Registration Dossier
Registration Dossier
Data platform availability banner - registered substances factsheets
Please be aware that this old REACH registration data factsheet is no longer maintained; it remains frozen as of 19th May 2023.
The new ECHA CHEM database has been released by ECHA, and it now contains all REACH registration data. There are more details on the transition of ECHA's published data to ECHA CHEM here.
Diss Factsheets
Use of this information is subject to copyright laws and may require the permission of the owner of the information, as described in the ECHA Legal Notice.
EC number: 200-821-6 | CAS number: 74-90-8
- Life Cycle description
- Uses advised against
- Endpoint summary
- Appearance / physical state / colour
- Melting point / freezing point
- Boiling point
- Density
- Particle size distribution (Granulometry)
- Vapour pressure
- Partition coefficient
- Water solubility
- Solubility in organic solvents / fat solubility
- Surface tension
- Flash point
- Auto flammability
- Flammability
- Explosiveness
- Oxidising properties
- Oxidation reduction potential
- Stability in organic solvents and identity of relevant degradation products
- Storage stability and reactivity towards container material
- Stability: thermal, sunlight, metals
- pH
- Dissociation constant
- Viscosity
- Additional physico-chemical information
- Additional physico-chemical properties of nanomaterials
- Nanomaterial agglomeration / aggregation
- Nanomaterial crystalline phase
- Nanomaterial crystallite and grain size
- Nanomaterial aspect ratio / shape
- Nanomaterial specific surface area
- Nanomaterial Zeta potential
- Nanomaterial surface chemistry
- Nanomaterial dustiness
- Nanomaterial porosity
- Nanomaterial pour density
- Nanomaterial photocatalytic activity
- Nanomaterial radical formation potential
- Nanomaterial catalytic activity
- Endpoint summary
- Stability
- Biodegradation
- Bioaccumulation
- Transport and distribution
- Environmental data
- Additional information on environmental fate and behaviour
- Ecotoxicological Summary
- Aquatic toxicity
- Endpoint summary
- Short-term toxicity to fish
- Long-term toxicity to fish
- Short-term toxicity to aquatic invertebrates
- Long-term toxicity to aquatic invertebrates
- Toxicity to aquatic algae and cyanobacteria
- Toxicity to aquatic plants other than algae
- Toxicity to microorganisms
- Endocrine disrupter testing in aquatic vertebrates – in vivo
- Toxicity to other aquatic organisms
- Sediment toxicity
- Terrestrial toxicity
- Biological effects monitoring
- Biotransformation and kinetics
- Additional ecotoxological information
- Toxicological Summary
- Toxicokinetics, metabolism and distribution
- Acute Toxicity
- Irritation / corrosion
- Sensitisation
- Repeated dose toxicity
- Genetic toxicity
- Carcinogenicity
- Toxicity to reproduction
- Specific investigations
- Exposure related observations in humans
- Toxic effects on livestock and pets
- Additional toxicological data

Additional toxicological data
Administrative data
- Endpoint:
- additional toxicological information
- Type of information:
- experimental study
- Adequacy of study:
- weight of evidence
- Reliability:
- 2 (reliable with restrictions)
- Rationale for reliability incl. deficiencies:
- other: see 'Remark'
- Remarks:
- Experimental data was reviewed by the ECETOC Task Force, author of the JACC Report No. 53, “Cyanides of Hydrogen, Sodium and Potassium, and Acetone Cyanohydrin (CAS No. 74-90-8, 143-33-9, 151-50-8 and 75-86-5)”, 2007. The report is a weight of evidence approach to an extensive body of literature, much of which was undertaken prior to development of guidelines. The report was peer reviewed by the scientific non-governmental organization (NGO), which judged the data to be reliable with restrictions.
Data source
Reference
- Reference Type:
- review article or handbook
- Title:
- Unnamed
- Year:
- 2 007
Materials and methods
Test material
- Reference substance name:
- Hydrogen cyanide
- EC Number:
- 200-821-6
- EC Name:
- Hydrogen cyanide
- Cas Number:
- 74-90-8
- Molecular formula:
- CHN
- IUPAC Name:
- hydrogen cyanide
Constituent 1
Results and discussion
Applicant's summary and conclusion
- Conclusions:
- Cyanide poisoning is caused by complex formation with the iron in cytochrome oxidase which is
present in tissues at cellular level. The complex formation inhibits oxygen from receiving electrons from the cytochrome oxidase and a so-called intracellular or cytotoxic anoxia occurs, i.e. oxygen is present but cannot be utilised by the cell. Because neurons and cardiac myocytes are highly dependent on aerobic metabolism they are extremely sensitive to the deprivation of oxygen. If aerobic metabolism fails due to the inactivated cytochrome oxidase by cyanide, the neuron immediately loses its capacity to conduct nervous pulses properly and the brain fails to function with consequent loss of consciousness. If this stage continues for some minutes, the damage becomes irreversible and the neurons die. For these reasons, prolonged hypoxia, regardless of its cause, often results in injury to the brain. Toxicants that inhibit aerobic cell respiration like HCN and hydrogen sulphide have the same effect (Anthony and Graham, 1991).
Molecular mechanism of action: Hypoxic anoxia
Over the last 6 decades, a number of authors have worked on the identification of the molecular
mode of action of cyanide poisoning. In this evaluation emphasis is placed on the more recent work, in particular of the group of Isom (Isom et al, 1975, 1982) on the molecular basis of sublethal cyanide intoxication that has been conducted using model cell cultures of neuronal cells (e.g. undifferentiated or differentiated rat phaeochromocytome PC12 cells, cerebellar granular cells), rodent brain slices and in vivo experiments.
