations among the four different compounds. The values ranged from 0.14x105 to 1.02x105 particles/g dry tissue compared to control values of 0.03x105. The alveolar clearance of slightly soluble chromium(III) oxide and chromium(III) sulfate was calculated to be 11 and 80 days, respectively. The insoluble lead chromate particles appeared to break up, forming isometric particles of lead chromate as well as lead-containing particulates that may have retarded clearance. Retention of chromium particulates from exposure to soluble chromium trioxide may have resulted in the formation of a less-soluble hydroxyl complex and/or chemical interaction between chromium and protein that prolongs the retention of the metal. Analyses of the particulates in lavage samples indicate that these diameters increase with time for lead chromate, decrease with time for chromium sulfate and chromium trioxide, and are unchanged for chromium(III) oxide. The authors state that their findings indicate that slightly soluble chromium(III) oxide and chromium sulfate that are chemically stable can be cleared from lungs at different rates, depending on the nature and morphology of the compound.
Amounts of total chromium were measured in lymphocytes, blood, and urine after intratracheal administration of either sodium dichromate(VI) or chromium(III) acetate hydroxide (a water-soluble chromium(III) compound) to male Wistar rats (Gao et al. 1993). The total amount of chromium administered was 0.44 mg chromium/kg body weight for each compound. The highest concentrations in tissues and urine occurred at 6 hours after treatment, the first time point examined. Mean chromium concentrations (n=4 rats per time point) from treatment with chromium(III) were 56.3μg/L in whole blood, 96μg/L in plasma, 0.44μg/1010in lymphocytes, and 4,535.6μg/g creatinine in urine. For treatment with chromium(VI) the levels were 233.2μg/L for whole blood, 138μg/L for plasma, 2.87μg/1010for lymphocytes, and 2,947.9μg/g creatinine in urine. The levels in lymphocytes in the chromium(III) treated animals were no different than in untreated animals. However, for chromium(VI) the lymphocyte levels were about 6-fold higher than control values. After 72 hours, the chromium levels were significantly reduced. These results suggest that absorbed chromium(III) compounds may be excreted more rapidly than absorbed chromium(VI) compounds because of a poorer ability to enter cells.
1.2 Oral exposure
The absorption fraction of soluble chromium(VI) is higher than that of soluble chromium(III) (Anderson et al. 1983; Donaldson and Barreras 1966; Kerger et al. 1996a). Average absorption fractions, determined from cumulative urinary excretion in 8 healthy adults who ingested 5 mg chromium (in 10 mg Cr/L drinking water) as CrCl3 or K2Cr2O7 were 0.13% (±0.04, standard error [SE]) and 6.9% (±3.7, SE), respectively. Chromium(VI) can be reduced to chromium(III) when placed in an ascorbic acid solution (Kerger et al. 1996a). When K2Cr2O7 was ingested in orange juice (where it was reduced and may have formed complexes with constituents of the juice), the mean absorption fraction was 0.60% (±0.11, SE; Kerger et al. 1996a). Plasma concentrations generally peaked around 90 minutes following exposure for all three chromium mixtures tested. Based on measurements of urinary excretion of chromium in 15 female and 27 male subjects who ingested 200 μg chromium(III) as CrCl3, the absorption fraction was estimated to be approximately 0.4% (Anderson et al. 1983). The absorption fraction of chromium(VI) (as sodium chromate) was substantially higher when administered directly into the duodenum (approximately 10%) compared to when it is ingested (approximately 1.2%), whereas the absorption fraction for CrCl3 was similar when administered into the small intestine (0.5%; Donaldson and Barreras 1966). These results are consistent with studies that have shown that gastric juice can reduce chromium(VI) to chromium(III) (De Flora et al. 1987a).
The absorption of chromium(VI) and chromium(III) was measured in four male and two female volunteers (ages ranging from 25 to 39 years) treated orally with potassium chromate (chromium(VI)) or chromic oxide (chromium(III)) in capsules at doses of 0.005 and 1.0 mg/kg/day, respectively (Finley et al. 1996b). Subjects were exposed to each compound for 3 days. Based on urinary excretion data, the mean absorption of potassium chromate was 3.4% (range 0.69–11.9%). No statistically significant increase in urinary chromium was observed during chromic oxide dosing, indicating that little, if any, was absorbed.In a follow-up study by the same group (Finley et al. 1997), five male volunteers ingested a liter, in three volumes of 333 mL, of deionized water containing chromium(VI) concentrations ranging from 0.1 to 10.0 mg/L (approximately 0.001–0.1 mg chromium(VI)/kg/day) for 3 days.A dose-related increase in urinary chromium was seen in all subjects and the percent of the dose excreted ranged from <2 to 8%. Dose-related increases in plasma and erythrocyte chromium levels were also observed.
In a repeated dose study, three healthy adults ingested chromium(VI) (as K2Cr2O7) in water at 5 mg chromium/day for 3 consecutive days (Kerger et al. 1997). Three divided doses were taken at approximately 6-hour intervals over a 5–15-minute period. After at least 2 days without dosing, the 3-day exposure regimen was repeated at 10 mg chromium/day. Estimated doses based on body weight were 0.05 and 0.1 mg/kg/day, respectively. Bioavailability based on 4-day urinary excretion was 1.7% (range 0.5–2.7%) at 0.05 mg chromium(VI)/kg/day and 3.4% (range 0.8–8.0%) at 0.1 mg chromium(VI)/kg/day. Absorption of 0.05 mg chromium(VI)/kg appeared to be somewhat lower when given as three divided doses rather than when given as a single bolus dose (1.7 versus 5.7%).
Uptake of potassium dichromate was determined in a man who was given 0.8 mg of chromium(VI) in drinking water 5 times each day for 17 days (Paustenbach et al. 1996). Steady-state concentrations of chromium in blood were attained after 7 days. Red blood cell and plasma levels returned to background levels within a few days after exposure was stopped. The data are consistent with a bioavailability of 2% and a plasma elimination half-life of 36 hours.
Studies with 51chromium in animals indicate that chromium and its compounds are also poorly absorbed from the gastrointestinal tract after oral exposure. When radioactive sodium chromate (chromium(VI)) was given orally to rats, the amount of chromium in the feces was greater than that found when sodium chromate was injected directly into the jejunum. Since chromium(III) is absorbed less readily than chromium(VI) by the gastrointestinal tract, these results are consistent with evidence that the gastric environment has a capacity to reduce chromium(VI) to chromium(III). Ex vivo studies in rats and mice demonstrate that the reduction of chromium(VI) to chromium(III) in the stomach follows second-order kinetics and that the reduction rate is concentration-dependent and capacity-limited (Proctor et al. 2012). The investigators estimated second-rate constants of 0.3 L/mg·hour for rats and 0.2 L/mg·hour for mice and total reducing capacities of 15.7 and 16.6 mg chromium(VI) equivalents/L of stomach contents, respectively.
