Registration Dossier
Registration Dossier
Data platform availability banner - registered substances factsheets
Please be aware that this old REACH registration data factsheet is no longer maintained; it remains frozen as of 19th May 2023.
The new ECHA CHEM database has been released by ECHA, and it now contains all REACH registration data. There are more details on the transition of ECHA's published data to ECHA CHEM here.
Diss Factsheets
Use of this information is subject to copyright laws and may require the permission of the owner of the information, as described in the ECHA Legal Notice.
EC number: 273-309-3 | CAS number: 68956-56-9
- Life Cycle description
- Uses advised against
- Endpoint summary
- Appearance / physical state / colour
- Melting point / freezing point
- Boiling point
- Density
- Particle size distribution (Granulometry)
- Vapour pressure
- Partition coefficient
- Water solubility
- Solubility in organic solvents / fat solubility
- Surface tension
- Flash point
- Auto flammability
- Flammability
- Explosiveness
- Oxidising properties
- Oxidation reduction potential
- Stability in organic solvents and identity of relevant degradation products
- Storage stability and reactivity towards container material
- Stability: thermal, sunlight, metals
- pH
- Dissociation constant
- Viscosity
- Additional physico-chemical information
- Additional physico-chemical properties of nanomaterials
- Nanomaterial agglomeration / aggregation
- Nanomaterial crystalline phase
- Nanomaterial crystallite and grain size
- Nanomaterial aspect ratio / shape
- Nanomaterial specific surface area
- Nanomaterial Zeta potential
- Nanomaterial surface chemistry
- Nanomaterial dustiness
- Nanomaterial porosity
- Nanomaterial pour density
- Nanomaterial photocatalytic activity
- Nanomaterial radical formation potential
- Nanomaterial catalytic activity
- Endpoint summary
- Stability
- Biodegradation
- Bioaccumulation
- Transport and distribution
- Environmental data
- Additional information on environmental fate and behaviour
- Ecotoxicological Summary
- Aquatic toxicity
- Endpoint summary
- Short-term toxicity to fish
- Long-term toxicity to fish
- Short-term toxicity to aquatic invertebrates
- Long-term toxicity to aquatic invertebrates
- Toxicity to aquatic algae and cyanobacteria
- Toxicity to aquatic plants other than algae
- Toxicity to microorganisms
- Endocrine disrupter testing in aquatic vertebrates – in vivo
- Toxicity to other aquatic organisms
- Sediment toxicity
- Terrestrial toxicity
- Biological effects monitoring
- Biotransformation and kinetics
- Additional ecotoxological information
- Toxicological Summary
- Toxicokinetics, metabolism and distribution
- Acute Toxicity
- Irritation / corrosion
- Sensitisation
- Repeated dose toxicity
- Genetic toxicity
- Carcinogenicity
- Toxicity to reproduction
- Specific investigations
- Exposure related observations in humans
- Toxic effects on livestock and pets
- Additional toxicological data

Endpoint summary
Administrative data
Link to relevant study record(s)
Description of key information
Key value for chemical safety assessment
- Bioaccumulation potential:
- no bioaccumulation potential
Additional information
1) Information on monocyclic terpenes:
1 -1) Information on terpinolene and dipentene (via d-limonene):
d-limonene and terpinolene are monocyclic monounsaturated terpenes and they have very similar physico-chemical parameters as shown in the table below, therefore information on the structure-related d-limonene is given because it is considered to be representative of the toxicokinetic properties of terpinolene.
|
Molecular weight |
Water solubility (mg/L) |
Log Kow |
Vapour pressure |
d-limonene |
136 |
Column elution method : 3.99 Slow stirring method : 5.69 |
4.43 |
200 Pa at 25°C |
Terpinolene |
136 |
Column elution method : 4.55 Slow-stirring method : 7.03 |
4.36 |
101 Pa at 20°C |
Terpinolene has a relatively low molecular weight of 136. It is a liquid with a low water solubility of about 4.6 mg/L and has high lipophilic properties (log Kow = 4.36). Vapour pressure was determined to be about 101 Pa at 20°C.
