Registration Dossier
Registration Dossier
Data platform availability banner - registered substances factsheets
Please be aware that this old REACH registration data factsheet is no longer maintained; it remains frozen as of 19th May 2023.
The new ECHA CHEM database has been released by ECHA, and it now contains all REACH registration data. There are more details on the transition of ECHA's published data to ECHA CHEM here.
Diss Factsheets
Use of this information is subject to copyright laws and may require the permission of the owner of the information, as described in the ECHA Legal Notice.
EC number: 203-090-1 | CAS number: 103-23-1
- Life Cycle description
- Uses advised against
- Endpoint summary
- Appearance / physical state / colour
- Melting point / freezing point
- Boiling point
- Density
- Particle size distribution (Granulometry)
- Vapour pressure
- Partition coefficient
- Water solubility
- Solubility in organic solvents / fat solubility
- Surface tension
- Flash point
- Auto flammability
- Flammability
- Explosiveness
- Oxidising properties
- Oxidation reduction potential
- Stability in organic solvents and identity of relevant degradation products
- Storage stability and reactivity towards container material
- Stability: thermal, sunlight, metals
- pH
- Dissociation constant
- Viscosity
- Additional physico-chemical information
- Additional physico-chemical properties of nanomaterials
- Nanomaterial agglomeration / aggregation
- Nanomaterial crystalline phase
- Nanomaterial crystallite and grain size
- Nanomaterial aspect ratio / shape
- Nanomaterial specific surface area
- Nanomaterial Zeta potential
- Nanomaterial surface chemistry
- Nanomaterial dustiness
- Nanomaterial porosity
- Nanomaterial pour density
- Nanomaterial photocatalytic activity
- Nanomaterial radical formation potential
- Nanomaterial catalytic activity
- Endpoint summary
- Stability
- Biodegradation
- Bioaccumulation
- Transport and distribution
- Environmental data
- Additional information on environmental fate and behaviour
- Ecotoxicological Summary
- Aquatic toxicity
- Endpoint summary
- Short-term toxicity to fish
- Long-term toxicity to fish
- Short-term toxicity to aquatic invertebrates
- Long-term toxicity to aquatic invertebrates
- Toxicity to aquatic algae and cyanobacteria
- Toxicity to aquatic plants other than algae
- Toxicity to microorganisms
- Endocrine disrupter testing in aquatic vertebrates – in vivo
- Toxicity to other aquatic organisms
- Sediment toxicity
- Terrestrial toxicity
- Biological effects monitoring
- Biotransformation and kinetics
- Additional ecotoxological information
- Toxicological Summary
- Toxicokinetics, metabolism and distribution
- Acute Toxicity
- Irritation / corrosion
- Sensitisation
- Repeated dose toxicity
- Genetic toxicity
- Carcinogenicity
- Toxicity to reproduction
- Specific investigations
- Exposure related observations in humans
- Toxic effects on livestock and pets
- Additional toxicological data

Bioaccumulation: aquatic / sediment
Administrative data
Link to relevant study record(s)
- Endpoint:
- bioaccumulation in aquatic species: fish
- Type of information:
- experimental study
- Adequacy of study:
- key study
- Reliability:
- 2 (reliable with restrictions)
- Rationale for reliability incl. deficiencies:
- other: Acceptable, well documented and peer reviewed publication which meets basic scientific principles
- Qualifier:
- according to guideline
- Guideline:
- other: flow through system according to ASTM
- Principles of method if other than guideline:
- A 28 d uptake and 14 d depuration bioconcentration study of the bluegill sunfish was conducted following U.S. EPA and ASTM procedures.