The role of cytochrome oxidase inhibition and depletion of ATP energy equivalents has been
identified relatively early (Albaum et al, 1946; Johnson et al, 1987a). Brain and liver are the main
target organs for cytochrome c inhibition and ATP depletion (Isom et al, 1982). This leads to an
inhibition of oxidative glucose metabolism in favour of anaerobic pathways, such as the pentosephosphate shunt and non-enzymatic pyruvate formation, and results in an increased formation of NADPH and lactate (Figure 26) (Isom et al, 1975).
At the cellular level, ATP depletion leads to an inhibition of Ca2+ and Ca2+/Mg2+ dependent K-Na-ATPases that regulate, inter alia, cytosolic levels of calcium ions. A dose-dependent increase in cytosolic calcium levels occurs with a certain time delay indicating a metabolic process rather than a direct interaction with ion channels. Most of the effects that follow can, at least partially, be suppressed by calcium antagonists.
More recent studies have shown that cyanide may increase intracellular calcium levels by two
additional mechanisms. Initially, intracellular calcium may be mobilised by a reaction activated
by phospholipase C, leading to formation of polyphosphoinosite hydrolysis and inositol triphosphate (IP3). Low levels of cyanide very rapidly resulted in elevated IP3 levels in a cell culture system. IP3 is known to mobilise Ca2+ from intracellular storage compartments (Yang et al, 1996). A delayed calcium influx might be mediated by the N-methyl-D-aspartate (NMDA) receptor channel. Initial mobilisation of intracellular calcium in brain cells leads to an increased release of glutamic acid, an excitatory central neurotransmitter. Increased calcium influx through glutamate gated NMDA-receptor channels leads to a prolonged release of glutamic acid. High concentrations of glutamic acid can, in turn, induce neuronal degeneration due to excessive calcium influx. NMDA-mediated calcium influx is also triggered via IP3 and a receptor-mediated phospholipase C (Patel et al, 1991; Yang et al, 1996). Increase of cytosolic calcium triggers a number of other processes that have been observed
following cyanide exposure.
1. Formation of reactive oxygen species (ROS) and NO is mediated by an activation of phosphokinase C (PKC). PKC activates phospholipase A2 (PLA2) and NO synthetase. The latter leads to an increased NO formation. PLA2 activates the arachidonic acid cascade and both cyclo-oxygenase (in particular cyclo-oxygenase-2) and lipoxygenase are activated, leading to increased levels of ROS (Gunasekar et al, 1998) in the endoplasmic reticulum (Kanthasamy et al, 1997). The elevated ROS and NO levels can explain the increased lipid peroxidation that was observed by several authors following cyanide treatment (Johnson et al, 1987a; Ardelt et al, 1989, 1994) in particular in the endoplasmic reticulum membranes (Ardelt et al, 1994). The elevated ROS and NO levels also underlie formation of Malone dialdehyde resulting in cytotoxicity and apoptotic cell death (Mills et al, 1996).
2. Differentiated neuronal cells have been demonstrated to be more sensitive to ROS-induced apoptotic cell death following cyanide exposure than undifferentiated neuronal cells (Mills et al, 1996).
3. Cyanide-mediated elevated intracellular calcium levels lead to an activation of brain xanthine oxidase, thereby inducing an inhibition of antioxidative enzymes such as catalase, GSH dependent peroxidase and GSH reductase, and consequently depletion of reduced glutathione (GSH) (Ardelt et al, 1989). This implies that the cells’ ability to neutralise increased levels of ROS is also impaired adding to the effect of lipid peroxidation as described under 1.
4. Elevated intracellular calcium levels also cause the increased release of excitatory neurotransmitters like dopamine and noradrenaline from granular stores within neuronal cells in brain and periphery (Kanthasamy et al, 1991a). Adrenal catecholamine secretion may also be enhanced as a secondary effect (Borowitz et al, 1988). This contributes to an increased energy demand of the organism that aggravates the consequences of cyanide-induced ATP reduction and leads to a spread of the cytotoxic effect to other energy demanding organs, such as the heart which are less sensitive to the primary effect of cyanides.
Thiocyanate mediated
The secondary mechanism of action relevant to repeated exposure to cyanide is formation of the primary metabolite thiocyanate. The main target for thiocyanate is the thyroid gland. Thiocyanate is a competitive inhibitor of the iodine uptake by the thyroid, causing depletion of iodine in the thyroid depending on the ratio of thiocyanate and iodine levels in blood. Reduced intra-thyroidal iodine levels result in reduced thyroid hormone synthesis, excretion and blood levels, in particular tri-iodothyronin (T3) and serum thyroxin (T4). The lower T3 and T4 levels, in turn, up-regulate the hypothalamus, increasing secretion of thyrotropin releasing hormone (TRH). This leads to an increased secretion of thyroid stimulating hormone (TSH) by the pituitary and an increase in hormone producing cells of the thyroid that may result in hyperthyroidism (goitre) formation.
Information on Registered Substances comes from registration dossiers which have been assigned a registration number. The assignment of a registration number does however not guarantee that the information in the dossier is correct or that the dossier is compliant with Regulation (EC) No 1907/2006 (the REACH Regulation). This information has not been reviewed or verified by the Agency or any other authority. The content is subject to change without prior notice.
Reproduction or further distribution of this information may be subject to copyright protection. Use of the information without obtaining the permission from the owner(s) of the respective information might violate the rights of the owner.
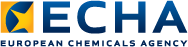