Studies of terpene hydrocarbons indicate that they are rapidly absorbed, distributed, metabolized and excreted. The principal metabolic pathway involves side chain oxidation to yield monocyclic terpene alcohols and carboxylic acids. These metabolites are mainly conjugated with glucuronic acid and excreted in the urine, or to a lesser extent in the feces. A secondary pathway involves epoxidation of either the exocyclic or endocyclic double bond yielding an epoxide that is subsequently detoxicated via formation of the corresponding diol or conjugation with glutathione. Humans are continually exposed to limonene and terpinolene throughout their lifetimes, via consumption of a traditional diet or inhalation of air. Extensive studies on d-limonene show rapid metabolism to polar oxidized metabolites, followed by conjugation and rapid excretion.
Absorption:
Terpinolene being lipophilic (log Kow = 4.36), the rate of uptake into the stratum corneum is expected to be high while the rate of penetration is likely to be limited by the rate of transfer between the stratum corneum and the epidermis. Moreover, it is assumed that the dermal uptake is also limited by the low water solubility of terpinolene. These assumptions are supported by the absence of systemic effects following single-dose dermal application of terpinolene up to 2000 mg/kg bw which would suggest a limited systemic absorption through cutaneous barriers. However, terpinolene was found to be skin sensitizing therefore some uptake, even limited, must have occurred. Thus, dermal absorption of terpinolene is expected to be limited but not inexistent.
In shaved mice, the dermal absorption of [3H]d/l-limonene from bathing water was rapid, reaching the maximum level in 10 minutes (von Schäfer & Schäfer, 1982). In one study (one hand exposed to 98% d-limonene for 2 hours), the dermal uptake of d-limonene in humans was reported to be low compared with that by inhalation (Falk et al., 1991); however, quantitative data were not provided.
Terpinolene has high log Kow (>4) and it is a small molecule (molecular weight < 200) therefore, it could be absorbed orally by passive diffusion. It is of adequate molecular size to participate in endogenous absorption mechanisms within the mammalian gastrointestinal tract. Being lipophilic, it may cross gastrointestinal epithelial barriers even if the absorption may be limited by the inability of the substance to dissolve into gastro-intestinal fluids and hence make contact with the mucosal surface. The acute oral gavage toxicity study identified no evidence of systemic toxicity, i.e. neither mortality nor macroscopic effects although high lethal doses were tested (up to 5 mL/kg bw). Oral bioavailability is confirmed in a combined repeated dose toxicity study with reproduction/developmental toxicity screening test where bodyweight gain was reduced at the highest dose group for females. Macroscopic observations at necropsy showed an increase in liver weight both absolute and relative to terminal body weight in males. Histopathology revealed reversible minimal to slight centrilobular hepatocellular hypertrophy in males treated with 2500 and 5000 ppm. These adaptive changes recorded in liver are compatible with metabolism in this detoxifying organ in response to xenobiotic exposure.
Orally administered d-limonene is rapidly and almost completely taken up from the gastrointestinal tract in humans as well as in animals (Igimi et al., 1974; Kodama et al., 1976). Infusion of labelled d-limonene into the common bile duct of volunteers revealed that the chemical was very poorly absorbed from the biliary system (Igimi et al., 1991).
Thus, indications of oral uptake of terpinolene at high doses are given while dermal uptake would be more limited.
No study by inhalation was performed. However, considering the low vapour pressure of terpinolene (<500 Pa), exposure to terpinolene by inhalation is likely to be very limited.
d-limonene has a high partition coefficient between blood and air and is easily taken up in the blood at the alveolus (Falk et al., 1990). The net uptake of d-limonene in volunteers exposed to the chemical at concentrations of 450, 225, and 10 mg/m3 for 2 hours during light physical exercise averaged 65% (Falk Filipsson et al., 1993).