The administration of radioactive chromium(III) or chromium(VI) compounds directly into the jejunum decreased the amount of chromium recovery in the feces indicating that the jejunum is the absorption site for chromium (Donaldson and Barreras 1966). Absorption of either valence state was ≤1.4% of theadministered oral dose in rats (Sayato et al. 1980) and hamsters (Henderson et al. 1979). Based on distribution and excretion studies in rats administered chromium by gavage for 2–14 days from various sources, that is, from sodium chromate (chromium(VI)), from calcium chromate (chromium(VI)), or from soil contaminated with chromium (30% chromium(VI) and 70% chromium(III)), the low gastrointestinal absorption of chromium from any source was confirmed. Chromium appeared to be better absorbed from the soil than from chromate salts, but <50% of the administered chromium could be accounted for in these studies, partly because not all tissues were examined for chromium content and excretion was not followed to completion (Witmer et al. 1989, 1991). Adult and immature rats given chromium(III) chloride absorbed 0.1 and 1.2% of the oral dose, respectively (Sullivan et al. 1984). This suggests that immature rats may be more susceptible to potential toxic effects of chromium(III) compounds.
Treatment of rats by gavage with a nonencapsulated lead chromate pigment or with a silica-encapsulated lead chromate pigment resulted in no measurable blood levels of chromium (detection limit=10μg/L) after 2 or 4 weeks of treatment or after a 2-week recovery period. However, kidney levels of chromium were significantly higher in the rats that received the nonencapsulated pigment than in the rats that received the encapsulated pigment, indicating that silica encapsulation reduces the gastrointestinal bioavailability of chromium from lead chromate pigments (Clapp et al. 1991).
The issue of whether or not chromium(VI) absorption occurs only when or principally when the reducing capacity of the gastrointestinal tract is exhausted is a factor to consider in evaluating and interpreting oral dosing bioassays in animals and human epidemiology studies of health outcomes related to ingestion exposures to chromium. Potentially, tumor responses could be enhanced if the reducing capacities of saliva and stomach fluid were exhausted. This is more likely to occur at the relatively high doses of chromium(VI) administered in animal bioassays than at doses experienced by humans from environmental exposures. However, results of experimental studies of chromium absorption in humans have not found evidence for an effect of limited reducing capacity on absorption of chromium. The range of doses of chromium administered to humans in these different studies was considerable and demonstrated oral bioavailability at all doses. Donaldson and Barreras (1996) administered 20 ng of radiolabeled chromium(VI), Kerger et al. (1996a) administered 5 mg of chromium(VI), Finley et al. (1996b) administered 0.005 mg/kg/day of chromium(VI) for 3 days, and Finley et al. (1997) administered 0.1, 0.5, 1.0, 5.0 or 10 mg/day of chromium(VI) for 4 days. In the Finley et al. (1997) study, the percent of the administered dose of chromium(VI) recovered in the urine did not increase with dose. The results of these studies do not indicate that oral absorption of administered chromium(VI) only begins to occur when the reducing capacity of the stomach is exhausted, and are consistent with estimates of gastrointestinal reducing capacity (De Flora 2000; Proctor et al. 2002).
1.3 Dermal absorption
Both chromium(III) and chromium(VI) can penetrate human skin to some extent, especially if the skin is damaged. Systemic toxicity has been observed in humans following dermal exposure to chromium compounds, indicating significant cutaneous absorption. Fourteen days after a salve containing potassium chromate was applied to the skin of an individual to treat scabies, appreciable amounts of chromium were found in the blood, urine, feces, and stomach contents (Brieger 1920). It should be noted that the preexisting condition of scabies or the necrosis caused by the potassium chromate could have facilitated dermal absorption of potassium chromate. Potassium dichromate (chromium(VI)), but not chromium(III) sulfate, penetrated the excised intact epidermis of humans (Mali et al. 1963). Chromium(III) from a concentrated chromium sulfate solution at pH 3 penetrated cadaverous human skin at a rate of 5x10-11 cm/sec, compared with a rate for chromium(VI) (source unspecified) of 5x10-7 cm/second (Spruit and van Neer 1966). In contrast, both chromium(VI) from sodium chromate and chromium(III) from chromium trichloride penetrated excised human mammary skin at similar rates, but the rate was generally slightly faster for chromium(VI). Absolute rates of absorption in nmol chromium/hour/cm2 increased with increasing concentration of both chromium(VI) and chromium(III) (Wahlberg 1970). The average rate of systemic uptake of chromium in four volunteers submersed up to the shoulders in a tub of chlorinated water containing a 22 mg chromium(VI)/L solution of potassium dichromate for 3 hours was measured to be 1.5x10-4 μg/cm2-hour based on urinary excretion of total chromium (Corbett et al. 1997).
The influence of solvent on the cutaneous penetration of potassium dichromate by humans has been studied. The test solutions of potassium dichromate in petrolatum or in water were applied as occluded circular patches of filter paper to the skin. Results with dichromate in water revealed that chromium(VI) penetrated beyond the dermis and penetration reached steady state with resorption by the lymph and blood vessels by 5 hours. About 10 times more chromium penetrated when potassium dichromate was applied in petrolatum than when applied in water. About 5 times more chromium penetrated when potassium dichromate was applied than when a chromium trichloride glycine complex was applied (Liden and Lundberg 1979). The rates of absorption of solutions of sodium chromate from the occluded forearm skin of volunteers increased with increasing concentration. The rates were 1.1 μg chromium(VI)/ cm2/hour for a 0.01 M solution, 6.4 μg chromium(VI)/cm2/hour for a 0.1 M solution, and 10 μg chromium(VI)/cm2/hour for a 0.2 M solution (Baranowska-Dutkiewicz 1981).
Chromium and its compounds are also absorbed dermally by animals. The dermal absorption of sodium chromate (chromium(VI)) by guinea pigs was somewhat higher than that of chromium(III) trichloride, but the difference was not significant. At higher concentrations (0.261–0.398 M), absorption of sodium chromate was statistically higher than that of chromium trichloride. The peak rates of absorption were 690–725 and 315–330 nmol/hour/cm2 for sodium chromate at 0.261–0.398 M and chromium trichloride at 0.239–0.261 M, respectively. Percutaneous absorption of sodium chromate was higher at pH≥6.5 compared with pH≤5.6 (Wahlberg and Skog 1965).