- GLP compliance:
- not specified
- Radiolabelling:
- no
- Vehicle:
- no
- Test organisms (species):
- Lepomis macrochirus
- Route of exposure:
- aqueous
- Test type:
- flow-through
- Water / sediment media type:
- natural water: freshwater
- Total exposure / uptake duration:
- 28 d
- Total depuration duration:
- 14 d
- Hardness:
- 265 mg/L
- pH:
- 7.8 - 8.3
- Dissolved oxygen:
- 9.2 - 10.2 mg/L
- Details on test conditions:
- TEST WATER
alkalinity of 350 mg/L
conductance of 700 micro ohm/cm
TEST PROCEDURE
- A proportional diluter delivered water and DOA in acetone to the test and control aquaria at a rate of 10 replacement volumes per day.
- The nominal exposure concentration was 0.20 mg/L.
- The test concentration was confirmed by radioanalysis before test fish were introduced.
- Groups of 130 fish were transferred to the control and test chambers.
- The fish were observed initially and every 24 h during the exposure period for mortality and unusual behaviour.
- Water and fish were sampled during the uptake period at 4 h and 1, 3, 7, 14, 21 and 28 d. Muscle filet and viscera portions were analyzed.
- Individual samples were converted to 14CO2 by oxidation/combustion in a Packard 306B Tricarb Sample Oxidizer. Radioassay of the combustion gases were performed by liquid scintillation counting.
- On day 28 of the exposure period, the addition of the [14C]DOA test material was terminated and the fish were exposed to flowing uncontaminated water for an additional 14 d. During this period, water and fish were sampled on days 29, 31, 35, 38 and 42 and analyzed as during the uptake period.
MEASUREMENT
- The BCF measured by using 14-C-labelled test substance.
- The BCF was calculated from the fresh weight.
- The half life of the labelled 14-C was < 1 day. - Nominal and measured concentrations:
- 0.25 mg/L
- Key result
- Type:
- BCF
- Value:
- 27 dimensionless
- Basis:
- whole body w.w.
- Validity criteria fulfilled:
- not applicable
Reference
Description of key information
Bis(2-ethylhexyl) adipate does not significantly accumulate in organisms.
Key value for chemical safety assessment
- BCF (aquatic species):
- 27 L/kg ww
Additional information
One study investigating the bioaccumulation potential of Bis(2-ethylhexyl) adipate (DEHA, CAS 103-23-1) is available. The potential for accumulation of the poorly soluble, highly lipophilic substance in aquatic organisms was examined in a bioconcentration test with bluegill sunfish (Lepomis macrochirus) using 14C-labelled DEHA (Felder et al. 1986). The test was carried out for 42 days. Concentrations of DEHA in water, whole fish, viscera, and fillet were analyzed at intervals during the test. After the first 35 days of exposure, the remaining fish were exposed to clean water for an additional 14 days and concentrations of DEHA were measured in the fish at intervals. A whole fish bioconcentration factor (BCF) of 27 was reported at day 35. Following exposure to clean water, a depuration rate for DEHA of 0.26/day (t 1/2 = 2.7 days) was determined. The results imply that the accumulation of DEHA is low despite a high log Pow (log Pow = 8.94), most likely due to rapid metabolization. Furthermore, when transferred to freshwater, the substance is apparently rapidly and extensively excreted from the fish. Similar results were observed in monkeys, rats and mice (see chapter 7.1.1 for details) and by valid QSARcalculations considering metabolism (BCFBAF v3.01, Arnot-Gobas method and Catalogic v5.11.9.8 BCF base-line model v02.05).
This experimental result can be easily explained by the general enzymatic processes, which are numerously published in the scientific literature: If taken up by living organisms, aliphatic esters such as DEHA will be initially metabolized via enzymatic hydrolysis to the respective dicarboxylic acid and alcohol components as would dietary fats (e.g., Linfield 1984, Lehninger 1970, Mattson and Volpenhein 1972). The hydrolysis is catalyzed by carboxylesterases and esterases, with B-esterases located in hepatocytes of mammals being the most important (e.g., Heymann 1980). Carboxylesterase activity has also been reported from a wide variety of tissues in invertebrates and fishes (e.g., Barron et al. 1999, Wheelock et al. 2008). In fish, the high catalytic activity, low substrate specificity and wide distribution of the enzymes in conjunction with a high tissue content lead to a rapid biotransformation of aliphatic esters, which significantly reduces its bioaccumulation potential (Lech and Melancon 1980, Lech and Bend 1980).