Therefore the potential bioavailability of terpinolene can be considered mainly by oral route.
Distribution:
Terpinolene is a small molecule with low water solubility and high lipophilicity which indicates that terpinolene could be widely distributed; based on its lipophilic character, the substance would readily cross cellular barriers or would be distributed into fatty tissues with a low potential to accumulate.
d-Limonene is rapidly distributed to different tissues in the body. A high oil/blood partition coefficient and a long half-life during the slow elimination phase suggest high affinity to adipose tissues (Falk et al., 1990; Falk Filipsson et al., 1993). In rats, the tissue distribution of radioactivity was initially high in the liver, kidneys, and blood after the oral administration of [14C]d-limonene (Igimi et al., 1974); however, negligible amounts of radioactivity were found after 48 hours. Differences between species regarding the renal disposition and protein binding of d-limonene have been observed. For rats, there is also a sex-related variation (Lehman-McKeeman et al., 1989; Webb et al., 1989). The concentration of d-limonene equivalents was about 3 times higher in male rats than in females, and about 40% was reversibly bound to the male rat specific protein, 2µ-globulin (Lehman-McKeeman et al., 1989; Lehman-McKeeman & Caudill, 1992).
Metabolism:
No data are available but, in in vitro genotoxicity studies, differences in cytotoxicity were observed with and without metabolic activation: in Ames test, terpinolene concentrations leading to cytotoxicity were higher in presence of metabolic activation than without metabolic activation. The same effect was observed in chromosome aberration test on human lymphocytes. This indicates that terpinolene is metabolised by hepatic microsomal fractions.
Also, in a combined repeated dose toxicity study with reproduction/developmental toxicity screening test, adaptive effects such as increased liver weight and minimal to slight diffuse hepatocellular hypertrophy were observed in males at high doses, which is suggestive of metabolism in this organ.
In humans, limonene given orally yields the following major plasma metabolites: perillic acid, limonene-1,2-diol, limonene-8,9-diol, and dihydroperillic acid, probably derived from perillic acid (Poon et al., 1996; Crowell et al., 1994; Vigushin et al., 1998). Peak plasma levels for all metabolites were achieved 4-6 hours after administration, with the exception of limonene-8,9-diol which reached its peak level one hour after administration (Crowell et al., 1994). Phase II glucuronic acid conjugates have been identified in the urine of human volunteers for all metabolites (Poon et al., 1996; Kodama et al., 1974; 1976).
The biotransformation of d-limonene has been studied in many species, with several possible pathways of metabolism (Figure 1). Metabolic differences between species have been observed with respect to the metabolites present in both plasma and urine. About 25–30% of an oral dose of d-limonene in humans was found in urine as d-limonene-8,9-diol and its glucuronide; about 7–11% was eliminated as perillic acid (4-(1- methylethenyl)-1-cyclohexene-1-carboxylic acid) and its metabolites (Smith et al., 1969; Kodama et al., 1976). In another study, perillic acid was reported to be the principal metabolite in plasma in both rats and humans (Crowell et al., 1992). Other reported pathways of limonene metabolism involve ring hydroxylation and oxidation of the methyl group (Kodama et al., 1976). Urinary metabolites isolated from male rabbits orally administered [14C]-d-limonene included perillic acid-8,9-diol (major), p-menth-1,8-dien-10-ol, p-menth-1-ene-8,9-diol, perillic acid, p-mentha-1,8-dien-10-yl glucuronic acid and 8-hydroxy-p-menth-1-en-9-ylbeta-glucopyranosiduronic acid [Kodama et al., 1974].