2. Distribution
2.1 Inhalation exposure
Examination of tissues from Japanese chrome platers and chromate refining workers at autopsy revealed higher chromium levels in the hilar lymph node, lung, spleen, liver, kidney, and heart, compared to normal healthy males (Teraoka 1981). Analysis of the chromium concentrations in organs and tissues at autopsy of a man who died of lung cancer 10 years after his retirement from working in a chromate producing plant for 30 years revealed measurable levels in the brain, pharyngeal wall, lung, liver, aorta, kidney, abdominal rectal muscle, suprarenal gland, sternal bone marrow, and abdominal skin. The levels were significantly higher than in five controls with no occupational exposure to chromium. The man had been exposed mainly to chromium(VI), with lesser exposure to chromium(III) as the chromite ore (Hyodo et al. 1980). The levels of chromium were higher in the lungs, but not in the liver or kidneys, of autopsy specimens from 21 smeltery and refinery workers in North Sweden compared with that for a control group of 8 individuals. The amount of enrichment in the lungs decreased as the number of elapsed years between retirement and death increased (Brune et al. 1980). Tissues from three individuals having lung cancer who were industrially exposed to chromium were examined by Mancuso (1997b). One was employed for 15 years as a welder, a second worked for 10.2 years, and a third for 31.8 years in ore milling and preparations and boiler operations. The three cumulative chromium exposures for the three workers were 3.45, 4.59, and 11.38 mg/m3 years, respectively. Tissues from the first worker were analyzed 3.5 years after last exposure, the second worker 18 years after, and the third worker 0.6 years after last exposure. All tissues from the three workers had elevated levels of chromium with the possible exception of neural tissues. Levels were orders of magnitude higher in lungs than other tissues. The highest lung level reported was 456 mg/10 g tissue in the first worker, 178 in the second worker, and 1,920 for the third worker. There were significant chromium levels in the tissue of the second worker even though he had not been exposed to chromium for 18 years. Chromium concentrations in lung tissues from autopsy samples were 5 times higher in subjects who originated from the Ruhr and Dortmund regions of Germany, where emissions of chromium are high, than in subjects from Munster and vicinity. The lung concentrations of chromium increased with increasing age. Men had twice as high concentrations of chromium in the lungs than did women, which may reflect the greater potential for occupational exposure by men, the higher vital capacity of men, and possibly a greater history of smoking (Kollmeier et al. 1990).
Chromium may be transferred to fetuses through the placenta and to infants via breast milk. Analysis of chromium levels in women employees of a dichromate manufacturing facility in Russia during and after pregnancy revealed that the exposed women had significantly higher levels of chromium in blood and urine during pregnancy, in umbilical cord blood, placentae, and breast milk at child birth, and in fetuses aborted at 12 weeks than did nonexposed controls (Shmitova 1980). The reliability of this study is suspect because the levels of chromium reported in the blood and urine of the control women were much higher than usual background levels of chromium in these biological fluids, perhaps due to problems with analytical methods. Measurement of the chromium content in 255 samples from 45 lactating American women revealed that most samples contained <0.4 μg/L, and the mean value was
0.3 μg/L (Casey and Hambidge 1984). While these probably represent background levels in women whose main exposure to chromium is via the diet, the findings indicate that chromium may be transferred to infants via breast milk.
The distribution of radioactivity in rats given 51chromium as sodium dichromate intratracheally was followed for 40 days by autoradiography and scintillation counting. Three days after the administration of 0.01 mg chromium(VI)/m3 as radioactive sodium dichromate, the tissue distribution based on the relative concentrations in the tissue was lung > kidney > gastrointestinal tract > erythrocytes > liver > serum > testis > skin. Twenty-five days after dosing, the tissue distribution was lung > kidney > erythrocytes > testis > liver > serum > skin > gastrointestinal tract. Kidney, erythrocytes, and testis maintained their chromium levels for a period of 10–15 days before decreasing (Weber 1983). The distribution of chromium(VI) compared with chromium(III) was investigated in guinea pigs after intratracheal instillation of potassium dichromate or chromium trichloride. At 24 hours after instillation, 11% of the original dose of chromium from potassium dichromate remained in the lungs, 8% in the erythrocytes, 1% in plasma, 3% in the kidney, and 4% in the liver. The muscle, skin, and adrenal glands contained only a trace. All tissue concentrations of chromium declined to low or nondetectable levels in 140 days with the exception of the lungs and spleen. After chromium trichloride instillation, 69% of the dose remained in the lungs at 20 minutes, while only 4% was found in the blood and other tissues, with the remaining 27% cleared from the lungs and swallowed. The only tissue that contained a significant amount of chromium 2 days after instillation of chromium trichloride was the spleen. After 30 and 60 days, 30 and 12%, respectively, of the chromium(III) was retained in the lungs, while only 2.6 and 1.6%, respectively, of the chromium(VI) dose was retained in the lung (Baetjer et al. 1959a).
2.2 Oral exposure
Autopsy studies in the United States indicate that chromium concentrations in the body are highest in kidney, liver, lung, aorta, heart, pancreas, and spleen at birth and tend to decrease with age. The levels in liver and kidney declined after the second decade of life. The aorta, heart, and spleen levels declined rapidly between the first 45 days of life and 10 years, with low levels persisting throughout life. The level in the lung declined early, but increased again from mid life to old age (Schroeder et al. 1962).
The distribution of chromium in human body tissue after acute oral exposure was determined in the case of a 14-year-old boy who ingested 7.5 mg chromium(VI)/kg as potassium dichromate. Despite extensive treatment by dialysis and the use of the chelating agent British antilewisite, the boy died 8 days after admission to the hospital. Upon autopsy, the chromium concentrations were as follows: liver,
2.94 mg/100 cc (normal, 0.016 mg/100 cc); kidneys, 0.64 and 0.82 mg/100 cc (normal, 0.06 mg/100 cc); and brain, 0.06 mg/100 cc (normal, 0.002 mg/100 cc) (Kaufman et al. 1970). Although these data were obtained after extensive treatment to rid the body of excess chromium, the levels of chromium remaining after the treatment clearly demonstrate that these tissues absorbed at least these concentrations after an acute, lethal ingestion of a chromium(VI) compound.
Chromium may be transferred to infants via breast milk as indicated by breast milk levels of chromium in women exposed occupationally (Shmitova 1980) or via normal levels in the diet (Casey and Hambidge 1984). It has been demonstrated that in healthy women, the levels of chromium measured in breast milk are independent of serum chromium levels, urinary chromium excretion, or dietary intake of chromium (Anderson et al. 1993, Mohamedshah et al. 1998), but others (Engelhardt et al. 1990) have disputed this observation.