2-ethylhexanol and adipic acid are the expected hydrolysis products from the enzymatic reaction catalyzed by carboxylesterase. These metabolites exhibit no potential for bioaccumulation (BCF < 50, BCFBAF v3.01 calculation): The metabolism of alcohols has been extensively reviewed in the literature (e.g., see Rizzo et al. 1987, Hargrove et al. 2004). The free alcohols can either be esterified to form wax esters (which are similar to triglycerides) or they can be transformed to fatty acids in a two-step enzymatic process catalyzed by alcohol dehydrogenase (ADH) and aldehyde dehydrogenase (ALDH). The responsible enzymes ADH and ALDH are present in a large number of animals including plants, microorganisms and fish (e.g., Sund and Theorell 1963, Nilsson 1990, Watabiki et al. 1999, Reimers et al. 2004, Lassen et al. 2005).The metabolism of alcohols in fish was extensively studied by Reimers et al. (2004). They isolated and characterized two cDNAs from the zebra fish, Danio rerio, encoding ADHs, which showed specific metabolic activity in in-vitro assays with various alcohol components ranging from C4 to C8. The emerging aldehydes were shown to be further oxidized to the corresponding fatty acid by ALDH enzymes. The most effective ALDH2, which is mainly located in the mitochondria of liver cells showed a sequence similarity of 75% to mammalian ALDH2 enzymes and a similar catalytic activity (also see Nilsson 1988).
These assumptions are confirmed by metabolism studies performed with DEHA or the hydrolysis product 2-ethylhexanol (2-EH) in rats. Guest et al. (1985) showed that at a single oral dose (of 50 or 500 mg/kg 14C-labeled DEHA), B6C3F1 mice excreted 91, 7, and 1 to 2 % of the radioactive dose in the urine, feces, and expired air in 24 hours, respectively. El-Hawari et al. (1985) administered 500 mg/kg of 14C-labeled DEHA to test animals and in 24 hours observed over 91 percent and 74 percent of the administered dose in the urine of mice and rats, respectively. Takahashi et al. (1981) administered a single oral dose of 500 mg/kg to two male Wistar rats. Eighty-six percent of the administered dose was excreted within 24 hours and over 90 percent of the dose in 48 hours. Roughly equal amounts of radioactivity were excreted in breath and urine. Deisinger et al. (1994) conducted excretion balance studies with 2-EH in female Fischer 344 rats following single high and low oral doses, repeated oral dosing and dermal exposure.The high, low and repeated low oral dose studies showed similar excretion balance profiles with some evidence of metabolic saturation at the high dose. No evidence of metabolic induction was seen following repeated low oral dosing. All of the oral doses were eliminated rapidly, predominantly in the urine during the first 24 h following dosing. The dermal dosing resulted in only about 5% absorption of the 1 g/kg dose, with the major portion of the dose recovered unabsorbed from the dermal exposure cell at 6h. Urinary metabolites eliminated following the oral and dermal doses were predominately glucuronides of oxidized metabolites, including glucuronides of 2-ethyladipic acid, 2-ethylhexanoic acid, 5-hydroxy-2-ethylhexanoic acid and 6-hydroxy-2-ethylhexanoic acid. Albro (1975) found that 14C associated with 2-ethyl[14C]hexanol was rapidly excreted in respiratory CO2 (6-7%), faeces (8-9%) and urine (80-82%), with essentially complete elimination by 28 h after administration. The amount of label recovered in 14CO2 matched the amount of unlabelled 2-heptanone plus 4-heptanone recovered from urine, suggesting that both types of metabolite may have been derived from the major urinary metabolite, 2-ethyl-hexanoic acid, by decarboxylation following partialβ-oxidation. El-Hawari et al. (1985) administered radiolabeled DEHA to Cynomolgus monkeys via the oral route and found that 49-69 percent of the radioactivity was excreted in the urine and 23-40 percent in the feces by 48 hours after administration.