In Phase I metabolism, the biotransformation of d-limonene and terpinolene are catalyzed by NADPH-dependent cytochrome P450 (CYP). d-Limonene (monocyclic hydrocarbon) has been shown to be substrate (upon repeated administration) and competitive inhibitor of the isoenzyme, specifically CYP2B1 and CYP2C11 (Miyazawa et al., 2002). Limonene has also been shown to induce the members of the CYP2B family in several studies (Maltzman et al., 1991; Hiroi et al., 1995).
Excretion:
Having a molecular weight lower than 300, terpinolene is expected to be mainly excreted in urine and no more than 5-10% may be excreted in bile. Urinary excretion is supported by metabolism data described above but also by the effects identified in the repeated dose toxicity study with reproduction/developmental toxicity screening test. At 3000 and 9000 ppm, partly reversible changes in kidney (tubular degeneration/regeneration, hyaline droplets and granular casts) were observed in main phase and recovery males. Although these effects are specific to male rat, they are suggestive of excretion via urine of the parent molecule or its metabolites.
Clearance from the blood was 1.1 litre/kg body weight per hour in males exposed for 2 hours to d-limonene at 450 mg/m3 (Falk Filipsson et al., 1993). About 60% of the radiolabelled d-limonene administered by inhalation was recovered from the urine, with 5% from feces and 2% from expired CO2in rats (Igimi et al., 1974). Following the inhalation exposure of volunteers to d-limonene at 450 mg/m3 for 2 hours, three phases of elimination were observed in the blood, with half-lives of about 3, 33, and 750 minutes, respectively (Falk Filipsson et al., 1993). About 1% of the amount taken up was eliminated unchanged in exhaled air, whereas about 0.003% was eliminated unchanged in the urine. When male volunteers were administered (per os) 1.6 g [14C]d-limonene, 50–80% of the radioactivity was eliminated in the urine within 2 days with less than 10% appearing in the feces (Kodama et al., 1976). Limonene has been detected, but not quantified, in breast milk of non-occupationally exposed mothers (Pellizzari et al., 1982).
Accumulative potential:
Terpinolene has a low water solubility (< 100 mg/L) and high log Kow (>4) therefore it has affinity to adipose tissues; however, bioaccumulation is not expected to occur, since it is efficiently metabolized to yield oxygenated metabolites that are subsequently conjugated with glucuronic acid and excreted mainly in the urine.
1-2) Information on Terpinolene multiconstituent:
In a Combined Repeated Dose Toxicity Study with the Reproduction / Developmental Toxicity Screening Test conducted according to OECD Guideline 422, main phase males exposed to Terpinolene multiconstituent showed a slight increase in absolute and body weight relative liver weights, compared to controls. Centrilobular hepatocellular hypertrophy was observed in males with an incidence and/or severity proportional to the dose administered at all dietary inclusion levels. Liver of females receiving 7500 ppm also showed minimal centrilobular hypertrophy. After fourteen days of recovery, liver morphology was considered to have returned to normal. The hepatocellular hypertrophy observed was considered as an adaptive metabolic response of the liver to the presence of a xenobiotic. In kidneys, treatment-related lesions characterized by tubular degeneration and regeneration, granular casts, interstitial fibrosis and mixed cell infiltration, mainly of the proximal portion of the nephrons, were observed in males receiving 7500 ppm. The lesions were suggestive of alpha2μ-globulin nephropathy.
These observations are suggestive of metabolism of Terpinolene multiconstituent in the liver and excretion in urine; they confirm the data available on terpinolene and d-limonene for which similar effects were observed on liver and kidneys in repeated dose toxicity studies.