The tissue distribution of chromium was studied in rats administered chromium from a variety of sources. In one experiment, sodium chromate in water was administered by gavage for 7 days at 0, 1.2, 2.3, or
5.8 mg chromium(VI)/kg/day. Very little chromium (generally <0.5 μg/organ) was found in the organs analyzed (liver, spleen, lung, kidney, and blood) after administration of the two lower doses. The levels were generally comparable to those in controls. After 5.8 mg/kg/day, the largest amount of chromium (expressed as μg chromium/whole organ) was found in the liver (≈22 μg), followed by the kidney (≈7.5 μg), lung (≈4.5 μg), blood (≈2 μg), and spleen (≈1 μg). The total amount of chromium in these
tissues represented only 1.7% of the final dose of 5.8 mg/kg/day, but not all organs were analyzed. In the next experiment, rats were exposed by gavage to 7 mg chromium/kg/day for 7 days from various sources: (1) sodium chromate; (2) calcium chromate; (3) soil containing chromium (30% chromium(VI), 70% chromium(III)); or (4) a mixture of calcium chromate and the contaminated soil. The highest levels of chromium were found in liver, spleen, kidney, lung, blood, brain, and testes after dosing with sodium chromate, but the relative levels in these tissues after the other treatments followed no consistent pattern. Rats gavaged for 14 days with 13.9 mg chromium/kg/day from the four different sources had higher levels of chromium in the tissues after they were dosed with the contaminated soil or the mixture of calcium chromate and the contaminated soil than with either of the chromate salts alone. Thus, the relative organ distribution of chromium depends on the source of chromium (Witmer et al. 1989, 1991). Components in soil may affect the oxidation state and the binding of chromium to soil components, and pH of the soil may also affect the bioavailability from soil.
The chromium content in major organs (heart, lung, kidney, liver, spleen, testes) of mice receiving drinking water that provided doses of 4.8, 6.1, or 12.3 mg chromium(III)/kg/day as chromium trichloride or 4.4, 5.0, or 14.2 mg chromium(VI)/kg/day as potassium dichromate was determined after 1 year of exposure. Chromium was detected only in the liver in the chromium(III)-treated mice. Mice treated with chromium(VI) compounds had accumulation in all of the above organs, with the highest levels reported in the liver and spleen. Liver accumulation of chromium was 40–90 times higher in the chromium(VI)-treated group than in the chromium(III)-treated group (Maruyama 1982). Chromium levels in tissue (bone, kidney liver, spleen) were 9 times higher in rats given chromium(VI) as potassium chromate in drinking water for 1 year than in rats given the same concentration of chromium(III) as chromium trichloride (MacKenzie et al. 1958). In rats exposed to potassium chromate in the drinking water for 3 or 6 weeks, a general trend of increasing chromium concentration with time of exposure was apparent in the liver and kidneys, but only the kidneys showed a difference in the concentration after exposure to 100 and 200 ppm. Blood concentrations were almost saturated by 3 weeks with little further accumulation by 6 weeks. No chromium was detected in the lungs after drinking water exposure (Coogan et al. 1991a).
The distribution of potassium chromate(VI) was compared in male Fisher rats and C57BL/6J mice exposed either by drinking water (8 mg chromium(VI)/kg/day for 4 and 8 weeks) or by intraperitoneal injection (0.3 and 0.8 mg chromium(VI)/kg/day for 4 or 14 days) (Kargacin et al. 1993). The concentrations of chromium (μg/g wet tissue) after drinking water exposures for 8 weeks in mice were: liver 13.83, kidney 4.72, spleen 10.09, femur 12.55, lung 1.08, heart 1.02, muscle 0.60, and blood 0.42. These concentrations were not markedly different than for 4-week exposures. For rats, the concentrations were: liver 3.59, kidney 9.49, spleen 4.38, femur 1.78, lung 0.67, heart 1.05, muscle 0.17, and blood
0.58. These results demonstrate that considerable species differences exist between mice and rats and need to be factored into any toxicological extrapolations across species even if the routes of administration are the same. In the drinking water experiments, blood levels in rats and mice were comparable, but in intraperitoneal injection experiments, rats’ levels were about 8-fold higher than mice after 4 days of exposure. This difference appeared to be due to increased sequestering by rat red blood cells, since accumulation in white blood cells was lower in rats than mice. The higher incidence of red cell binding was also associated with greater binding of chromium to rat hemoglobin.
The higher tissue levels of chromium after administration of chromium(VI) than after administration of chromium(III) (MacKenzie et al. 1958; Maruyama 1982; Witmer et al. 1989, 1991) reflect the greater tendency of chromium(VI) to traverse plasma membranes and bind to intracellular proteins in the various tissues, which may explain the greater degree of toxicity associated with chromium(VI). In an experiment to determine the distribution of chromium in red and white blood cells, rats were exposed orally to 0.0031 mg/kg of 51chromium(VI) as sodium chromate. The 51chromium content of the fractionated blood cells was determined either 24 hours or 7 days after exposure. After 24 hours, the white blood cells contained much more 51chromium (≈250 pg chromium/billion cells) than did the red blood cells (≈30 pg chromium/billion cells). After 7 days, the 51chromium content of the white blood cells was reduced only 2.5-fold, while that of the red blood cells was reduced 10-fold. Thus, white blood cells preferentially accumulated chromium(VI) and retained the chromium longer than did the red blood cells. A small amount of chromium(III) entered red blood cells of rats after intravenous injection of 51chromium trichloride, but no 51chromium was detectable in white blood cells (Coogan et al. 1991b).
Twelve pregnant female albino rats (Druckrey strain) and 13 Swiss albino mice were exposed to 500 ppm potassium dichromate(VI) in their drinking water during pregnancy up to 1 day before delivery (Saxena et al. 1990a). The chromium(VI) daily intake was calculated to be 11.9 mg chromium(VI)/day for the rats and 3.6 mg chromium(VI)/day for mice which were considered to be maximal nontoxic doses for both species. In rats, concentrations of chromium were 0.067, 0.219, and 0.142 μg/g fresh weight in maternal blood, placenta, and fetuses, respectively, and 0.064, 0.304, and 0.366 μg/g fresh weight in mice, respectively. In treated rats, chromium levels were 3.2-fold higher in maternal blood, 3-fold higher in placenta, and 3.1-fold higher in fetal tissue when compared to control values. In treated mice, chromium levels were 2.5-fold higher in maternal blood, 3.2-fold higher in placenta, and 9.6-fold higher in fetuses when compared to control values. In treated mice, there was a significant elevation in chromium levels in placental and fetal tissues over maternal blood levels, and a significant increase in chromium levels in fetal tissue over placental concentrations when compared to controls. These differences were not observed in rats, indicating that the distribution patterns in mice and rats are different.
2.2 Dermal exposure
The findings of toxic effects in the heart, stomach, blood, muscles, and kidneys of humans who were dermally exposed to chromium compounds is suggestive of distribution to these organs. Fourteen days after a salve containing potassium chromate(VI) was applied to the skin of an individual to treat scabies, appreciable amounts of chromium were found in the blood (2– 5 mg/100 mL), urine (8 mg/L), feces (0.61 mg/100 g), and stomach contents (0.63 mg/100 mL) (Brieger 1920). The preexisting condition of scabies or the necrosis caused by the potassium chromate could have facilitated its absorption. A transient increase in the levels of total chromium in erythrocytes and plasma was observed in subjects immersed in a tank of chlorinated water containing potassium dichromate(VI) (Corbett et al. 1997).