Metabolism data for adipic acid in aquatic organisms is not available. However, metabolism of adipic acid in rats has been studied by Rusoff et al. (1960). The investigators administered approximately 50 mg 14C-labeled adipic acid to rats by gavage and within 24 hours recovered up to 70 percent of the radioactivity in the breath as carbon dioxide. In addition to adipic acid, Rusoff et al. (1960) also detected five radioactive metabolites including urea, glutamic, lactic,β-ketoadipic, and citric acids in the urine. Due to the presence ofβ-ketoadipic acid in the urine, they suggested that some of the administered adipic acid underwent β-oxidation to β-ketoadipic acid which in turn could be further metabolized to succinic acid.
Two conflicting result are available. One study reports a BCF value of 20770 for DEHA in the common mussel Mytilus edulis (Brown 1984a) and the other study a BCF value of 815 in Daphnia magna (Brown 1984b). The lower test concentration used in the study with the mussel was 5 µg/L, which is above the water solubility of the substance (3.2 µg/L).
Acetone was used as vehicle for the test solution preparation in both studies. On the one hand the mussels and daphnids might well have filtered and ingested undissolved material, adding to their body burden. Additionally, mussels and daphnids were fed microalgae. Again, animals possibly have taken up undissolved test material, which has been attached to the surface of or incorporated by the algae.
Finally and most importantly, measurements were based on 14C-activity and not on the substance itself. Thus, radioactivity measured in the mussels and daphnids may be due to (i) incorporation of 14C during metabolic processes (see metabolism of adipic acid) or via ingestion of algae, which assimilated 14CO2. In summary, the results of the studies – if at all valid – are likely to clearly overestimate the bioaccumulation potential of bis(2-ethylhexyl) adipate. Therefore, and since more valid information is available, the studies are not taken into account to assess the bioaccumulation potential of bis(2-ethylhexyl) adipate.
Conclusion
DEHA is not expected to be bioaccumulative. Due to their readily biodegradable nature, extensive degradation of these substances in conventional STPs will take place and only low concentrations are expected to be released into the environment. Once present in the aquatic compartment, further biodegradation will occur and, depending on their log Pow, water solubility and adsorption potential, DEHA (and its metabolites) will be bioavailable to aquatic organisms such as fish mainly via water or on the other hand via feed and contact with suspended organic particles. After uptake by fish species, extensive and fast biotransformation of DEHA by carboxylesterases into adipic acid and via 2-ethylhexanol to 2-ethylhexanoic acid is expected. The alcohol is used by these organisms as their main source of energy throughout all the different life stages (early development, growth, reproduction, etc.). Adipic acid does not have the potential to accumulate in adipose tissue due to their low log Pow. The key study reports a BCF value of 27, which clearly indicate that rapid metabolism takes place even when log Pow values are above the trigger value of 4.5. Supporting BCF/BAF values, estimated using EPISuite and Catalogic models, confirm the experimental result (all well below 2000).
The information above provides strong evidence supporting the statement that rapid metabolism and low bioaccumulation potential can be expected for DEHA and its metabolites.