2) Information on bicyclic terpenes (mainly via alpha pinene, beta pinene, delta-3 -carene and turpentine oil):
2-1) Information on alpha pinene and beta pinene:
The toxicokinetics of alpha pinene was studied in humans. Eight healthy males were exposed to 10, 225, or 450 mg/m3 (+)-alpha pinene or 450 mg/m3 (-)-alpha pinene for 2 h in an inhalation chamber while performing light work (50 watts). Average pulmonary uptake of (+)-alpha pinene and (-)-alpha pinene amounted to 59% of the exposure concentration. Absolute uptake increased linearly with concentration. Mean blood concentration at the end of exposure were linearly related to inhaled concentration. The terminal t1/2 of alpha pinene from the blood 695 min for (+)-alpha pinene and 555 min for (-)-alpha pinene. Cumulative urinary excretion of unchanged alpha pinene amounted to less than 0.001% of each dose. Respiratory elimination of (+)-alpha pinene and (-)-alpha pinene was 7.7 and 7.5% of total uptake, respectively (Falk et al., 1990; cited in HSDB 2003 and 2009a).
Similarly, the renal elimination of verbenols after experimental exposure to (+) and (-)alpha pinene was studied in humans following exposure to 10, 225, and 450 mg/m3 terpene in an exposure chamber. The pulmonary uptake was about 60%. About 8% was eliminated unchanged in exhaled air. Depending on the exposure level, about 1%-4% of the total uptake was eliminated as cis- and trans-verbenol. Most of the verbenols were eliminated within 20 h after a 2-h exposure. The renal excretion of unchanged alpha-pinene was less than 0.001%. (Levin et al., 1992, cited in HSDB 2009a)
Both alpha pinene and beta pinene are readily absorbed through the pulmonary system, the skin, and the intestines (Clayton and Clayton,1981-1982; Budavari et al., 1996; cited in HSDB, 2009a and 2009b)
Pulmonary uptake of delta-3-carene increased linearly with exposure, approaching 70% for 225 and 450 mg/m3 exposure levels during 2-h exposures. Delta-3-carene persists in the blood with an extended half-life, suggesting a high affinity for adipose tissues (Falk et al., 1991).
The biotransformation of (+)-, (-)-, and (+/-)-alpha pinenes, (-)-beta pinene and (+)-delta-3-carene in rabbits was investigated. The major metabolites were as follows: (-)-trans-verbenol from (+)-, (-)-, and (+/-)-alpha pinenes; (-)-10-pinanol and (-)-1-p-menthene-7,8-diol from (-)-beta pinene; (-)-m-mentha-4,6-dien-8-ol, 3-caren-9-ol, (-)-3-carene-9-carboxylic acid, and 3-carene-9,10-dicarboxylic acid from (+)delta-3-carene. (Ishida, 1981)
Dermal absorption: Results demonstrate rapid penetration of pinenes not only to the first stratum corneum layers but also to viable epidermis and dermis. In an vitro study, alpha pinene and beta pinene did not permeate across the skin to the acceptor medium due to large cumulation in the skin tissue (Cal et al., 2006). However, following immersion of young pigs and one human subject for 30 minutes in baths containing 150 mL of a pine-oil mixture, alpha and beta pinene were detected in exhaled air within 20 minutes reaching maximum levels 50-75 minutes after start of the bath and remained detectable after 1 day. (Opdyke, 1979, cited in HSDB 2009)
2 -2) Information on turpentine oil:
Eight male volunteers were exposed to 450 mg/m3 turpentine oil by inhalation (2 h, 50 W) in an exposure chamber. The mean relative uptakes of alpha pinene, beta pinene, and delta-3-carene were 62%, 66%, and 68% respectively, of the amount inhaled. Between 2% and 5% of the net uptake was excreted unchanged in the expired air after the end of exposure. The mean blood clearance 21 h after exposure (CL21h) of alpha pinene, beta pinene and delta-3-carene, were 0.8, 0.5, and 0.4 L/kg/h, respectively. The mean half lives (t1/2) of the last phase of alpha pinene, beta pinene, and delta-3-carene averaged 32, 25, and 42 h, respectively. (Filipsson et al., 1996)
Since turpentine oil is a lipophilic substance, it can potentially accumulate in fatty tissues. In rats, the highest concentrations of inhaled turpentine oil were found in the spleen, kidneys, brain, and perinephric fat (Sperling et al., 1967; Savolainen et al., 1978).