Chromium compounds are absorbed after dermal administration by guinea pigs. Measurement of 51chromium in the organs and body fluids revealed distribution, due to dermal absorption of chromium(III) and chromium(VI) compounds, to the blood, spleen, bone marrow, lymph glands, urine, and kidneys. Absorption was greater for chromium(VI) than for chromium(III) (Wahlberg and Skog 1965).
3. Metabolism
Chromium(III) compounds are essential to normal glucose, protein, and fat metabolism. In addition, chromium(III) is capable of forming complexes with nucleic acids and proteins. Chromium(III) may also participate in intracellular reduction and oxidation reactions. Chromium(VI) is unstable inside the body and is ultimately reduced to chromium(III) in vivo by a variety of reducing agents. Chromium(V) and chromium(IV) are transient intermediates in this process.
Currently, the biological target for the essential effects of chromium(III) is unknown. Chromodulin, also referred to as GTF, has been proposed as one possible candidate (Jacquamet et al. 2003). The function of chromodulin, an oligopeptide complex containing four chromic ions, has not been established; however, a possible mechanism is that chromodulin facilitates the interaction of insulin with its cellular receptor sites, although this has not been proven (Anderson 1998a, 2003; IOM 2001).
In vivo and in vitro experiments in rats indicated that, in the lungs, chromium(VI) can be reduced to chromium(III) by ascorbate. The reduction of chromium(VI) by ascorbate results in a shorter residence time of chromium in the lungs and constitutes the first defense against oxidizing reagents in the lungs. When ascorbate is depleted from the lungs, chromium(VI) can also be reduced by glutathione. The level of ascorbic acid in the adult human lung has been estimated as approximately 7 mg/100 g wet tissue (Hornig 1975). The reduction of chromium(VI) by glutathione is slower and results in greater residence time of chromium in the lungs, compared to reduction by ascorbate (Suzuki and Fukuda 1990). Other studies reported the reduction of chromium(VI) to chromium(III) by epithelial lining fluid (ELF) obtained from the lungs of 15 individuals by bronchial lavage. The average reduction accounted for 0.6 μg chromium(VI)/mg of ELF protein. In addition, cell extracts made from pulmonary alveolar macrophages derived from five healthy male volunteers were able to reduce an average of 4.8 μg chromium(VI)/106 cells or 14.4 μg chromium(VI)/mg protein (Petrilli et al. 1986b). Metabolism of the chromium(VI) to chromium(III) by these cell fractions significantly reduced the mutagenic potency of the chromium when tested in the Ames reversion assay. Postmitochondrial (S12) preparations of human lung cells (peripheral lung parenchyma and bronchial preparations) were also able to reduce chromium(VI) to chromium(III) (De Flora et al. 1984). Moreover, large individual differences were observed (De Flora et al. 1984, 1987b), and extracts from pulmonary alveolar macrophages of smokers reduced significantly more chromium(VI) to chromium(III) than extracts from cells of nonsmokers. Because chromium(III) does not readily enter cells, these data suggest that reduction of chromium(VI) by the ELF may constitute the first line of defense against toxicity of inhaled chromium compounds. Furthermore, uptake and reduction of chromium compounds by the pulmonary alveolar macrophages may constitute a second line of defense against pulmonary toxicity of chromium(VI) compounds. Microsomal reduction of chromium(VI) occurs in the lungs mainly as it does in the liver, as discussed below.
The first defense against chromium(VI) after oral exposure is the reduction of chromium(VI) to chromium(III) in the gastric environment where gastric juice (De Flora et al. 1987a) and ascorbate (Samitz 1970) play important roles. Studies using low-frequency electron paramagnetic resonance (EPR) spectrometry have shown that chromium(VI) is reduced to chromium(V) in vivo (Liu et al. 1994, 1995, 1997a, 1997b; Ueno et al. 1995b). In vitro, low concentrations of ascorbate favor the formation of chromium(V), whereas higher concentrations of ascorbate favor the formation of the reduced oxidation state, chromium(III) (Liu et al. 1995). EPR spectrometric monitoring also showed that chromium(VI) was rapidly reduced to chromium(V) on the skin of rats, with a 3-fold greater response when the stratum corneum was removed (Liu et al. 1997a). Thus, dermal effects from direct skin contact with chromium(VI) compounds may be mediated by rapid reduction to chromium(V). In whole blood and plasma, increasing ascorbate levels led to an increased oxidation of chromium(VI) to chromium(III) (Capellmann and Bolt 1992).
For humans, the overall chromium(VI)-reducing/sequestering capacities were estimated to be 0.7–
2.1 mg/day for saliva, 8.3–12.5 mg/day for gastric juice, 11–24 mg for intestinal bacteria eliminated daily with feces, 3,300 mg/hour for liver, 234 mg/hour for males and 187 mg/hour for females for whole blood, 128 mg/hour for males and 93 mg/hour for females for red blood cells, 0.1–1.8 mg/hour for ELF, 136 mg/hour for pulmonary alveolar macrophages, and 260 mg/hour for peripheral lung parenchyma. Although these ex vivo data provide important information in the conversion of chromium(VI) to reduced states, the values may over-or underestimate the in vivo reducing capabilities (De Flora et al. 1997).
Reduction of chromium(VI) in the red blood cell occurs by the action of glutathione. Since the red blood cell membrane is permeable to chromium(VI) but not chromium(III), the chromium(III) formed by reduction of chromium(VI) by glutathione is essentially trapped within the erythrocyte for the life-span of the cell (Paustenbach et al. 2003), with approximately 1% of chromium eluting from the erythrocyte daily (ICSH 1980). Eventually, the diffusion of chromium(VI), the reduction to chromium(III), and complexing to nucleic acids and proteins within the cell will cause the concentration equilibrium to change so that more chromium(VI) is diffused through the membrane. Thus, there is a physiological drag so that increased diffusion results in greater chromium concentrations in the cell (Aaseth et al. 1982). It appears that the rate of uptake of chromium(VI) by red blood cells may not exceed the rate at which they reduce chromium(VI) to chromium(III) (Corbett et al. 1998). In vitro incubation of red blood cells with an excess of sodium chromate(VI) (10 mM) decreased glutathione levels to 10% of the original amount (Wiegand et al. 1984). The above concepts are applicable to the uptake and reduction of chromium(VI) in other cell types.