References
Albro PW. 1975. The metabolism of 2-ethylhexanol in rats. Xenobiotica 5: 625-636
Barron MG et al. 1999. Tissue carboxylesterase activity of rainbow trout. Environ Toxicol Chem 18(11): 2506-2511
Deisinger PJ, Boatman RJ, Guest D. 1994. Metabolism of 2-ethylhexanol administered orally and dermally to the female Fischer 344 rat. Xenobiotica 24(5): 429-440
El-Hawari M, Murrill E, Stoltz M, Skalsky H, Guest D. 1985. Species differences in the disposition and metabolism of di-(2-ethylhexyl)adipate (DEHA).Toxicologist 5: 237
Felder et al. 1986. Assessment of the safety of dioctyl adipate in freshwater environments. Environ Toxicol Chem 5: 777-784
Guest D, Pallas F, Northup S, Moran E, El-Hawari M. 1985. Metabolic studies with di(2-ethylhexyl)adipate (DEHA) in the mouse. Toxicologist 5: 237
Heymann E. 1980. Carboxylesterases and amidases. Pp 291-316. In: Jakoby WB (ed) Enzymatic basis of detoxification Vol 2. Biochem Pharmacol Toxicol: A series of monographs, Academic Press
Hargrove JL. 2004. Nutritional significance and metabolism of very long chain fatty alcohols and acids from dietary waxes. Exp Biol Med 229: 215-226
Lassen N et al. 2005. Molecular cloning, baculovirus expression and tissue distribution of the zebrafish aldehyde dehydrogenase 2. Drug Metabol Disposit 33(5): 649-656
Lech JJ and Bend JR. 1980. Relationship between biotransformation and the toxicity and fate of xenobiotic chemicals in fish. Environmental Health Perspectives 34: 115-131
Lech JJ and Melancon MJ. 1980. Uptake, metabolism, and elimination of 14c‐labeled 1,2,4‐trichlorobenzene in rainbow trout and carp. J Toxicol Environ health 6(3): 645-658
Lehninger AL. 1970. Biochemistry. Worth Publishers, Inc.
Linfield WM et al. 1984. Enzymatic fat hydrolysis and synthesis. J Am Oil Chem Soc 61(2): 191-195
Mattson FH and Volpenhein RA. 1972.Hydrolysis of fully esterified alcohols containing from one to eight hydroxyl groups by the lipolytic enzymes of rat pancreatic juice. J Lip Res 13: 325-328
Nilsson GE. 1990. Distribution of aldehyde dehydrogenase and alcohol dehydrogenase in summer-acclimatized crucian carp, Carassius carassius L. J Fish Biol 36(2): 175-179
Nilsson GE. 1988. A comparative study of aldehyde dehydrogenase and alcohol dehydrogenase activities in crucian carp and three other vertebrates: apparent adaptations to ethanol production. J Comp Physiol 158(4): 479-485
Reimers et al. 2004. Two zebrafish alcohol dehydrogenases share common ancestry with mammalian class I, II, IV, and V alcohol dehydrogenase genes but have distinct functional characteristics. J Biol Chem 279: 38303-38312
Rizzo WB et al. 1987. Fatty alcohol metabolism in cultured human fibroblasts. Evidence for a fatty alcohol cycle. J Biol Chem 262: 17412-17419
Rusoff II, Baldwin RR, Domingues FJ, Monder C, Ohan WJ, Thiessen R Jr. 1960. Intermediary metabolism of adipic acid. Toxicol Appl Pharmacol 2: 316-330
Sund H and Theorell H. 1963. Alcohol dehydrogenases. The Enzymes 7: 25-83
Takahashi T, Tanaka A, Yamaha T. 1981.Elimination, distribution and metabolism of di-(2-ethylhexyl)adipate (DEHA) in rats. Toxicology 22: 223-233
Watabiki T et al. 1999. Intralobular distribution of class I alcohol dehydrogenase and aldehyde dehydrogenase 2 activities in the hamster liver. Alc Clinic Exp Res 23: 52-55
Wheelock CE et al. 2008. Applications of carboxylesterase activity in environmental monitoring and toxicity identification evaluations (TI Es).Rev Environ Contam Toxicol 195: 117-178
Information on Registered Substances comes from registration dossiers which have been assigned a registration number. The assignment of a registration number does however not guarantee that the information in the dossier is correct or that the dossier is compliant with Regulation (EC) No 1907/2006 (the REACH Regulation). This information has not been reviewed or verified by the Agency or any other authority. The content is subject to change without prior notice.
Reproduction or further distribution of this information may be subject to copyright protection. Use of the information without obtaining the permission from the owner(s) of the respective information might violate the rights of the owner.
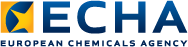