Elimination of turpentine oil and its metabolites is primarily through the urinary tract (Lewander and Aleguas, 1998, cited in HSDB 2003). A portion of the turpentine oil absorbed in industrial exposures is exhaled unchanged in expired air. The remainder is excreted in the urine as glucuronic acid conjugates (Bingham, 2001, cited in HSDB 2003).
The blood and urine monoterpene concentrations were continuously monitored from a patient attempting suicide by ingestion of 400-500 mL pine oil. The blood and urine monoterpene concentrations were continuously monitored. The data suggest that monoterpenes are poorly resorbed in the gastrointestinal tract. The resorbed portion of the hydrocarbons cumulates in the lipophilic body compartments and is slowly metabolized and then excreted by the kidneys. The main metabolic pathways are hydratation, hydroxylation, rearrangement, and acetylation (Koppel, 1981).
In conclusion, bioaccumulation of these terpene hydrocarbons does not occur, since the substances are efficiently metabolised to yield oxygenated metabolites that are subsequently conjugated with glucuronic acid and excreted mainly in the urine.
References:
Belsito, D., Bickers, D., Bruze, M., Calow, P., Greim, H., Hanifin, J.M., Rogers, A.E., Saurat, J.H., Sipes, I.G., Tagami, H., 2008. A toxicologic and dermatologic assessment of cyclic acetates when used as fragrance ingredients. Food Chem. Toxicol. 46 Suppl 12, S1–27.
Ishida, T., Toyota, M., Asakawa, Y., 1989. Terpenoid biotransformation in mammals. V. Metabolism of (+)-citronellal, (+-)-7-hydroxycitronellal, citral, (-)-perillaldehyde, (-)-myrtenal, cuminaldehyde, thujone, and (+-)-carvone in rabbits. Xenobiotica 19, 843–855.
Alicyclic Primary Alcohols, Aldehydes, Acids, and Related Esters,WHO Food Additives Series 50
Falk Filipsson, A., 1998. Concise International Chemical Assessment Document 5 - Limonene, 32 Chapitre 7. Comparative kinetics and metabolism in laboratory animals and humans.
Flavor and Fragrance High Production Volume Chemical Consortia, 2006, HPV Monoterpene Hydrocarbons
Madyastha KM, Chadha A. 1986 Metabolism of 1,8-cineole in rat: its effects on liver and lung microsomal cytochrome P-450 systems. Bull Environ Contam Toxicol. Nov;37(5):759-66.
Miyazawa M, Shindo M. 2001 Biotransformation of 1,8-cineole by human liver microsomes. Nat Prod Lett.;15(1):49-53.
HSDB (Hazardous Substances Data Bank). 2003. Turpentine. HSDB No. 204. Produced by the National Library of Medicine (NLM), Bethesda, M.D. Last updated 15 October 2003.
HSDB (Hazardous Substances Data Bank). 2009a. Alpha pinene. HSDB No. 720. Produced by the National Library of Medicine (NLM), Bethesda, M.D. Last Revision Date: 26 June 2009
HSDB (Hazardous Substances Data Bank). 2009b. beta pinene. HSDB No. 5615. Produced by the National Library of Medicine (NLM), Bethesda, M.D. Last Revision Date: 26 June 2009
Information on Registered Substances comes from registration dossiers which have been assigned a registration number. The assignment of a registration number does however not guarantee that the information in the dossier is correct or that the dossier is compliant with Regulation (EC) No 1907/2006 (the REACH Regulation). This information has not been reviewed or verified by the Agency or any other authority. The content is subject to change without prior notice.
Reproduction or further distribution of this information may be subject to copyright protection. Use of the information without obtaining the permission from the owner(s) of the respective information might violate the rights of the owner.
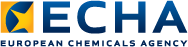