The effect of glutathione-depleting agents on the amounts of cellular chromium(III) and chromium(V) was determined in Chinese hamster V-79 cells treated with sodium chromate (Sugiyama and Tsuzuki 1994). Buthionine sulfoximine at 25 μM reduced glutatione levels to about 1% of control values, and increased chromium(V) levels by about 67%. The total chromium uptake was decreased by about 20% and chromium(III) levels were decreased by 20%. Diethylmaleate (1 mM) decreased glutathione levels to <1%, decreased chromium(V) levels by 27% and chromium(III) levels by 31%. However, the cellular uptake of total chromium was decreased to nearly 46%. The authors explained that the reason that the diethylmaleate inhibited the reduction of chromium(VI) to both chromium(III) and chromium(V) was not due to the decreased uptake, but involved the inhibition of the chromate-reducing enzymes in the cell.
In addition to the reduction of chromium(VI) by ascorbate or glutathione, in vitro studies have demonstrated reduction of chromium(VI) by microsomal enzymes. Hepatic microsomal proteins from male Sprague-Dawley rats pretreated with chromium(VI) reduced chromium(VI) to chromium(III). The rate of reduction varied both with the concentration of microsomal protein and the concentration of nicotinamide adenine dinucleotide phosphate (NADPH). In the absence of NADPH, microsomes did not reduce significant amounts of chromium(VI) over the 24-hour observation period. Therefore, the reduction of chromium(VI) in rat hepatic microsomes is NADPH-dependent (Gruber and Jennette 1978). Another study followed the kinetics of chromium(VI) reduction in hepatic microsomes from rats (Garcia and Jennette 1981). Induction of cytochrome P448 enzymes had no effect on the kinetics of the reaction, while induction of cytochrome P450 and NADPH-cytochrome P450 reductase resulted in a decrease in the apparent chromate-enzyme dissociation constant, and an increase in the apparent second-order rate constant, and no change in the apparent turnover number. Inhibition of NADPH-cytochrome P450 reductase and NADH-cytochrome b5 reductase inhibited the rate of microsomal reduction of chromium(VI), as did the addition of specific inhibitors of cytochrome P450. The results demonstrate the involvement of cytochrome P450, NADPH-dependent-cytochrome P450 reductase, and to a lesser extent cytochrome b5 and NADH-dependent-cytochrome b5 reductase, in the reduction of chromate by rat hepatic microsomes. The conversion of chromium(VI) to chromium(III) in rats can occur by electron transfer through cytochrome P450 and cytochrome b5. Both oxygen and carbon monoxide were found to inhibit the in vitro cytochrome P450 and cytochrome b5-dependent reduction of chromium(VI) (Mikalsen et al. 1989). The assertion that cytochrome P450 is involved in the reduction of chromium(VI) to chromium(III) has been further strengthened by Petrilli et al. (1985), who demonstrated that inducers of cytochrome P450 can increase the conversion of chromium(VI) to chromium(III) in S-9 mixtures prepared from the liver and lungs of exposed rats. Furthermore, it was observed that chromium(VI) can induce pulmonary cytochrome P450 and thus its own reduction in the lungs (Petrilli et al. 1985). Chromium(VI) apparently can alter the P450 activity in isolated rat microsomes. Witmer et al. (1994) demonstrated that hepatic microsomes from male rats treated with chromium(VI) resulted in a significant
decrease in hydroxylation of testosterone at the 6β, 16α, and 2α positions, indicating a decrease in the activity of P4503A1 and 3A2. In lung microsomes, an increased hydroxylation was observed at the 16α and 16β positions, indicating an increase in P450IIB1 activity. However, hepatic microsomes from treated females showed a 4-to 5-fold increase in hydroxylation activity of testosterone at the 6β position, which demonstrated that the metabolic effects of chromium differ between males and females.
Two studies have examined possible species differences in the ability of microsomes to reduce chromium(VI) (Myers and Myers 1998; Pratt and Myers 1993). Chromium(VI) reduction was enzymatic and NADPH-dependent, and the rates were proportional to the amount of microsome added. In humans, the Km for chromium(VI) was 1–3 orders of magnitude lower than Km values in rats, although the Vmax was similar. This suggests that the human liver has a much greater capacity to reduce chromium(VI) than the rat liver. Also contrary to the rodent data, oxygen and cytochrome P450 inhibitors (carbon monoxide, piperonyl butoxide, metyrapone, and aminopyrine) did not inhibit chromium(VI) reduction. These differences indicate that, in humans, cytochrome P450 does not play a significant role in the reduction process, but that other microsomal flavoproteins are responsible for reducing chromium(VI). Inhibition of flavoproteins by TlCl3 decreased chromium(VI) reduction by 96–100%, while inhibition of cytochrome c reductase (P450 reductase) by bromo-4'-nitroacetophenone resulted in an 80–85% inhibition of chromium(VI) reduction. Combined, these observations implicate P450 reductase, working independently of cytochrome P450, as a major contributor in the reduction of chromium(VI) in human microsomes. These findings suggest that metabolism of chromium(VI) in rodent systems may not readily be extrapolated to humans.
Microsomal reduction of chromium(VI) can also result in the formation of chromium(V), which involves a one-electron transfer from the microsomal electron-transport cytochrome P450 system in rats. The chromium(V) complexes are characterized as labile and reactive. These chromium(V) intermediates persist for 1 hour in vitro, making them likely to interact with deoxyribonucleic acid (DNA), which may eventually lead to cancer (Jennette 1982). Because chromium(V) complexes are labile and reactive, detection of chromium(V) after in vivo exposure to chromium(VI) was difficult in the past. More recently, Liu et al. (1994) have demonstrated that chromium(V) is formed in vivo by using low-frequency electron paramagnetic resonance (EPR) spectroscopy on whole mice. In mice injected with sodium dichromate(VI) intravenously into the tail vein, maximum levels of chromium(V) were detected within 10 minutes and declined slowly with a life-time of about 37 minutes. The time to reach peak in vivo levels of chromium(V) decreased, in a linear manner as the administered dose levels of sodium dichromate decreased. The relative tissue distributions of chromium(V) indicated that most was found in the liver and much lesser amounts in blood. None was detected in kidney, spleen, heart, or lung. When the mice were pretreated with metal ion chelators, the intensity of the EPR signal decreased demonstrating that the formation of chromium(V) was inhibited. Reactions of chromium(VI) with glutathione produced two chromium(V) complexes and a glutathione thiyl radical. Reactions of chromium(VI) with DNA in the presence of glutathione produced chromium-DNA adducts. The level of chromium-DNA adduct formation correlated with chromium(V) formation. The reaction of chromium(VI) with hydrogen peroxide produced hydroxyl radicals. Reactions of chromium(VI) with DNA in the presence of high concentrations of hydrogen peroxide (millimolar compared to 10-7–10-9 M inside cells) produced significant DNA strand breakage and the 8-hydroxy guanosine adduct, which correlated with hydroxyl radical production (Aiyar et al. 1989, 1991). Very little chromium(V) was generated by this pathway. It was postulated that the reaction of chromium(VI) with hydrogen peroxide produces tetraperoxochromium(V) species that act as a catalyst in a Fenton-type reaction producing hydroxyl radicals in which chromium(V) is continuously being recycled back to chromium(VI). The regeneration of chromium(VI) through interactions with chromium(V) and hydrogen peroxide is consistent with the findings of Molyneux and Davies (1995). As discussed above, chromium(VI) is ultimately reduced to chromium(III) within the cell. Chromium(III) can form stable complexes with DNA and protein (De Flora and Wetterhahn 1989).
The mechanism for clearance of chromium(VI) once reduced inside the liver cell may involve a chromium(III)-glutathione complex. The glutathione complex would be soluble through the cell membrane and capable of entering the bile (Norseth et al. 1982). The complexing of chromium(III) to other ligands has been shown to make them more permeable to the cell membrane (Warren et al. 1981).
4. Elimination and Excretion
4.1 Inhalation exposure
Normal urinary levels of chromium in humans have been reported to range from 0.22 to 1.8 μg/L (0.00024–0.0018 mg/L) with a median level of 0.4 μg/L (0.0004 mg/L) (IOM 2001; Iyengar and Woittiez 1988). Humans exposed to 0.05–1.7 mg chromium(III)/m3 as chromium sulfate and 0.01–0.1 mg chromium(VI)/m3 as potassium dichromate (8-hour TWA) had urinary excretion levels of 0.0247–
0.037 mg chromium(III)/L. Workers exposed mainly to chromium(VI) compounds had higher urinary chromium levels than workers exposed primarily to chromium(III) compounds. An analysis of the urine did not detect the hexavalent form of chromium, indicating that chromium(VI) was rapidly reduced before excretion (Cavalleri and Minoia 1985; Minoia and Cavalleri 1988). Chromium(III) compounds were excreted rapidly in the urine of workers, following inhalation exposure to chromium(III) as chromium lignosulfonate. Workers exposed to 0.005–0.23 mg chromium(III)/m3 had urine concentrations of 0.011–0.017 mg chromium(III)/L. The half-time for urinary excretion of chromium was short, 4–10 hours, based on an open, one-compartment kinetic model (Kiilunen et al. 1983). Tannery workers had higher urinary chromium(III) concentrations in postshift urine samples taken Friday afternoon and in preshift urine samples taken Monday, compared to controls. These workers also had hair concentrations of chromium that correlated with urinary levels. Analysis of workroom air revealed no detectable chromium(VI) and 0.0017 mg chromium(III)/m3 (time-weighted average) (Randall and Gibson 1987). Elimination of chromium(III) from hair, serum, and urine has been studied in a group of 5 men who had ceased working in a leather tannery 9 months earlier (Simpson and Gibson 1992). Compared to levels recorded during employment, the mean level of chromium in hair was reduced from 28.5 to
2.9 μmol/g; serum levels were reduced from 9.4 to 3.8 nmol/L. These levels are comparable to those in the general population. Urine levels were unchanged (13.8 nmol/L while working and 14.4 nmol/L 9 months later); the authors stated that this was probably caused by consumption of beer (a source of chromium) the night before sampling. Data from autopsy studies indicate that chromium can be retained in the lung for decades following cessation of occupational exposures (Brune et al. 1980; Hyodo et al. 1980; Mancuso 1997b).
Peak urinary chromium concentrations were observed at 6 hours (the first time point examined) in rats exposed intratracheally to 0.44 mg/kg chromium(III) as chromium acetate hydroxide or chromium(VI) as sodium dichromate (Gao et al. 1993). Chromium urinary concentrations decreased rapidly, falling from 4,535 μg chromium/g creatinine at 6 hours to 148 μg chromium/g at 72 hours for the chromium acetate hydroxide and from 2,947 μg chromium/g creatinine at 6 hours to 339 μg chromium/g at 72 hours for sodium dichromate.
Elimination of chromium was very slow in rats exposed to 2.1 mg chromium(VI)/m3 as zinc chromate 6 hours/day for 4 days. Urinary levels of chromium remained almost constant for 4 days after exposure and then decreased, indicating that chromium bound inside the erythrocyte is released slowly (Langård et al. 1978).
4.2 Oral exposure
Given the low absorption of chromium compounds by the oral route, the major pathway of excretion after oral exposure is through the feces.
An acute, oral dose of radioactive chromium(III) as chromium chloride or chromium(VI) as sodium chromate was administered to humans after which feces and urine were collected for 24 hours and 6 days, respectively, and analyzed for chromium. The amount of chromium in the 6-day fecal collection was99.6 and 89.4% of the dose for chromium(III) and chromium(VI) compounds, respectively. The amount of chromium in the 24-hour urine collection was 0.5 and 2.1% of the dose for chromium(III) and chromium(VI) compounds, respectively (Donaldson and Barreras 1966). In subjects drinking 0.001–0.1 mg chromium(VI)/kg/day as potassium chromate in water for 3 days, <2–8% of the dose was excreted in the urine (Finley et al. 1997). The percentage of the dose excreted appeared to increase with increasing dose.
Urinary excretion rates have been measured in humans after oral exposure to several chromium compounds (Finley et al. 1996b). A group of four male and two female volunteers ingested capsules containing chromium(III) picolinate at a dose of 200 μg/day for 7 days, to ensure that chromium deficiency was not a confounding factor. They then ingested 0.005 mg/kg/day chromium(VI) as potassium chromate (3 days), and 1.0 mg/kg/day chromium(III) as chromic oxide (3 days), with 3 days without dosing between the potassium chromate and chromic oxide doses. Urinary excretion rates of chromium were significantly elevated compared to postdosing control levels after seven daily doses of chromium(III) picolinate (2.4±0.8 versus 0.75±0.53 μg/day). The excretion rate increased sharply on the first of 3 days of potassium chromate dosing (11±17 μg/day) and remained steady over the next 2 days (13–14 μg/day). Excretion rates fell to 2.5±0.72 during 2 days without dosing and continued to fall during the 3 days of chromic oxide dosing, reaching rates similar to those seen postdosing. Mean pooled urinary concentrations during the dosing periods were 2.4 μg chromium/g creatinine from exposure to chromium(VI) and 0.4 μg chromium/g creatinine from exposure to chromium(III) as compared to 0.23 μg chromium/g creatinine during the postdosing time periods. The lower urinary excretion of chromium(III) after exposure to chromic oxide reflects the poorer absorption of inorganic chromium(III) compounds compared to inorganic chromium(VI) compounds.
The half-life for chromium urinary excretion after administration in drinking water as potassium dichromate has been estimated in humans (Kerger et al. 1997). Ingestion of 0.05 mg chromium(VI)/kg resulted in an extended time course of excretion. Approximately 76–82% of the 14-day total amount of chromium in the urine was excreted within the first 4 days (mean peak concentration 209 μg chromium/g creatinine; range 29–585 μg chromium/g creatinine). The average urinary excretion half-life for four of the volunteers was 39 hours at this dose. All subjects had returned to background concentrations (0.5–2.0 μg chromium/g creatinine) by 14 days postdosing. About 87% of the total amount of chromium in the urine measured over 8 days was excreted during the first 4 days for one volunteer ingesting 0.03 mg chromium(VI)/kg (peak 97 μg chromium/g creatinine on day of ingestion). Urinary chromium concentrations had returned to an average of 2.5 μg chromium/g creatinine within 7 days postdosing, the last time point measured. Urinary excretion half-life in this volunteer was 37 hours. Similar time courses of excretion were observed when volunteers took the same doses as daily doses over 3-day periods. An earlier study by this group (Kerger et al. 1996a) examined urinary excretion half-lives following a bolus dose of 10 ppm (approximately 0.06 mg chromium/kg) chromium(III) chloride, potassium dichromate reduced with orange juice (presumably, the juice reduced the potassium dichromate to chromium(III)-organic complexes and chromium(III) ions), or potassium dichromate. The calculated urinary excretion half-lives for the three chromium solutions were 10.3, 15, and 39.3 hours, respectively. The potassium dichromate half-life is consistent with the results from the Kerger et al. (1997) study. If, in these studies, all of the absorbed chromium(VI) was rapidly and completely converted to chromium(III), there should be no difference in urinary half-life. The difference in excretion half-lives following dosing with chromium(III) and chromium(VI) appears to reflect incomplete reduction of absorbed chromium(VI) to chromium(III) as well as longer retention of chromium(VI) in tissues. The prolonged half-life following dosing with chromium(VI) appears to be a composite of the half-lives the chromium(VI) and chromium(III) derived from the reduction of chromium(VI) in the blood. Given that most is converted to chromium(III), the half-life for the sequestered chromium is quite long (much longer than 40 hours) and reflects the half-life of chromium observed in the red blood cells. Pretreatment of chromium(VI) with orange juice apparently did not convert all chromium(VI) to chromium(III), as indicated by a half-life of 15 hours.
An estimate of the half-life of elimination from plasma has been reported in humans. Uptake of potassium dichromate was determined in a man who was given 0.8 mg of chromium(VI) in drinking water 5 times each day for 17 days (Paustenbach et al. 1996). Steady-state concentrations of chromium in blood were attained after 7 days and a plasma elimination half-life of 36 hours was estimated.
Measurement of the chromium content in 255 milk samples from 45 lactating American women revealed that most samples contained <0.4 μg/L with a mean value of 0.3 μg/L (Casey and Hambidge 1984). Another study (Anderson et al. 1993) measured chromium levels in the breast milk of 17 women 60 days postpartum, and reported mean levels of ~0.2 μg/L. Lactation, therefore, represents a route of excretion of chromium and a potential route of exposure to the nursing infant. However, the precise relationship between maternal chromium levels and levels in breast milk is unclear, if such a relationship exists at all (Anderson et al. 1993; Engelhardt et al. 1990; Mohamedshah et al. 1998).
Chromium can be excreted in hair and fingernails. Mean trace levels of chromium detected in the hair of individuals from the general population of several countries were as follows: United States, 0.23 ppm; Canada, 0.35 ppm; Poland, 0.27 ppm; Japan, 0.23 ppm; and India, 1.02 ppm (Takagi et al. 1986). Mean levels of chromium in the fingernails of these populations were: United States, 0.52 ppm; Canada,
0.82 ppm; Poland, 0.52 ppm; Japan, 1.4 ppm; and India, 1.3 ppm (Takagi et al. 1988).
Rats given 18 mg chromium(VI)/kg as potassium dichromate by gavage excreted about 25 μg chromium in the first 24 hours after dosing and ≈10 μg chromium in each of the next 24-hour periods (Banner et al. 1986).
In rats and hamsters fed chromium compounds, fecal excretion of chromium varied slightly from 97 to 99% of the administered dose. Urinary excretion of chromium varied from 0.6 to 1.4% of the dose administered as either chromium(III) or chromium(VI) compounds (Donaldson and Barreras 1966; Henderson et al. 1979; Sayato et al. 1980). The urinary and fecal excretion over 2-day periods in rats treated for 8 days by gavage with 13.92 mg chromium/kg/day in corn oil was higher when soil containing 70% chromium(III) and 30% chromium(VI) was the source of chromium than when chromium(VI) as calcium chromate was the source. Total urinary and fecal excretion of chromium on days 1 and 2 of dosing were 1.8 and 19%, respectively, of the dose from soil and <0.5 and 1.8%, respectively, of the dose from calcium chromate. Total urinary and fecal excretion of chromium on days 7 and 8 of dosing were higher than on days 1 and 2. For contaminated soil, urinary excretion was 1.12% and fecal excretion was 40.6% of the dose. For calcium chromate, urinary excretion was 0.21% and fecal excretion was 12.35% of the dose (Witmer et al. 1991). Whether the higher excretion of chromium after dosing with soil than with the chromate salt represents greater bioavailability from soil could not be determined because about 50% of the administered dose could not be accounted for from the excretion and distribution data. Excretion of chromium(III) in dogs was approximately equal to the clearance of creatinine, indicating little tubular absorption or reabsorption of chromium in the kidneys (Donaldson et al. 1984).
4.3 Dermal exposure
Information regarding the excretion of chromium in humans after dermal exposure to chromium or its compounds is limited. Fourteen days after application of a salve containing potassium chromate(VI), which resulted in skin necrosis and sloughing at the application site, chromium was found at 8 mg/L in the urine and 0.61 mg/100 g in the feces of one individual (Brieger 1920). A slight increase (over background levels) in urinary chromium levels was observed in four subjects submersed in a tub of chlorinated water containing 22 mg chromium(VI)/L as potassium dichromate(VI) for 3 hours (Corbett et al. 1997). For three of the four subjects, the increase in urinary chromium excretion was <1 μg/day over the 5-day collection period.
51Chromium was detected in the urine of guinea pigs after radiolabeled sodium chromate(VI) or chromium(III) trichloride solutions were placed over skin depots that were monitored by scintillation counting to determine the dermal absorption (Wahlberg and Skog 1965).
Information on Registered Substances comes from registration dossiers which have been assigned a registration number. The assignment of a registration number does however not guarantee that the information in the dossier is correct or that the dossier is compliant with Regulation (EC) No 1907/2006 (the REACH Regulation). This information has not been reviewed or verified by the Agency or any other authority. The content is subject to change without prior notice.
Reproduction or further distribution of this information may be subject to copyright protection. Use of the information without obtaining the permission from the owner(s) of the respective information might violate the rights of the owner.
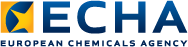